1. Introduction
Emerging contaminants include pharmaceutical products, pesticides, personal care products, surfactants, industrial additives and a wide variety of chemical products, which affect the quality of water, and thus the quality of life of aquatic organisms and humans 1. Specifically, the presence of pharmaceutical compounds in water sources, especially non-steroidal anti-inflammatory drugs, is a major concern because they can affect human health, and also produces negative effects on terrestrial and aquatic ecosystems, due to bio-accumulation 2. The emissions of pharmaceutical compounds (PhCs) to the ecosystem are increasing as result of the hospital discharge, self-medication, elimination of expired drugs, and other sources of emission 3. Therefore, the induced pollution by these residues is classified as being one of the major problems requiring a deep study in order find solutions for their elimination 4. Thus, these residues are still residues in the environment are considered treated as “compounds of emerging concern” and their hazardous potential was recently stablished, determining that some environmental effects of pharmaceuticals can be established in the μg/L and ng/L concentration ranges 5.
There have been advances who have allowed the identification of many pharmaceutical metabolites in the environment; thus could help to unravel their mobility, fate, possible effects and toxicity as well as methods for their efficient removal from different water sources 6. Pharmaceuticals compounds have ecological and environmental risks such as inhibition of protein synthesis, nucleic acid (DNA/RNA) synthesis, exert toxic effects on numerous organisms in the environment, abnormal protein and enzyme activities, antibiotic resistance in bacteria, gene expression alterations, and growth malformations in rats, birds 7. Likewise, various processes are designed to remove toxic pollutants from wastewater prior to entering rivers, including adsorption 4,8, membrane filtration 9, precipitation, and electrochemical advanced oxidation methods 10, in order to preserving the freshwater sources. Finding that, these technologies have good approach at low initial concentrations of pollutants (0.1 to 6 ppm), reaching efficiencies around 55% 11-13.
The adsorption process is one of the most used techniques for water treatment 14; which consist in the retention of the pollutant on the surface of a solid by the action of a physical or chemical bond 15. In this sense, agricultural wastes are substances discarded in the process of agricultural production, and refers to crop stalks and animal manure 16. Several works have used activated carbon as a low-cost adsorbent in the removal of (PhCs) through adsorption process, due of its great pore diffusion and adsorption kinetics, and developed microporous structure, high specific surface area, and can be easy handled 17. Different by-products such as date, peach and olive stones 18-20, cocoa pod husk 3, Mung bean husk 21,22, pine residues 23,24, coffee beans 25, and so on, had been valorized and used in the elimination of ibuprofen from aqueous solutions.
The final disposal of agro-industrial waste, represents an environmental problem such as the appearance of unpleasant odors and the deterioration of the landscape; the cocoa industry increased its production in last years, and the husk is taken as a waste while the fruit is harvested, becoming in a problem of final disposal 26. Cocoa husk has been used for the preparation of activated carbon to remove methylene blue 27, dyes and drugs such as diclofenac and nimesulide 28.
Thus, this study aims to transform cocoa husk into activated carbon with chemical activation with ZnCl2, and to evaluate their characteristics as an Ibuprofen adsorbent compare the behavior of the adsorbents, regarding to the adsorption capacity as well as the kinetic performance, through the development of equilibrium and dynamic adsorption tests. The bio-adsorbent was characterized using a BET analysis. The capacity of ibuprofen removal was determined by UV-Vis.
2. Methodology
2.1. Synthesis of activated charcoal
For the preparation of the raw material, the cocoa shells were initially reduced in size and washed with deionized water, then taken to the oven at a temperature of 105°C for 48 hours. After this time, they could stand until they reached room temperature and were subjected to grinding and sieving to take the particles of size between 1 and 2 mm.
The activated carbon was obtained taking 5g of the prepared biomass, impregnated with a solution of 15 mL of ZnCl2 solution at ratios biomass: solution of 1:2 and 1:3 29,30, and the mixture was brought to the shaker at 50°C for 3 hours, to ensure the access of ZnCl2 to the interior of the biomass. Then, it was heated to a temperature range from 150 to 350°C in one muffle with a ramp of 5°C/min in Nitrogen atmosphere (110mL/min) 31, leaving the final temperature for 3 hours.
Once the heating was finished, the system was cooled at room temperature, and the product obtained, was washed with a 0.1 M hydrochloric acid solution in order to remove the ZnCl2 residues. For a better removal, the samples were placed in a shaking incubator (Germmy shaker co) at 120 rpm, at 30°C for 2 hours.
2.2. Characterization of biomass and carbons
Physicochemical characteristics of the bio-adsorbents used were studied using the following techniques: Brunauer-Emmett-Teller analysis (BET), Scanning Electron Microscope (SEM), Energy Dispersive X-ray Spectrometry (EDS) and elemental analysis. These techniques were important to investigate the structure of the materials, bromatological composition and possible interactions with the studied pollutant. Analysis SEM was made by preparing the samples, coating the surface with a gold plating coating in a Denton Vacum Model Desk IV Equipment, this in order to generate a surface that was conductive.
Subsequently, the JEOL Model JSM 6490 LV microscope was inspected in secondary electrons mode (magnifications of 50, 250, 500 and 1000 magnifications were used with 20kV). Analysis EDS: the chemical composition of the samples was evaluated on several points or areas of inspection, by means of the EDS probe of Oxford Instrument Model INCAPentaFETx3. Analysis BET: the measurement of the surface area of charcoals was obtained by the N2 adsorption isotherm at 77 K using the BET equation 32, the BET analysis was performed by using the Micro Active Tri Star II Plus 2.03 equipment.
2.3. Adsorption tests
To evaluate the adsorbent capacity of the coals, 100 mL of an ibuprofen solution with an initial concentration of 20 ppm was prepared and contacted with 0.4 g of bio-adsorbent at 120 rpm during 24 h; the adsorbent was filtered, and the sample was taken to be analyzed by visible ultraviolet spectrophotometry analysis (UV-VIS). The same procedure was also performed for an initial concentration of 30 ppm and 40 ppm 33,34. Adsorption efficiency (%E) and capacity (qt) were determined according to Equations 1 and 2:
Where Ci and Cf are the initial and final concentration in ppm, V is the volume of solution in L and m the mass of adsorbent in g.
3. Results
3.1. Characterization of biomass and activated carbons
Figure 1 shows the images reported by the SEM analysis for unmodified cocoa husk. In this, a fibrous appearance and little porosity was evidenced in comparison with the morphology of the coals presented in Figures 2 and 3.
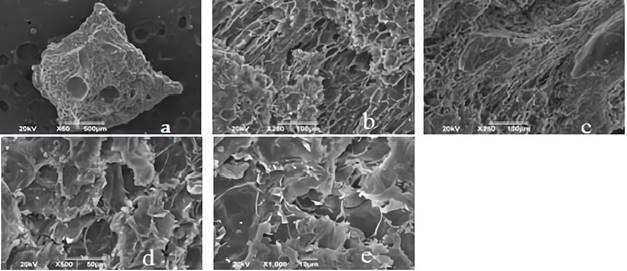
Source: own elaboration
Figure 1 I mages of the biomass (cocoa husk) shown by the SEM analysis with an approach of: a. * 50, b. * 250, c. * 250, d. * 500, e. 1000.
Figures 2 and 3 shows the SEM images for synthetized biochars; in there, the fibrous appearance of the UHC (Figure 1) disappears, suggesting a disintegration of the original structure of the biomaterial; due to the modification with ClZn2 and carbonization process. The roughness of the coals, greater porosity and appearance of cavities is also evident, indicating that these characteristics were developed by the activating agent 28.
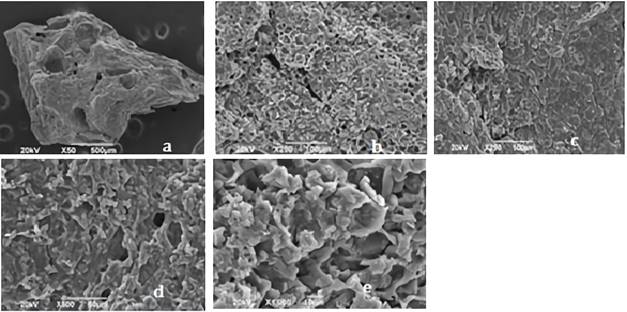
Source: own elaboration
Figure 2 Images of CA1: 2 shown by the SEM analysis with an approach of a. * 50, b. * 250, c. * 25,. d. * 500, e. 1000. CA1: 2= activated carbon with relation 1:2 biomass:ZnCl2 solution.
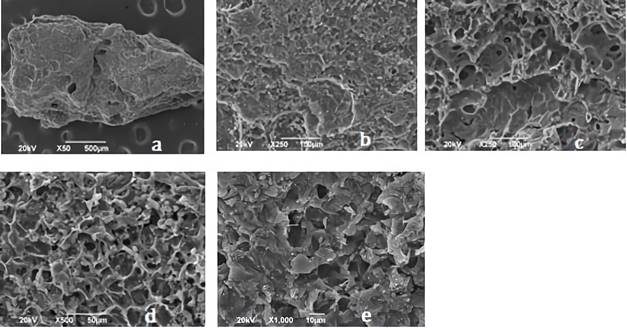
Figure 3 Images of CA1: 3 shown by the SEM analysis with an approach of a. * 50, b. * 250, c. * 250, d. * 500, e. 1000. CA1: 3= activated carbon with relation 1:2 biomass:ZnCl2 solution.
Different types of pores in size and shape are clearly visible, causing the increase in the BET surface area and the micropore volume of the coals. CA1:2 evidenced more pores, while CA1:3 exhibited a more defined pore morphology, this could be attributed to the characteristic impregnation ratio of each carbon; ZnCl2 dehydrates the sample and increases its porosity, as having a 1:2 ratio greater amount of this activating agent results in a material with more pores 35. In Table 1 is shown the elemental analysis of the bio-adsorbents.
Table 1 Elemental Analysis of the biomass
Bio-adsorbent | Elemental Analysis (% p/p) | ||||
---|---|---|---|---|---|
C | O | K | Cl | Zn | |
Cacao husk | 45.01 | 43.78 | 11.21 | -- | -- |
CA 1:2 | 61.73 | 21.24 | -- | 1.01 | 16.77 |
CA 1:3 | 63.04 | 22.72 | -- | 1.46 | 12.78 |
CA1: 2= activated carbon with relation 1:2 biomass:ZnCl2 solution; CA1: 3= activated carbon with relation 1:2 biomass:ZnCl2 solution. Source: own elaboration
According to the elemental analyses (Table 1), the carbons obtained CA1:2 and CA1: 3 exhibited higher carbon content (68.41% - 64.64%) than cocoa husk (51.43%), while the oxygen content decreased after chemical activation, this may be due to the elimination of some groups that contained oxygen from the surface of the material; during the chemical modification the ZnCL2 causes dehydration reactions in the UHC (unmodified cocoa husk) structure occur, and catalyzes dehydration during carbonization; for this reason, the hydrogen and oxygen atoms were released in the form of water to form a porous structure that affect the amount of oxygen groups in the carbonaceous matrix, causing a decrease in oxygen, as observed in the elemental analysis of the carbons obtained and coincides with that reported by Wang et al. 36, it indicates that more O-containing functional groups were lost in biochar. In other studies, similar amounts of carbon and oxygen have been found in prepared carbons, presenting the same tendency after activation (increase in carbon and decrease in oxygen) 37,38. Also, loss of moisture or volatile components, due of these reactions of dehydration 35,38. The biomass presented potassium content, which disappeared once the modification has been made, which is related with the presence of chlorine and zinc in the biochar, this could be due to the replacement and exchange of K ions for Cl and Zn for electrostatic forces as reported by Liu, Ji and Wang 39. As observed in the elemental analysis the biomaterial changed remarkably after the activation process, and the biochars presented similar elementary compositions. After applying the BET method, the information presented in Table 2 was obtained.
Table 2 Characteristics of CH, CA 1:2. CA 1:3. shown by the BET analysis
Sample | SBET (m2/g) | SLangmiur (m2/g) | VTotal (cm³/g) | DBET (nm) | %E |
---|---|---|---|---|---|
UCH | 0.0208 | 0.0675 | 0.000159 | 1.0940 | ---- |
CA1:2 | 297.2066 | 460.4968 | 0.123115 | 1.0566 | 75.96 |
CA1:3 | 287.5375 | 441.4094 | 0.117930 | 1.0634 | 65.75 |
CH= unmodified cocoa husk; CA1: 2= activated carbon with relation 1:2 biomass: ZnCl2 solution; CA1: 3= activated carbon with relation 1:2 biomass: ZnCl2 solution. Source: own elaboration
In Table 2 is observed that an increasing in surface area is proportionally related with the adsorption efficiency of remotion. The UCH textural properties in regard to activated carbons, showed that chemical impregnation produces materials with better characteristics for the adsorption of pharmaceutical compounds 28. It was also evidenced the increased in the surface area with after modification with ZnCl2. About this, CA 1:2 has a greater surface area (297.2066m2/g) than CA 1:3, which is attributed to the impregnation ratio 1: 2 allows better interaction between the chemical agent and the surface of the carbonized solid material, favoring the detachment of the carbonaceous particles that are part of the cellulosic material. With this, the alternative hypothesis is verified in which it is affirmed that the carbon surface area depends on the zinc chloride ratio used.
On the other hand, interactions between the hydroxyl groups of the cellulosic material with the Cl and Zn dissolved in the solution, generated complex reactions producing changes in the carbonaceous matrix, which is evidenced by the formation of the pores 40. It is important to highlight that, activated carbons prepared by chemical activation from lignocellulosic materials, results in microporous materials which is related with volume of micro-pores (Table 2) 31. In addition, when the diameter is less than 2 nm, it is indicated the presence of micropores 41. The area presented by the Langmuir isotherm showed that the adsorption in the monolayer on the surface of the adsorbent at low pressures, directly influences the surface area, which is greater than the BET area; it can be assumed that the process is controlled by physisorption, due to the formation of multilayers on the external surface of the adsorbent 42.
Figure 4 shows a partial isotherm of adsorption of CO2, used to define the porosity for the unmodified cocoa husk (UCH). Initially the unmodified biomass was subjected to N2 adsorption without obtaining adsorption data, since the N2 did not manage to enter due to the lack of porosity of the material.
From Figure 4 is evidenced that the relative pressure increases, this means there is no relationship that shows a considerable adsorption value between the gas adsorbed in the pores of the material and the relative pressure, due there are no inflection points that show this behavior 43. The almost zero adsorption of the material, is evident when noticing the values of the axis of the ordinates. Figure 5 shows the Nitrogen adsorption and desorption of the synthesized carbon-type bio-adsorbents.
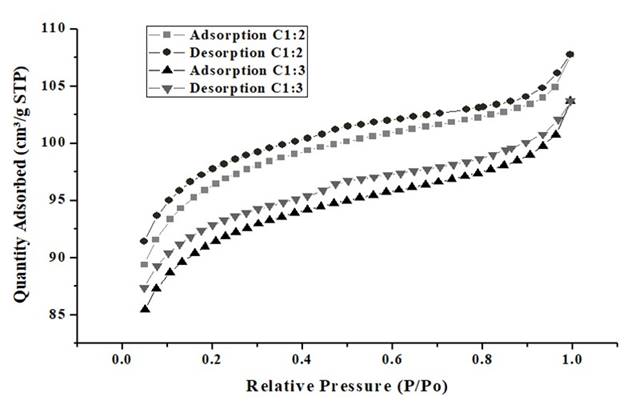
Source: own elaboration
Figure 5 BET analysis of CA 1:2 and CA 1:3; nitrogen adsorption and desorption. CA1: 2= activated carbon with relation 1:2 biomass:ZnCl2 solution; CA1: 3= activated carbon with relation 1:2 biomass:ZnCl2 solution.
Considering Figure 5, it can be seen that the isotherm is Type I, and is according to the IUPAC classification, therefore CA 1:2 and CA 1:3 are mostly microporous, which is consistent with the results of the BET analysis, in which the most of the area is represented by micropores, which allows initially, N2 adsorption to increase rapidly to 𝑝/𝑝0 < 0.2 due to the filling of micropores present in the monolayer, between 𝑝/𝑝0 < 0.2 y 𝑝/𝑝0 < 0.9 some of the available pores continue to be covered and at the same time multilayers begin to be developed 44,45.
Between 𝑝/𝑝0 < 0.9 y 𝑝/𝑝0 < 0.1 there is a greater inclination, because there is no adsorption on the pores of the material, producing a saturation or condensation of N2 molecules (desorption). It is important to note that for chemically activated lignocellulosic materials, the BET equation gives an adequate description of a relative pressure range, normally between 0.05 and 0.3, in which, for this figure, the behavior of Type I isotherm is more than evident 46. In addition, can see that in the initial stage, the adsorbed amount of N2 increases rapidly at low relative pressures, which is due to the microporous structure of the carbon as reported for Arampatzidou and Deliyanni 47, and thus, the curve has a behavior analogous to the characteristic curve of CA 1:2.
3.2. Adsorption essays
Figures 6 and 7show a high decrease in the concentration of IBP (Ibuprofen) during the initial stage, for both carbons.
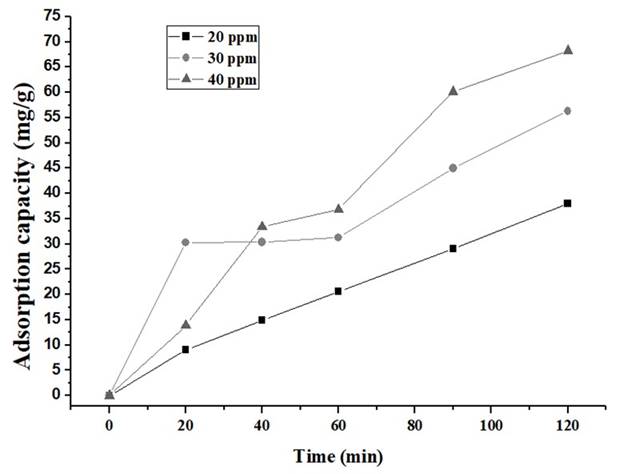
Source: own elaboration
Figure 6 Removal of IBP with CA1:2 in the solution of 20, 30 and 40 ppm. Source: own elaboration
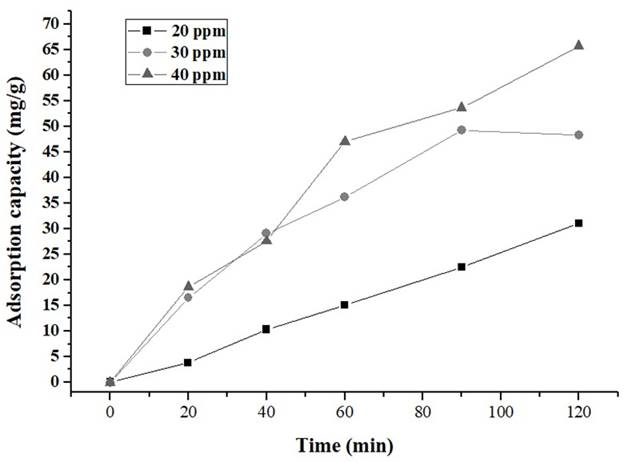
Source: own elaboration
Figure 7 Removal of IBP with CA1:3 in the solution of 20, 30 and 40 ppm CA1: 2= activated carbon with relation 1:2 biomass:ZnCl2 solution; CA1: 3= activated carbon with relation 1:2 biomass:ZnCl2 solution.
The adsorption experiments were carried out at 20, 30 and 40 ppm as initial concentration and results are shown in Table 3. It was evidenced that CA 1:2 has higher IBP removal percentages than the CA1:3. This, could be attributed to the difference in surface areas and volume of pores (Table 2). Nevertheless, in both biochars were observed removal percentages above 60%, due to the presence of micropores which facilitates the removal of ibuprofen 48.
Table 3 Removal percentage obtained with CA1: 2 and CA1: 3
Type of Carbon | Initial concentration (ppm) | Final concentration (ppm) | % E | qt (mg/g) |
---|---|---|---|---|
CA1:2 | 20 | 4.809 | 75.96 | 37.98 |
30 | 7.486 | 75.05 | 56.28 | |
40 | 12.69 | 68.28 | 68.27 | |
CA1:3 | 20 | 7.587 | 62.07 | 31.03 |
30 | 10.52 | 64.93 | 48.7 | |
40 | 13.7 | 65.75 | 65.75 |
CA1: 2= activated carbon with relation 1:2 biomass:ZnCl2 solution; CA1: 3= activated carbon with relation 1:2 biomass:ZnCl2 solution; %E= Adsorption efficiency. Source: own elaboration
Numerous applications have been reported in the use of activated carbons from biomass. In Table 4, some representative studies of this type are presented, disclosing the precursor material and the activation process used, BET surface area and specific application. As can be seen, the objective is to remove emerging contaminants, and the activating agent that leads to greater surface areas is ZnCl2.
Table 4 Comparison of activated carbons from agricultural waste with different types of activation
Biomass | Treatment | SBET (m2/g) | Pollutant | qt (mg/g) | References |
---|---|---|---|---|---|
Cocoa Husks | Physical, CO2 | 85.09 | Blue methylene dye | 212.77 | 49 |
Cocoa Husks | Physical, CO2 | 248.75 | 4-nitrophenol | 166.67 | 50 |
Cocoa Husks | Physical, CO2 | 558.25 | ---- | ---- | 51 |
Seeds of Elaeagmus angustifolia | Chemical, ZnCl2 | 697 | Iodine | 1009 | 41 |
Cocoa Husks | Chemical, assisted by microwaves | 619 | Diclofenac | 63.47 | 28 |
Nimesulide | 74.81 | ||||
Pineapple | Chemical, ZnCl2 | 914.67 | Blue methylene dye | 288.34 | 35 |
Artemisia vulgaris | Physicochemical, H2SO4 y N2 | 358.2 | Ibuprofen | 16.945 | 52 |
Cocoa Husks | Chemical, ZnCl2 | 205.4 | Amoxicillin | 3.096 | 53 |
Ibuprofen | 5.462 | ||||
Cocoa Husks | Chemical, ZnCl2 | CA1: 2= 297.21 | Ibuprofen | 68.27 | Present study |
CA1: 3= 287.54 | 65.75 |
Source: own elaboration
The carbons obtained in the mentioned investigations presented N2 Type I adsorption-desorption isotherms, reflecting microporosity as the main characteristic, which is also seen in the coals obtained in this project as shown in Table 4 below. It is observed that, although the carbon obtained in the present study has a lower surface area, compared to some previously obtained at high temperatures, like the one prepared by cocoa shell microwave-assisted (619 m2/g) and Artemisia vulgaris modified with H2SO4 and N2 (358.2 m2/g), obtained an adsorption capacity over those; which shows that activated carbon from cocoa treated with ZnCl2 and synthesized at low temperature, is a good option for its implementation in the removal of contaminants dissolved in aqueous solution and pharmaceutical metabolites.
4. Conclusions
The activation of the cocoa husk caused an increase in its surface area and porosity, which was evidenced in the results of the SEM and BET analyzes, carried out to characterize the biomass and the activated carbons, being the CA1: 2 the one that presented such properties in greater proportion, whose percentage of removal of ibuprofen was 75.96% while for CA1: 3 was 65.75%, CA1: 2 being the most suitable for adsorption.
The coals in general, turned out to be good materials for the removal of the drug, with a maximum adsorption capacity of 68.27 mg/g and 65.75 mg/g respectively. The potential of the cocoa husk as a precursor for the generation of activated carbons was demonstrated, which represents a valuable contribution to the research lines related to the use of agro-industrial waste. The use of the low temperature method has the potential to improve the production of activated carbons in economic aspects, which differs from the conventional known method (high temperature).