1. INTRODUCTION
Different industrial sectors in the world generate impacts on the environment due to the use of coal as an energy source. Coal combustion has increased the presence of thick, fine, and ultrafine particles suspended in the air, with a relationship existing between morbidity and mortality of people due to exposure to contamination from these materials 1,2. In developing countries, the brick industry is one of the main sources of pollution due to the use of coal in its production processes 3. The health risks to people exposed to this type of activity have been demonstrated 2,4,5,6. Another important aspect is that ash waste is destined for outdoor landfills, becoming a danger to public health and the environment 7. Ash waste is not included in the list of hazardous waste in many regions, but its final disposal must be carried out with care due to the content of heavy metals 8. In this way, knowing about the effects produced by coal combustion waste is important for its management and regulation; in this way, people's health can be safeguarded, as well as protecting the environment.
On the other hand, brick manufacturing can be carried out in intermittent and continuous kilns 9. The fuel used in this type of oven is coal due to its high energy generation potential that is released through combustion 5. For the year 2021, British Petroleum (BP) published global energy statistics, where coal represents more than 35.1% of global energy 10. It is estimated that globally, coal combustion generates around 1000 tons of ash per year, of which less than 50% is recycled 11. It is worth mentioning that coal is a product of interactions of organic and inorganic materials in the upper layers of the earth until it becomes a deposit of peat related to the presence of components toxic to human health 12. The three-dimensional structure of carbon is made up of hydrocarbons and aromatic compounds connected by alkyl bridges, ether, and thioether bonds; these compounds in turn, through radical cyclization or condensation reactions, form polycyclic aromatic hydrocarbons (PAHs) 13. Where these are absorbed in ashes, which when emitted in gases or particles can cause pollution in the environment 14.
Among the residues from coal combustion, there is particulate matter (PM), with different physical, chemical, morphological, and biological characteristics 2. PM is classified into sub-micrometric particles with an aerodynamic diameter of less than 1 micron and micrometric particles with an aerodynamic diameter greater than 1 micron and less than 10 microns 15. In this sense, the most common waste is bottom ash (CFC) and fly ash (CVC) rich in minerals, heavy metals such as As, Pb, Hg, Cd, Cr, Sb, and radioactive elements that are preserved in the combustion of carbon as they are indestructible elements in the process 5,7. The composition of ash is classified into two categories: organic and inorganic, the first classification is composed of unburned carbon, which is usually used as an indicator of incomplete combustion 16. The composition of CFCs is mostly silicates, aluminates, carbonates, metalloids, and heavy metals 17. Gallardo et al, 18 measured the surface area of CFC and CVC which is between 1.16 and 2.92 m2 /g respectively; highlighting that small particles have a larger surface area; therefore CVCs tend to be more harmful to the environment and human health.
The coal combustion process also releases polluting gases, the main ones being COx, SOx, and NOx 1,5. Gas emissions generate effects on acid rain, the ozone layer, and the food network due to damage to plants and animals 3. CO and CO2 emissions present the most adverse effects on the environment due to their contribution to global warming 5. When inhaled, SOx destabilizes the heart rate, causing heart attacks, asphyxiation, cough and decreased lung activity, lung and skin cancer. Likewise, NOx is very corrosive and oxidizing. Contact with this gas causes a decrease in lung function, asthma, respiratory failure, and structural DNA changes 7. CO2 is the most harmful emission from brick kilns, followed by PM, COx, SOx, NOx, fluoride compounds, and carcinogenic dioxins 3.
This study aims at carrying out a characterization of CFCs generated in a Colombian brick industry, focusing on the evaluation of particle size through microscopy techniques; complemented with a brief ecotoxicity study. This is to analyze the possible impacts on human health and its ecotoxic effects. In this sense, optical microscopy, transmission electron microscopy (TEM), scanning electron microscopy (SEM), and thermogravimetric analysis (TGA) techniques were used for physical characterization. A chemical, mineralogical, and loss due to ignition analysis was also carried out. Finally, phytotoxicity was evaluated with a germination test.
2. METHODOLOGY
To study the characteristics of bottom ash, samples were taken from a continuous tunnel kiln in a Colombian brick industry. In this case, the ashes come from the burning of bituminous coal, as a source of energy, for firing clay bricks at a temperature of 850 °C. Three ash samples CFC1, CFC 2, and CFC3 were collected in different weeks of production, at two-week intervals.
a. Characterization of bottom ash
For particle size analysis, the optical microscopy technique was used with the Zeiss Axio Lab. A1 equipment; Transmission electron microscopy (TEM) with the Jeol Jem-1011 equipment and TESCAN VEGA fourth generation Scanning Electron Microscopy (SEM) with a tungsten filament electron source. The morphology of the ashes was also observed with a Jeol 5000 scanning electron microscope (SEM).
The preparation of samples in optical microscopy was as follows: three ash samples (CFC1, CFC2, and CFC3) were placed individually on the slides and covered with coverslips. The samples were observed with the 5x, 10x, 20x, and 40x objectives, and ten photographs were taken with the ZEISS 3.1 software for each objective with the scales of 200 µm, 100 µm, 50 µm, and 20 µm respectively. The analysis of the photographs was carried out with the ImageJ 1.53 k software in which the diameter was measured. This program provided the data table to make the histogram of the number of particles with their corresponding diameters.
For particle size analysis in TEM, ethanol suspensions of the three ashes studied were used. The function of the suspension is to break up the aggregates to form individual particles for analysis under the microscope since most minerals are insoluble in alcohol 19,20. The suspensions were stirred for one minute, and then with the pipette they were placed on copper grids and allowed to evaporate. With the Digital Micrograph 3.5-Gatan-Inc software, eight photographs were taken in TIFF format with dimensions of 3072x3072 pixels and 72 dpi at 32 bits.
Regarding the preparation of samples in SEM, it was necessary to dry the samples in a Pro-Jet Finisher oven at 50 °C for 24 hours. Subsequently, with the Cressington 108 Auto metalizing equipment, the samples were covered with a thin layer of gold to generate electric conductivity, with an exposure of 60 s. The samples were observed on the TESCAN VEGA microscope at the magnifications, 200x, 500x, and 1,000x and on the Jeol 5000 microscope at the magnifications 27x, 1000x.
Chemical analysis was determined by X-ray fluorescence with a MagisPro PW-2440 Philips X-ray fluorescence spectrometer (WDXRF) incorporated with a Rhodium tube, with a maximum power of 4 KW.
b. Ecotoxicity test
Toxicity was assessed for sample CFC2 with a Vigna radiata (mung bean or green soybean) germination test. This sample was selected according to the chemical composition, presented later, which shows a higher content of heavy metals. The test used was direct contact with the methodology proposed by 21. The tests were carried out in triplicate Petri dishes with the following concentrations of solid ash: 6.25%, 12.5%, 25%, 50%, and 100% (% w/w) which were mixed with commercial soil (Figure 1). The Petri dishes were filled with up to 4 g of mixture. 1200 µL of distilled water was also used for the soil mixtures and samples at 100% ash concentration. Ten vigna radiata seeds were placed on the surface of each sample. The Petri dishes were covered and allowed to germinate in the dark for 72 hours, with a temperature of 25°C and an average humidity of 68%. Measurement of pH was also carried out with the Thermo Scientific Orion Star A211 equipment and conductivity with the Sper Scientific Benchtop Meter equipment. The toxicity analysis parameters included the percentage of seed germination, root elongation, and germination index, used by the author Jain 22. The calculations were carried out according to equations 1, 2, and 3 respectively. The significant effects in the different ash concentrations were evaluated by analysis of variance (ANOVA) followed by Tukey's HSD (Honestly Significant Difference) comparison; in addition, InfoStat statistical software was used for data analysis.
3. RESULTS AND DISCUSSION
a. Particle size
Interquartile analysis of samples CFC1, CFC2, and CFC3 for optical microscopy showed that 25% of the particles have diameters less than 61 µm, 16 µm, and 149 µm respectively. 75% of the particles have diameters less than 254 µm for CFC1 and CFC2 and less than 292 µm for the CFC3 sample. 50% of the particles have diameters less than 158 µm, 119 µm, and 212 µm for CFC1, CFC2, and CFC3 respectively. The particles with the most frequent diameters are in the range of 4 to 69 µm for CFC1 (see Figure 2a), between 5 to 87 µm for CFC2 (see Figure 2b), for the CFC3 sample between 186 to 273 µm (see Figure 2c). In this way, PM10 content is evident in the CFC1 and CFC2 samples. However, in the CFC3 sample, higher sizes are reported, which may be due to variability in the batch of coal used (bituminous coal) and possibly to an incomplete burning, as reported later with the loss to fire. Some authors have reported that the presence of PM10 in coal combustion is associated with fertility problems in both the female and male reproductive systems and problems in pregnancy 2.
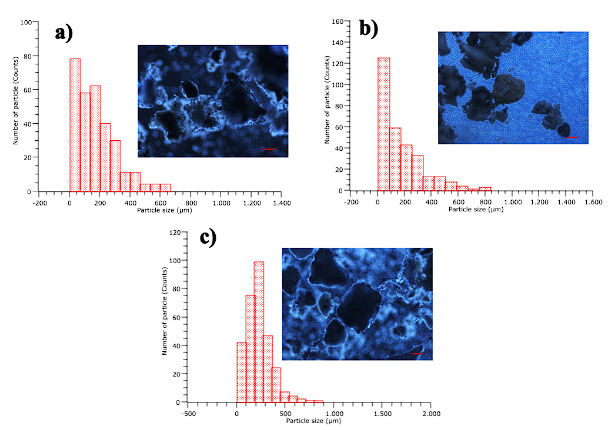
Source: authors
Figure 2 (a) particle size in optical microscopy; CFC1 x10 scale 100 µm, (b) CFC2 x10 scale 100 µm, (c) CFC3 x10 scale 100 µm.
The particle sizes analyzed by TEM indicated that 25% of the particles have diameters less than 17 nm, 75% less than 635 nm, and 50% less than 234 nm, the most frequent diameters are between 5 and 423 nm (see Figure 3). This technique indicated PM10 and PM2.5 particulate matter content. Furthermore, the PM content of less than 2.5 µm is evident, with 50% of the data being less than 234 nm (2.34 µm). These nanomaterials can be reactive because they accumulate a large amount of adsorbates per unit surface; inhaling air with this type of particle composed of transition metals can generate greater health risks 19. Any mineral material with a unit size <100 nm is more active in the environment due to bio adsorption; in the case of carbon particles, this characteristic allows them to encapsulate several dangerous elements 1. The lower the particle size and the higher the concentration of heavy metals, the toxicity increases 18.
On the other hand, coal cleaning waste is especially composed of Al, Si, K, O, and toxic elements such as As, Cd, Cr, Fe, Hg, and Pb with sizes from 5 to 100 nm, which the smaller they are can easily infiltrate the respiratory system, the nervous system and the blood, generating cellular damage, genetic mutations, among others, both in humans and animals 23.
The inter-quartile analysis for SEM showed 25% of particles with diameters less than 15 µm, 3 µm, and 12 µm respectively, 75% of the particles with 38 µm, 7 µm, 29 µm and 50% with 26 µm, 5 µm, 20 µm, for CFC1, CFC2, and CFC3 respectively. The most frequent particle sizes in SEM are in the range of 4 to 12 µm for CFC1 (Figure 4a), for CFC2 between 2 to 11 µm (Figure 4b), and for CFC3 between 12 to 20 µm (Figure 4c). The content of PM10 in CFC1 and CFC2 as well as PM2.5 in CFC2 is confirmed. A study carried out by Faria et al. 24 on exposure to PM2.5 of children in public spaces, in the city of Lisbon, found values of 19 μg/ m3; pollution that was associated with coal combustion processes at an industrial level. This value exceeds the daily value of the World Health Organization (WHO) of 15 μg/ m3 (25.
b. Morphology of CFCs
Some images taken in the SEM microscope are shown in Figure 5; where the CFC3 sample presented an irregular granular morphology (Figure 5a). Likewise, irregular shapes attached to fine particles were observed for CFC2 (Figure 5b). Regarding CFC1 ash, irregular particles along with a porous spherical shape were observed for CFC1 (Figure 5c). Furthermore, irregular hexagon structures were observed (Figure 5d).
Coal combustion processes give rise to different morphologies 15. In this sense, CFCs are characterized by being granular with an irregular hexagon structure and visibly porous 13,26-28. The morphology of unburned carbon in CFC appears as irregular shapes for the same technique 29. Silva & Da Boit 19 studied CFCs using SEM and found concentrations of ultrafine spherical particles and nanoparticles smaller than 50 nm, rich in silica crystals and predominance of fine quartz grains; where exposure to these crystals is related to the incidence of lung cancer.
c. Chemical and Elemental Composition
The chemical composition of the ashes of the study is presented in Table 1, where it is observed that the ashes are mainly composed of SiO2, Al2O3 y Fe3O4, in low quantities they present CaO, MgO, Na2O5, SO3, and in smaller quantities metal oxides such as MnO, P2O5 and K2O as well as elements of Ni, Sr and toxic metals such as Pb and Zn of concern for human health 15,27,30-33. Some authors mention that coal ash from brick manufacturing is extremely toxic to human health and that cancer can be contracted from exposure to elements such as chromium 34.
Another even more worrying effect is DNA damage related to heavy metals, such as Al and Si 2. Coal ash is also composed of rare earth elements and yttrium (Y) present only in inorganic components such as amorphous silica, and lesser quantities in fly ash spinel. However, in this study, the presence of Y was also found in the ashes studied 16.
Table 1 Characteristics of the bottom ash studied.
Characteristics (% p/p)) | CFC 1 | CFC 2 | CFC 3 |
---|---|---|---|
SiO2 | 59.04 | 63.12 | 59.86 |
Al2O3 | 22 | 24.15 | 24.24 |
Fe2O3 | 8.64 | 4.76 | 7.16 |
SO3 | 3.8 | 1.58 | 2.76 |
CaO | 1.77 | 1.85 | 1.39 |
TiO2 | 1.32 | 1.3 | 1.3 |
P2O5 | 1.12 | 1.05 | 1.01 |
K2O | 0.92 | 0.82 | 1.11 |
Na2O | 0.41 | 0.47 | 0.38 |
MgO | 0.35 | 0.39 | 0.33 |
Cr | 0.18 | 0.02 | 0.03 |
Ba | 0.13 | 0.15 | 0.15 |
MnO | 0.03 | 0.02 | 0.01 |
V | 0.02 | 0.03 | 0.02 |
Cu | 0.01 | 0.02 | 0.02 |
Pb | 0.01 | 97 ppm | - |
Zn | 76 ppm | 51 ppm | 46 ppm |
Se | - | 28 ppm | - |
Sr | 0,11 | 0,1 | 0,12 |
Ce | 0,06 | 0,09 | 0,04 |
Y | 81 ppm | 57 ppm | 60 ppm |
Loss On Ignition (LOI, %) | 22.81 | 16.65 | 30.62 |
Source: authors
Pb was found in the CF2 sample, this metal in the environment is in its oxidation state. Human exposure to this element causes damage to the kidneys, heart, and nervous system, risk of delay in babies, and even abortions 5.
The presence of metals in CFCs is due to their high melting points, therefore they do not volatilize and cause incomplete combustion 35. The problem is further aggravated because the body cannot digest the carbon components, causing bioaccumulation, prolonging the pro-inflammatory condition that leads to cell death, followed by the blood coagulation process that triggers cardiovascular accidents such as thrombosis and arteriosclerosis 2. Ti was also found in the CFC waste from the study, according to studies by Silva et al. 36 Ti in coal ash can be found in more than 30 amorphous phases in nanometer sizes, which can mix with soil and form urban dust. This element presents a different reactivity with the potential for carcinogenic risk, which leads to particular behaviors, especially in the health of children who frequent public spaces.
Mondal et al. 34 studied the bioavailable fractions in soils near brick kilns, the highest fractions were for Mn, Zn, Pb, and Cu and the lowest for Cd, and Cr; the soils were moderately acidic, which was confirmed by the calculation of the contamination index (PI), which classified the study area as extremely contaminated. Another index was the ecological risk index (ERI), which resulted in extreme ecological risk.
The unburned carbon content was also measured with the loss on ignition (LOI), for which 22.81%, 16.65%, and 30.62% were obtained (see Table 1) for CFC 1, CFC 2, and CFC 3 respectively. Similar results have been reported by other authors 27,29, which is attributed to proportions of unburned coal; where the latter has been reported with high levels of PHA 13. Concerning toxicity, it has been found that one of the most frequent occupational risks is the risk of contracting cancer due to exposure to byproducts of coal combustion, due to the content of PHA and radioactive natural radionuclides, either by ingestion or dermal contact 4,37.
d. Ecotoxicity test
The phytotoxicity of commercial soil amended with the CF2 ash sample was studied in Vigna radiata. According to what is reported in Table 1, this ash contains metals such as Cr, Ba, V, Cu, Zn, Sr, Ce, Y, and Pb. Studies report that ashes from coal combustion have a high adsorption capacity, which allows them to encapsulate several dangerous elements. This characteristic influences the bioavailability of metals and the growth of plants 1.
Figure 6 shows images of radicle growth at concentrations of 0, 50, and 100%; where elongation showed a dose-response of inhibition at higher concentrations, with the 50% concentration being the one with the lowest radicle elongation followed by the 100% ash concentration. However, the ANOVA analysis showed no significant differences between all levels of ash concentration. Other ecotoxicological studies report that elongation for Vigna radiata is inhibited at higher CFC concentrations, possibly by division of apical root meristematic cells. It has also been found that the morphology of the roots was also indicative of toxicity, due to their curved, thin, fragile, and sticky root characteristics, as seen in Figure 6b, c 22.
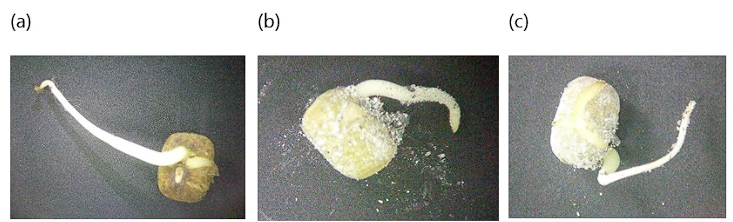
Source: authors
Figure 6 (a) radicle in soil without ash, (b) radicle in soil in 50% ash concentration., (c) radicle in soil in 100% ash concentration.
The percentage of radicle reduction was more noticeable in the concentration of 50% to 100% ash (see Figure 7). The germination rate is lower at ash concentrations of 50%, however, at a concentration of 100% there is a little more germination (see Figure 8).
The phytotoxicity of coal ash also depends on soil properties, with sandy soils being more susceptible to a phytotoxic response due to their low chemical buffering compared to clay soil 11. The toxicity of coal ash has also been studied in Brassica rapa (Pakchoi) cultivation, where contamination such as Pb, Cr, and Cu was shown in the soil, a characteristic of care for the food chain is that the edible parts of the plant presented higher heavy metal content than roots 38.
Samples CF1 and CF2 presented an alkaline pH of 7.03-7.06 in their order, and sample CF3 showed a slightly acidic PH of 6.35. This parameter, together with the content of soluble salts in the coal byproducts, has growth inhibition effects on plants. Therefore, care must be taken with the lime requirements in the soil 39. Alkaline pH can generate low bioavailability of essential nutrients, for this reason, growth and biomass decrease 11. Alkaline pH can also reduce the bioavailability of metals 21. An acidic pH in coal ash can lead to total organic carbon (TOC) and S in the source coal 29. The literature reports on the application of ash as a soil amendment, however, this should not be generalized because investigations of the physical and chemical characteristics of the site need to be carried out before use.
Regarding the conductivity values for CF1, CF2, and CF3, they were 215, 230, and 200 µS/cm respectively. The conductivity behavior has been studied in coal ash, where neutral reactions were obtained as a result of greater electrical conductivity, also indicative of the abundance of soluble salts of basic cations such as Ca 2+, Na +, and K + among others 29. Consequently, the alkaline behavior of CF1 and CF2 may be due to high conductivity values, however, the slightly acidic pH of sample CF3 may be due to the TOC and S content in the original coal, which tends to develop acidity.
4. CONCLUSIONS
The analysis of coal bottom ash through different microscopy techniques was able to reveal the content of particulate matter of environmental interest PM10 and PM2.5 as well as its granular and irregular morphology with some spherical particles. Exposure to this type of particle can cause adverse effects on human health, especially respiratory conditions.
The difference in physicochemical properties in the three samples may be due to the coal combustion conditions. For this reason, CFCs generated in different industrial processes can differ substantially in their characteristics, which is why it is necessary to carry out characterization studies that allow control for the elimination of these materials, thus reducing the environmental impact and public health, especially in communities of lower resources.
The parameters of the toxicity test for CF2 showed radicle reduction of Vigna radiata at higher ash concentrations, the germination index decreases up to 50% ash concentration but increases slightly for 100% ash concentration. Therefore, this sample could be compatible with agricultural use. However, prolonged use of these materials can affect soil characteristics such as acidification and deposition of elements.
It is recommended to carry out future research that contributes to delving deeper into the issue of the use of ashes for agricultural applications, where the appropriate concentration is standardized in different types of soil for agricultural use. In that sense, this industrial waste would be used, since it is currently not being used in its entirety.