Introduction
Agriculture is recognized as one of the causes of biodiversity loss (Foley et al., 2005; Power, 2010). However, agroecosystems, which encompass the interaction of physical, biological, socioeconomic and cultural subsystems (León et al., 2018), have the potential to contribute to biodiversity conservation (Perfecto et al., 1996; Perfecto et al., 2003; Schroth and Harvey, 2007). For instance, the availability of fruit and flower resources in agroecosystems enhances bird biodiversity (Hole et al., 2005; Perfecto et al., 2003), the input of litter affects the microorganism diversity (Sauvadet , 2019), and the presence of shade trees increases epiphytic diversity (Hietz, 2005). In the agroecosystems of Latin America, farmers remove epiphytes (e.g. orchids, ferns, and bromeliads), because they believe that epiphytes damage the tree crowns (Cruz-Angon and Greenberg 2005; DaRocha et al., 2015); this practice may reduce epiphytic diversity and the contribution of agroecosystem to biodiversity conservation.
In the context of bromeliads, the arrangement of the leaves forms a tank that collects rainwater and detritus from the canopy (Araujo et al., 2007; Montes de Oca et al., 2014). Within the bromeliad, a detritus-based aquatic food web develops (Becker et al., 2010). The decomposition of detritus releases fine particulate organic matter, which serves as a resource for the immature stages of mosquito species (Frank and Lounibos, 2009). Mosquito larvae also consume protozoa and algae (Souza et al., 2016; Travanty et al., 2019; Walker et al., 1988). Predators, such as Odonata nymphs, feed on mosquitoes and help regulate their populations (Becker et al., 2010). Additionally, interspecific competition among mosquito species plays a role in population size moderation (Haq et al., 2019). Bromeliads in agroecosystems can contribute to herbivore control through ecological interactions with ants (Rost-Komiya et al. 2022). Bromeliads in agroecosystems may serve as habitats for mosquito genera such as Toxorhynchites, Wyeomyia, Limatus, Onirion, Runchomyia, and Anopheles (Kerteszia), as well as disease vector mosquitoes of the Aedes genus (e.g., O’Meara et al., 2003; Mocellin et al., 2009; Ceretti-Junior et al., 2016). Disease vectors like Aedes aegypti, Ae. albopictus and Culex quinquefasciatus can breed in water bodies within agroecosystems (Muturi et al., 2006; Ohba et al., 2015; Foster and Walker, 2019) and may utilize bromeliads within agroecosystem as breeding site.
The tropical Andes of Colombia is one of the biodiversity hotspots (Myers et al., 2000), and harbors a high diversity of bromeliad (Betancur and García, 2006) and mosquitoes (Rozo-Lopez and Mengual, 2015). Despite its relevance for biodiversity, anthropogenic perturbations and the increase in the human population has transformed the tropical Andes ecosystems. The conversion of theses ecosystems to agriculture area has reduced the diversity of bromeliads and the habitat for mosquitoes, therefore trees in agroecosystems with bromeliads could contribute to conservation of mosquito diversity. Our goal was to determine the Culicidae species inhabiting the tanks of Tillandsia elongata in an agroecosystem of the Colombian Andes, based on taxonomic and molecular methods.
Materials and methods
Study area: We recorded the immature mosquitoes of the agroecosystem “Montelindo” in Santágueda (Palestina, Caldas, Colombia) located at 1050 m.a.s.l. on the western slope of the Central Andes Mountain Range (5°05’ N, 75°40’ W). It experiences an annual mean temperature of 22.5°C and the annual mean precipitation of 2 100 mm, with a bimodal rainfall pattern, with rainy seasons occurring in April-May and September-December (Jaramillo et al., 2011). The agroecosystem covers an area of 64 hectares and includes crops such as orange (Citrus X sinensis Osbeck), mandarin (Citrus nobilis Lour.), corn (Zea mays L.), and soy (Glycine max L.), as well as isolated mango trees and Guadua bamboo forests. In particular, the citrus and mango trees harbor individuals of Tillandsia elongata (Bromeliaceae).
From March to December 2018, we collected 48 bromeliad tanks inhabited by T. elongata at heights ranging from 1 to 4 m. To collect the immature mosquitoes in each bromeliad, we filtered the bromeliad water using a 150 µm-mesh sieve. Subsequently, each bromeliad leaf was cleaned using a brush (Ospina-Bautista et al., 2004). The collected specimens were preserved in separate 50 mL vials.
Morphological identification: We taxonomically identified the immature mosquitoes to the genus level. To allow them to reach maturity, we reared 21 immature mosquitoes in the Entomology Laboratory of Universidad del Valle (Cali, Colombia). For breeding, we used a 100 mL-plastic cup filled with distilled water and covered it with a 0.2 mm mesh. To prevent microbial contamination, we replaced 50 % of the water every two days. As food resources, we provided rabbit feed pellets or Aedes larvae every 48 hours. The exuviae generated during the breeding process were also collected and preserved in 75 % ethanol for taxonomic identification. The emerged adults mosquitoes were stored at 4°C (Gómez et al., 2015). We employed various characteristics, including coloration patterns, scale positions, proboscis length and shape, and setae coloration, size, and position, to identify the adult mosquitoes. For species determination, we referred to specialized literature, such as Lane (1953), Peyton et al. (1983), and The Walter Reed Biosystematics Unit (WRBU). To observe the structures necessary for species identification, we prepared mounts of male genitalia using 10 % KOH, acid fuchsin dye, and clove oil (Marcano et al., 1986). Photographic records were captured using a Canon EOS Rebel T5i camera mounted on a Nikon Eclipse Ci microscope, and Helicon Focus software version 7.5.4 (https://www.heliconsoft.com/heliconsoft-products/helicon-focus/).
Molecular determination: We performed molecular analyses on two individuals from each morphotype. DNA isolation was conducted using the DNeasy Blood and Tissue kit (Qiagen), following the manufacturer’s instructions. To amplify a ~710 bp DNA barcode sequence of the 5’ region of the cytochrome oxidase I (COI) mitochondrial gene, we utilized standard primers for invertebrates: LCO1490 (F) 5’-GGTCAACAAATCATAAAGATATTGG-3’ and HCO2198 (R) 5’-TAAACTTCAGGGTGACCAAAAAATCA-3’ (Folmer et al., 1994). The resulting PCR products were separated via 1 % agarose gel electrophoresis with TBE 1X pH 8.0 at 110v/50mA, stained with SYBR® Safe dye, and visualized using a Gel Doc-It2 310 (UVP) photodocumenter. Purification of the PCR products was performed with Wizard® SV Gel and PCR Clean-Up System (Promega), following the manufacturer’s instructions, and subsequently, Sanger sequencing was conducted by Macrogen (South Korea). The obtained DNA sequences were analyzed and edited using Geneious Trial v8.14 (Drummond et al., 2009) and searched against the GenBank database using BLAST. Sequence alignments were generated with ClustalW (Thompson et al., 1997) in MEGA version X (Kumar et al., 2018). Phylogenetic analyses were performed using Maximum Likelihood (ML) with the Kimura (K2P) model and 1000 Bootstrap replicas in MEGA X.
Results
We found a total of 53 immature mosquito specimens, with 20 belonging to the genus Toxorhynchites Theobald, 1901 (Culicinae: Toxorhynchitini) and 33 belonging to the genus Wyeomyia Theobald, 1901 (Culicinae: Sabethini) (Fig 1, Fig 2, Fig 3). Within each bromeliad, we observed a maximum of five Toxorhynchites individuals and four Wyeomyia individuals. Toxorhynchites larvae were collected from 11 bromeliads, while Wyeomyia larvae were present in 20 bromeliads. Additionally, Toxorhynchites and Wyeomyia larvae co-occurred in three bromeliads. Based on the morphological characteristics of adult females and male genitalia, we identified Toxorhynchites haemorrhoidalis (Fabricius) and Toxorhynchites bambusicola (Lutz and Neiva).
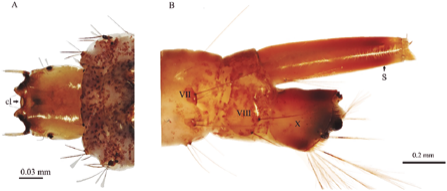
Figure 1 Toxorhynchites sp. fourth stage larva. (A) Dorsal view of head (B) Lateral aspect of abdominal segments VII, VIII and X. cl = Clipeus; S = respiratory siphon.
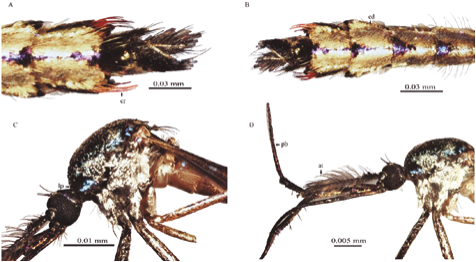
Figure 2 Toxorhynchites. (A) Tx. haemorroidalis dorsal view abdominal terminalia; (B) Abdomen, dorsal view of Tx. haemorroidalis; (C) Thorax and head, lateral view of Tx. bambusicolus; (D) Thorax, head and antenna, lateral view of Tx. bambusicolus. (cr) Setae; (ed) Scales; (pb) Proboscis; (at) Antenna; (lp) pronotal lobe.
The DNA sequence analyses on six individuals confirmed the identification of Toxorhynchites and Wyeomyia. However, we were unable to achieve species-level identifications as the sequences showed less than 97 % similarity to GenBank sequences of known species. The BLAST search results indicated that the partial COI gene sequences of the Toxorhynchites sp. were similar to Tx. haemorrhoidalis [MF172401], while the partial COI gene sequences of Wyeomyia sp. were related to Wy. pertinans (Williston) [MN997640] and Wy. mitchellii (Theobald) [MF950916] (Fig 4). The partial COI gene sequences were deposited in GenBank under accessions MT920486-MT920490.
Discussion
This study presents the first record of Toxorhynchites spp. and Wyeomyia spp. in bromeliads of agroecosystems. The abundance of mosquitoes in T. elongata was found to be low compared with studies conducted in urban and natural ecosystems of Brazil and Venezuela (Navarro et al., 2007; Marques and Forattini, 2008; Cardoso et al., 2015). This can be attributed to due to the smaller size of T. elongata and the environmental conditions present in the agroecosystem. The water-holding capacity of T. elongata tanks and the amount of leaf litter are lower compared to other Tillandsia species found in Colombia (Ospina-Bautista et al., 2004; Ospina-Bautista et al., 2008, IAVH, et al. 2006). Furthermore, the high temperature and low precipitation in the study area also contribute to reduced water availability in the tanks.
The physiological constraints of mosquito species determine the suitability of habitats for each species, thereby influencing their populations and communities (Medeiros-Sousa et al., 2020). In aquatic ecosystems, factors such as temperature, water availability, and depth play significant roles in the development of mosquito larvae (Lester and Pike, 2003; Ciota et al., 2014). As temperatures rise, the developmental times of immature stages decrease (Grech et al., 2015; Agyekum et al. 2022). Additionally, larval survival varies with temperature; for instance, an increase in temperature from 16.6 to 25.2°C favors the survival of Cx. quinquefasciatus (Grech et al. 2015), while Anopheles gambiae survival decreases between 25 and 36°C (Agyekum et al. 2022). Water availability and depth in bromeliads also influence insect density, biomass, and growth due to the sensibility of insects to drought conditions (Amundrud, 2015; Dézerald et al., 2017). For instance, larvae of Aedes triseriautus develop faster in ecosystems with low water depth (Juliano and Stoffregen, 1994).
Toxorhynchites species lay their eggs in various bromeliad species, such as Aechmea distichantha, A. aquilega, A. nudicaulis, Billbergia nana, Neoregelia compacta, Quesnelia quesneliana, Catopsis berteroniana, Tillandsia guatemalensis, Vriesea philippocoburgi, and V. splendens (Jabiol et al., 2009; Mocellin et al., 2009; Cardoso et al., 2015; Docile et al., 2017), as well as other phytotelmata, including tree holes and bamboo internodes. In our study, we observed Toxorhynchites ovopositing in T. elongate of the agroecosystem, which is commonly used by Toxorhynchites species. The predatory behavior of Toxorhynchites larvae could influence the insect community and the ant populations (Dézerald et al., 2015). Toxorhynchites larvae prey on other Diptera, such as sandflies and flower fly larvae, thereby reducing the insect richness bromeliad tanks (Lounibos et al., 1987). The predation of mosquito species by Toxorhynchites larvae could explain the low co-occurrence between Toxorhynchites and Wyeomyia in T. elongata. Some mosquito species exhibit antipredator behavior and avoid ovopositing in bromeliads with presence of the predator (Ferrari et al., 2008; Roux et al., 2014). Furthermore, Toxorhynchites species serve as biological control agents (Trimble and Lund, 1983). Therefore, the presence of Toxorhynchites larvae in bromeliads could reduce the colonization of the population of disease vector mosquitoes.
The cannibalistic behavior of Toxorhynchites (Campos and Lounibos, 2000) and the characteristics of T. elongata could explain the low abundance of Toxorhynchites larvae per bromeliad. Toxorhynchites can control their populations by consuming their own eggs and immature stages (Linley and Duzak, 1989). The arrangement of bromeliad leaves restricts the free movement of larvae between receptacles, reducing their access to prey and affecting larvae survival. However, when the water level in the bromeliad exceeds its maximum capacity (flooding), predator larvae can move freely between receptacles (Lounibos et al., 1987). In our study site, temperatures above 20°C and low precipitation reduced the frequency of flood events in bromeliads, thus affecting the number of Toxorhynchites larvae inhabiting them.
We morphologically identified two species of Toxorhynchites, namely Tx. bambusicola and Tx. haemorrhoidalis. However, at the molecular level, it was not possible to identify the sequences obtained at the species level. Tx. bambusicola is found in bromeliads in Brazil (Ceretti-Junior et al., 2016) and Colombia (Rozo-Lopez and Mengual, 2015), as well as in bamboo internodes in Argentina and Ecuador (Campos, 2013; Linton et al., 2013). Tx. haemorrhoidalis oviposit in bromeliads of the Aechmea genus in Venezuela, French Guiana, and Argentina (Lounibos et al., 1987; Campos, 2011; Talaga et al., 2017), as well as in bamboo internodes, palms, and Heliconia bracts (Lounibos et al., 1987; Hutchings, 1994; Linton et al., 2013). Previous studies have found Toxorhynchites haemorrhoidalis in the departments of Casanare and Valle del Cauca of Colombia (Barreto and Lee, 1969; de Hoyos et al., 2000). Our results extend the distribution range of Toxorhynchites species and the bromeliad species as a breeding site for Tx. bambusicola and Tx. haemorrhoidalis.
Species of the genus Wyeomyia inhabit tank bromeliads in Brazil and Venezuela (Cardoso et al., 2015; Navarro et al., 2018). The DNA sequences of individuals from the genus Wyeomyia showed similarity to two GenBank sequences of Wy. mitchelli and Wy. pertinans. Both species inhabit bromeliads. Wy. mitchelli inhabits bromeliads of Central America (Ibañez-Bernal, 2011), while Wy. pertinans lays its eggs in bromeliads in Venezuela, French Guiana, and Colombia (Navarro et al., 2007; Talaga et al., 2017; Rozo-Lopez and Mengual, 2015). Our results show that Wyeomyia larvae utilize T. elongata as a breeding site. In this habitat, Wyeomyia larvae coexist with Tipulidae larvae, which are shredder insects that fragment litter into fine particulate organic matter, thereby increasing bacterial density (Heard 1994) and serving as food resources for Wyeomyia larvae.
We found that bromeliads in agroecosystems are breeding sites for mosquitoes of the genera Toxorhynchites and Wyeomyia. Citrus crops have the potential to offer resources such as nectar for the adults of these mosquito species (Collins and Blackwell 2000; Fischer et al., 2016). Consequently, promoting the cultivation of diverse crops in agroecosystems and conserving bromeliads that are associated with trees can create favorable conditions for the breeding and sustained populations of Toxorhynchites and Wyeomyia mosquitoes.