Since the beginning of agricultural development, during Neolithic era, humans influenced the properties of virgin lands. This land use is one of the main drivers of many processes of environmental change, as it had influenced basic resources within the landscape. Without a doubt, the anthropogenic factor has played its role as a force that affects both soils and climate, having increased exponentially during the last 300 years, to such an extent that this phenomenon is now considered a new era in the evolution of the Earth: “The Anthropocene” (Crutzen and Steffen, 2003). During the last millennium, nearly all soils have undergone some modifications through human action, directly or indirectly. Thus, at present, the genesis of many soils has been determined more from human effects than from natural forming factors. Such is the magnitude of this transformation that some authors speak of an agrogenic evolution of soils (Lebedeva et al., 2008).
Tropical regions are characterized by having high rainfall intensities resulting in severe erosional rates if inappropriate land management practices are applied. In Cuba, there are extensively weathered tropical soils (Ferralsols, Nitisols, Oxisols, Lixisols, Acrisols, and Alisols)”. It is a country with soils subjected to anthropogenesis, constituting one example for tropical regions. I Cuba being a long-narrow island presents diverse soil groupings, dominated by the Nitisols, especially in the western and center of the country, which because of its good characteristics, has been the main source of food production for the inhabitants of this region. (Hernández et al., 2017). In the last 10 years some Cuban researchers has been working on the diagnosis for soil degradation, in order to achieve their sustainability through medium and long-term research on the basis of the so-called reference sectors (Hernández et al., 2006). Some of these results were presented by Hernández et al. (2009, 2013) and by Olivera (2017), about the change of Red Lixiviated Ferralitic soils properties (Nitisols) by continued cultivation. These authors show indicators and mechanism of soil degradation as well as agroproductive responses to improvement.
Changes in soil functionality are indicators of their performance and handling to which they are subjected. However, it should be noted that if a better understanding is to be achieved then studies of each soil type must be conducted., It is also noted that among soil samples with similarities in their relationships and some dependency, which makes it possible for the interpretation of one, to infer the behavior of another (Fultz et al., 2013; Duval et al., 2016). According to information from the Soil Institute of Cuba (2006), 69.6% of soils have low organic matter (OM) and 43.3% have strong erosion, limiting their productivity. In this sense the pursuit of quality of soil and timely identifying their limitations is one of the main objectives of research in soil physics, as demonstrated by (Zhu et al., 2015; Deng et al., 2016). Although significant knowledge about soil and landscape change in agriculture has been gained, much remains unknown and uncertain. Major reasons are the relative scarcity of soil studies, methodological shortcomings, the complexity of agricultural systems and soils, and imprints of multiple land use and environmental change. With this purpose, the aim of this study was to evaluate the influence of different tropical soil management systems reflected in their physical, chemical and hydro-physical properties.
MATERIALS AND METHODS
The study was carried out in Banao community in the Sancti Spiritus Province, which is located to the south in the central region of Cuba, between X: 216 000 - 218 000 and Y: 539 000 - 550 000 according to the rectangular plane coordinate system of Northern Cuba, cartographic sheet 4 281-II-b (Figure 1). This experimental area (“Hermanos Alonsos”) has suffered anthropic action for more than 30 years, despite the high income generated by the production of onions (Allium cepa L.). The production levels obtained in the last years have compromised the ecosystem’s stability and as a vulnerable factor increased soil erosion (Figure 2).
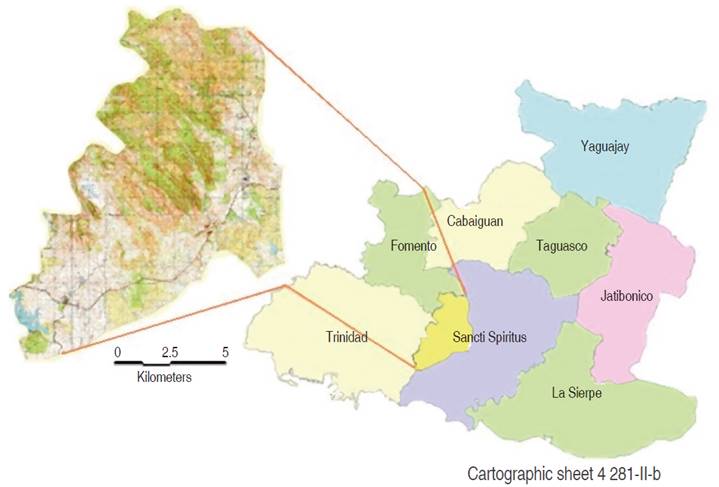
Figure 1 Cartographic sheet generated from the Map Info Professional 9.0 software (2010) (Banao comunity).
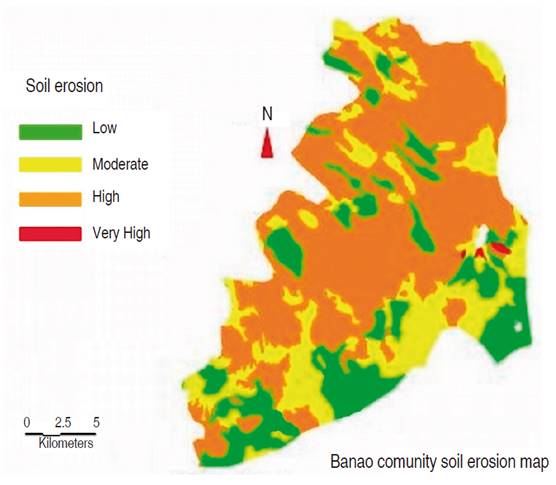
Figure 2 Soil erosion using the 1:25 000 map generated from the MapInfo Professional 9.0 software (2010).
We analyzed nine Nitisol profiles where three conditions were considered as treatments (three repetitions for each land use) grouped as: (I) native forest (Benchmark > 30 years); (II) soils formerly cultivated then turned to pasture (Conservation > 10 years); and (III) soils under continuous cultivation (Agrogenic > 50 years). The profile description was according to the manual for detailed cartography and integral evaluation of soils (Hernández et al., 1995). Soils were identified by the World Reference Base for Soil Resources (WRB) classification (International Union of Soil Sciences (IUSS), Working Group, 2014).
Soil samples were taken every 10 cm up to 50 cm deep for every profile. All soil samples were analyzed at the Territorial Station of Investigations of the Sugar Cane (ETICA), Villa Clara, Cuba, in accordance with the following analytical methods: organic matter (OM) according to (Walkley and Black, 1934); color profile (Figure 3) by (Munsell Soil Color Charts, 2000), bulk density (Db) was determined for a humidity close to the field capacity considering that some soils contain dilatable clays in which the humidity level exerts a big influence on their values. (Db) was measured using 100 cm3 cylinders (Grossman and Reinsch, 2002) and soil particle density (Dp) was determined according to the pycnometer method. Field capacity was obtained from periodical measurements of soil water content (Simeon, 1979). Soil texture Hydrometer Method (Bouyoucos, 1951) and structural index was determined according to Pieri (1995). The total porosity (P) was calculated using the mathematical model represented below [1].
Variance analysis (ANOVA) tests were carried out on the results considering a completely random design in which the profiles of each category were considered repetitions and each of the three conditions were considered treatments. Physical soil measurements were compared by the Tukey test, at 5% probability. Relationships between bulk density, field capacity, organic matter and structural index were investigated by regression models. All statistical analyses were performed using the statistical software Agroestat (Barbosa and Maldonado, 2011).
RESULTS AND DISCUSSION
The results demonstrated that the soils in this study presented differences in their physical properties due to its land utilization (Table 1). According to (Hernández et al., 2010), soil degradation processes increase where the management practices are selected without regard for the edaphological conditions, which are specific to every particular region. Benchmark topsoil (0 - 10 cm) had a mean (± standard deviation) bulk density of (0.90 ± 0.09 g cm-3), which was similar to that of topsoils taken from conservation profile (0.94 ± 0.06 g cm-3). The mean bulk density for Benchmark and Conservation profile were lower (P<0.05) than the bulk density values for agrogenic profile (1.21 ± 0.04 g cm-3). Bulk density values both for Benchmark and Conservation profile are considered normal, whereas for the agrogenic profile the soils are considered compacted according to Martin and Duran (2011). A tendency towards a lower bulk density for soils taken from Benchmark and the conservation profile system compared to those from agricultural land uses (agrogenic) could also be detected for the 10-50 cm (Table 1). Compaction, measured by increased bulk density, often accompanies structural degradation as soil porosity and pore size are reduced through physical compression and decreased organic matter. According to Homburg and Sandor (2011) in the Mimbres study, bulk density increased about 9% relative to uncultivated soils and may not have been a major problem, but that degree of compaction has been shown in experiments to inhibit maize seedling development and root elongation, especially in fine-grained soils.
Soil particle density (Pd) follows a similar behavior in all profiles without revealed significant differences. This could be explained because the soil macro particles can change in a short time with the management practices, but it does not happen in the same way with microparticles and soil texture. On the other hand, soil porosity did not present significant differences between the benchmark and conservation profile, but with the agrogenic profile. Higher bulk densities are usually attributed to compaction, structural damage and destruction of macropores of topsoil by overgrazing and use of machinery (Kelishadi et al., 2014; Lal, 1986; Price et al., 2010) or by intensive agricultural practices (Emadi et al., 2008). Our results are thus consistent with previous studies that reported higher bulk densities for pastures or croplands compared to native or forest plantations in Argentina, the north-eastern USA (Zhou et al., 2008), Costa Rica (Reiners et al., 1994), Iraq (Emadi et al., 2008) and Ethiopia (Selassie and Ayanna, 2013).
According to the regression analyses, as the bulk density increases, the field capacity decreases and the observed tendency is linear for Benchmark and Conservation soils (R 2=0.94) and (R 2=0.93) respectively (Figure 4A, B). That is, the field capacity decreases 42.6% for Benchmark profile and 49.1 % for Conservate profile for each unit of soil bulk density variation. It a polynomial regression can be observed For the Agrogenic profile (R 2=0.83) (Figure 4C). This non-linear behavior corresponding to the Agrogenic profile could be influenced by higher values of bulk density, presenting a greater soil compaction, lower structural index, organic matter content and porosity coinciding with a compacted soil according Martin and Duran (2011) as was previously described.
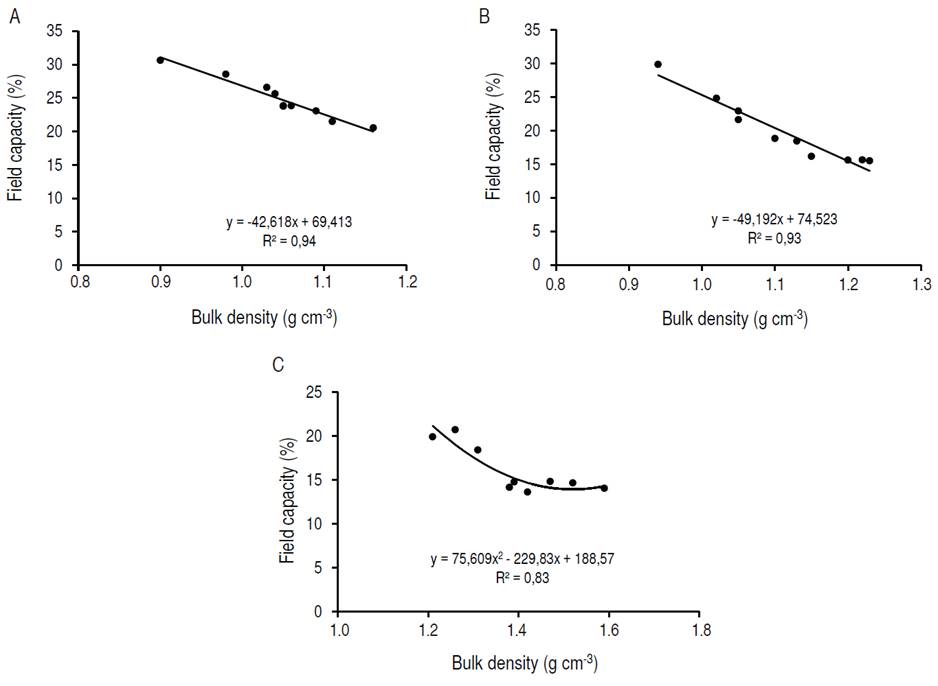
Figure 4 Relationship between field capacity and bulk density: A. Benchmark profile, B. Conservation profile, C. Agrogenic profile.
The texture of all land types and depths were predominantly clayey, with the clay content increasing with depth (Table 2). The Soils of all depths sampled were classified as (Nitisol Rodico Eutrico) based on (IUSS, 2014). On average, soils from the lower depths (25-50 cm) contained slightly less sand and slightly higher clay content (Table 2). According to Homburg and Sandor (2011) structure degradation is signaled by loss of granular structure, weaker structure grade, tendency toward larger aggregates (peds) and massive condition. These differences appear to be due to intensive anthropogenesis experienced by the soils which was not sub soiled in the last years. It is therefore reasonable to infer that the soil compaction is conditioned by the history of land use and management. According to Olivera (2017), as soil compaction increases under heavy farm machinery, the soil physical structure deteriorates, bulk density increases and total porosity decreases.
The organic matter content and the structural index shows a linear relationship with high degree of dependence (R 2=0.99). Therefore, with increasing organic matter the structural index increases and vice versa (Figure 5). This relation may be more dependent for this type of soil dominated by oxides and 1: 1 mineral compared to soils dominated by 2: 1 mineral (Six et al., 2000). It should be noted that predictions made by using the regression line fitting (R 2=0.99) obtained in this figure produced practically the same results as the regression on individual soil management.
CONCLUSIONS
Our study shows that all soil properties were markedly different between native forest (Benchmark) and the continuous cultivation (Agrogenic). The other land types increased bulk density, decreased field capacity, organic matter, porosity and structural index. Continuous cultivation caused the degradation of the topsoil eventually reaching a 50 cm depth which leads to a greater compaction, porosity limiting and humidity reserve. Benchmark profile did not present significant differences with the conservate profile which we attribute the No-till policy and adequate rest time. Establishing pastures could help reduce bulk density and improve the soil physical properties.