Starch is a polysaccharide macromolecule product of biosynthesis in the form of semi-crystalline granules stored in plant cells as an energy source (Li et al. 2017; Li et al. 2023). Structurally, it consists of linear amylose formed by α-D-glucan-(1,4) bonds but slightly branched (ca. 3-5%), and amylopectin molecule of higher molecular weight organized by α-D-glucan-(1,4)-bonds with a highly branched structure consisting of α-D-glucan-(1,6)-bonds (Li et al. 2023). Short- and long-range molecular studies deduce that single amylose helices generally form intermixed amorphous domains together with branched amylopectin groups, whereas regular packing of amylopectin or amylose-amylopectin double helices forms the crystalline domain (Ma et al. 2019). Amylose provides heterogeneity and significantly influences the structural and retrogradation properties of the granule, negatively affecting the quality and texture of the starch-formulated product (Keeratiburana et al. 2020). Therefore, to achieve the desired properties and meet the requirements of food applications, it is necessary to modify the structure and/or morphology of the granule in its native state.
Enzymatic modification emerges as a green technology to develop clean label products with desired properties such as solubility, retrogradation, and improved thermal stability (Miao et al. 2015). It has gained relevance, especially when performed below the gelatinization temperature, because of its lower energy cost and higher yields (Yang et al. 2019). Granular modification performed by biocatalytic processes can change the physicochemical nature of starch, product of the alteration of morphological properties and semicrystalline order, due to the cleavage of α-D-(1,4) or α-D-(1,6) glycosidic bonds on amylose or amylopectin chains (Li et al. 2017; Gao et al. 2023). Starch is generally hydrolyzed by the exogenous or endogenous action of amylolytic enzymes such as α-amylase (AAM), β-amylase (BAM), amyloglucosidase (AMG), and pullulanase (PUL). AAM is an endo-amylase capable of cleaving α-D-glucan-(1,4) linkages and yielding glucose, maltose, and oligosaccharides (DP<5), as well as branched-limit dextrin of configuration alpha (α) (Gui et al. 2021; Zhou et al. 2021; Gao et al. 2023). BAM and AMG are exo-amylases; BAM cleaves every two units of glucose from the non-reducing end to obtain maltose and branched-limiting dextrin of configuration (β) (Gui et al. 2021; Li et al. 2023), while AMG is capable of hydrolyzing as many α-(1,4) and α-(1,6) glycosidic linkages to maltose and glucose units, excluding the production of boundary dextrin (Yang et al. 2019; Zhou et al. 2021). PUL is a debranching enzyme, capable of producing partially linear short chains (Li et al. 2017; Ma et al. 2019; Semwal and Meera 2023). Thus, the use of polypeptides with amylolytic action has been subordinated to hydrolysis of polymeric chains to obtain soluble starches, granules with porous structures, and slow-digesting starches.
Polymer degradation during enzymatic modification is usually performed on granules in their native state instead of starch paste, processes characterized by low degrees of hydrolysis due to the semi-crystalline structure, which makes it difficult for enzymes to access the substrate. This poses a difficult challenge for enzymatic action, involving diffusion of the enzyme on the granular surface, followed by adsorption of the polypeptide within the granule, and finally initiating the biocatalytic process (Blazek and Gilbert 2010; Almeida et al. 2019; Almeida et al. 2022). Moreover, the exo- and endo-amylolytic action depends on the type of starch due to the intrinsic semicrystalline structure of the granules that confer a certain degree of molecular resistance (Miao et al. 2015). In addition, it has been reported that the rate and extent of depolymerization of starch chains depend not only on the characteristics of the polysaccharide material but also on the preferential and differentiated activities of each enzyme (Blazek and Gilbert 2010; Benavent-Gil and Rossell 2017).
In starch granules from cereals, it has been found that enzymatic hydrolysis starts preferentially by the amorphous growth ring, given the appearance of cracks and lacerations on the granular surface (exo-erosion), and that the inherent micro- and nano-porous structure of the granule influences the opportunity of the enzyme to access the substrate (endo-erosion) (Lin et al. 2016). In contrast, enzymatic action on tuber starches reveals confounding results, where surface exo-erosion phenomena predominate, with no noticeable porosities internally (Blazek and Gilbert 2010; Benavent-Gil and Rossell 2017). Some authors report that the enzymatic action on native starches is dependent on amylose/amylopectin packing, the interaction of close double helices, and the redistribution of amylose in the macromolecular structure of the granule (Lin et al. 2016; Zhou et al. 2021; Li et al. 2023). However, there is limited information about the mechanisms of endo- and exo-amylolytic action of different enzymatic catalysts on tuber-derived starches, especially on native cassava starches; likewise, the effect of various mechanisms of enzymatic action on the amorphous regions in tuber starches has been poorly discussed. In this sense, it is hypothesized that the semicrystalline order and morphological characteristics in hydrolyzed cassava starches depend on the differential action of amylolytic enzymes. Consequently, this research aims to analyze the morphological and structural changes during enzymatic attack on native cassava starch granules using commercial biological catalysts with differentiated mechanisms of action.
MATERIALS AND METHODS
Materials
Native cassava (Manihot esculenta cv M-TAI) starch was supplied by Almidones de Sucre S.A.S (Sincelejo, Colombia). α-amylase from Bacillus licheniformis (Lyquozyme Supra 2.2X®, Denmark), β-amylase from Hordeum vulgare (Optimalt®BBA, China), amyloglucosidase from Aspergillus niger (Dextrozyme®GA, Denmark) and pullulanase from Bacillus licheniformis (Optimax® L-1000, China) were donated by Dupont® and Novozymes®.
Determination of enzymatic activity
Biocatalytic activity was determined using the protocol described by De Schepper et al. (2021) with slight modifications. A 30 mg sample of starch was suspended in 10 mL of buffer solution at a defined pH (Table 1). The hydrolytic reaction was made in 15 mL hermetic tubes under stirring at 150 rpm for 15 min at a fixed temperature. Enzyme activity was estimated as a function of the concentration of reducing sugars released during hydrolysis based on the 3,5-dinitrosalicylic acid method (Salcedo-Mendoza et al. 2018). The protein concentration of the enzyme in the preservation medium was evaluated by the Bradford method using BSA as a standard (0-0.5 mg mL-1) to determine the specific activity. One enzyme unit (U) was defined as the enzyme quantity necessary to release 1.0 µmol of reducing sugar per minute in the assay conditions (Frota et al. 2020). In Equation 1, Ua is the enzyme activity (µM min-1 mL-1), [g] is the reducing sugar concentration (g L-1), VS is the volume of solution (L), Mw is the molar mass of glucose g mol-1, Ve is the volume of the enzyme, and t the hydrolysis time (min).
Determination of kinetic parameters
The enzymatic activity was determined as a function of the substrate concentration (S) corresponding to starch solutions in a range from 0.1 to 100 mM. Hydrolysis under the enzyme concentrations presented in Table 1 was carried out for 15 min under constant stirring at 150 rpm, and at optimum pH and temperature conditions. Reducing sugars released during hydrolysis were determined by the 3,5-dinitrosalicylic acid method using glucose as standard (0.0-2.0 g L-1). The molecular weight of native cassava starch was determined by size exclusion-high-performance liquid chromatography (SEC-HPLC) coupled to multi-angle laser light scattering detectors (Wyatt Technology Inc., Dawn 8+ Heleos, USA) and refractive index (Agilent, G1362A, UK) using pullulan as standard, eluting the samples in ammonium formate (10 mM) with a flow rate of 0.5 mL min-1 (Keeratiburana et al. 2020). Vmax and km as kinetic parameters were estimated using Lineweaver-Burk and Langmuir linearization methods (Torabizadeh et al. 2014).
Starch granule modification
Suspensions of native cassava starch at 100 mg mL-1 in citrate buffer were subjected to enzymatic hydrolysis using commercial polypeptides such as α-amylase, β-amylase, amyloglucosidase, and pullulanase under a specific activity of 10 U mg-1 at 60 °C for 24 h (Figueroa-Flórez et al. 2023). The pH was adjusted to the optimal conditions of maximum biocatalytic activity for each enzyme (Figure 1). At the end of the hydrolysis time, the supernatant was separated by centrifugation, and the sediment corresponding to modified starch samples was dried by forced convection for 8 h at 35 °C to be macerated, stored, and subsequently characterized. To evaluate the enzymatic kinetics of native cassava starch granules over time, hydrolysates were sampled every 15 min during the first 2 h, and then every hour until 24 h of hydrolysis were completed. The sugar release behavior over time was fitted to two-parameter nonlinear empirical models using SigmaPlot software (Systat Software Inc., Germany) (Lin et al. 2016). In Equation 2, t is the time (h), G is the concentration of sugar released (M), A is the maximum asymptote (M), and B is the time to reach half of the maximum asymptote (A).
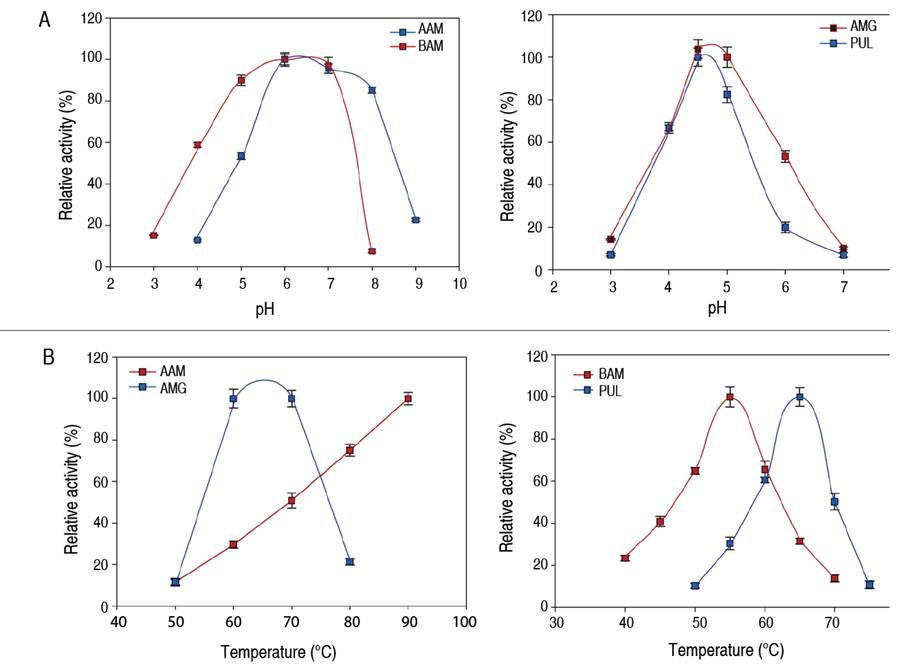
Figure 1 Enzyme activity profile as a function of pH (A) and temperature (B). AAM: α-amylase; BAM: β-amylase; AMG: amyloglucosidase; PUL: pullulanase.
In Equation 3, t is the hydrolysis time (h), G is the concentration of glucose released (M) at time t, and k is the hydrolysis rate constant (h-1). The absolute value of a can be assumed to be the specific biocatalysis coefficient of an enzyme.
Morphological characteristics
The morphological characteristics of native and modified starch were analyzed by scanning electron microscopy (JEOL, JSU LV-5600, Japan) on samples previously fixed on electro-conductive carbon strips, coated with a platinum/gold alloy (Almeida et al. 2022). Photomicrographs were acquired at 15 kV and 30 mA, using magnification approaches at 1,000 and 3,000X.
Amylose content
Starch samples were dissolved in 95% DMSO solution and then precipitated in 90% alcohol. Amylose content was determined using the iodine staining method and estimating the absorbance in a UV-VIs spectrometer at 620 nm by preparing a calibration curve (0-100% w/v) using potato amylose and corn amylopectin (Sigma Aldrich, USA) (Li et al. 2017).
Molecular order by spectroscopy FT-IR
The FTIR-ATR spectra were acquired by single rebound attenuated total reflectance spectroscopy using a diamond crystal with a diameter of 1.5 mm (UATR, PerkinElmer, USA). Samples were processed in triplicate and each spectrum was recorded with a resolution of 4.0 cm-1 and 64 readings in the range of 600 to 4,500 cm-1 (Gao et al. 2023). Spectra were processed including baseline correction, automatic smoothing, and normalization of absorbance with respect to the starch fingerprint in the 1,100-900 cm-1 region. The band ratios 1,047/1,022 cm-1 and 995/1,022 cm-1 were used to estimate the short-range ordered starch structure (OM1 and OM2, respectively), expressed as percentages according to Ma et al. (2019).
Diffraction patterns and crystallinity degree (CD)
X-ray diffraction patterns were obtained using a diffractometer (Panalytical, X'Pert MPD, Switherland) operated at 1.8 kW and 40 mA. The diffractograms were acquired over a range of 4-40°, at a scan rate of 2.0° min-1 and a sampling interval of 0.02° (Davoudi et al. 2022; Figueroa-Flórez et al. 2023).The data were then smoothed using the Savitzky-Golay algorithm and the deconvolution process was developed using the Gaussian function in the 5-30° region. The degree of crystallinity (CD) was estimated as the ratio of the areas of the crystalline peaks over the total area of the diffractogram, using numerical integration methods and MATLAB software (MathWorks, R2019a, USA).
RESULTS AND DISCUSSION
Enzymatic activity of biocatalysts: pH and temperature
The activity of commercial enzymes using native cassava starch as substrate was evaluated as a function of pH and temperature (Figure 1). Bacterial AAM presented higher enzymatic activity in a wide pH range from 6-8 and reached the best conditions at pH=6. Plant BAM presented a wide plateau of maximum values of biocatalytic activity in a pH range from 5-7, and the best conditions were found at pH=7. Polypeptides such as AMG and PUL presented higher enzymatic activities in the pH range from 4-5, reaching a maximum peak of activity at pH=4.5. It is evident that AAM presents higher stability to acidic conditions in the pH range from 5-8 compared to the rest of the polypeptides. In addition, the concentration of H+ ions altered the alkaline-tolerant stability of the enzymes, affecting their enzymatic activity. This behavior is consistent with that reported by Kikani and Singh (2015), who demonstrated that pH changes altered the protein structure, affecting the three-dimensional shape of the enzymes -with the consequent deformation of catalytic sites- and its biocatalytic activity.
Concerning temperature, AAM showed higher enzymatic activity under thermal conditions above 70 °C, possibly linked to its thermal resistance. AMG presented high enzymatic activity in the range from 60-70 °C with a maximum at 65 °C (Figure 1), bacterial PUL showed similar behavior, while plant BAM presented higher enzymatic activities between 50 and 60 °C with a maximum at 55 °C. Under thermal conditions above 80 °C, a decrease in enzymatic activity is observed (except for AAM); it could be due to thermal stress promoted during heating that leads to molecular disorder and subsequent denaturation of the polypeptide structure (Sagu et al. 2015). The molecular stability of bacterial AAM could be due to genetic adaptability, intrinsic to the conditions of the bacterial habitat where it performs its metabolic activities (Liu et al. 2017). In contrast, the reduction of enzyme activity at temperatures below 40 °C is probably due to insufficient kinetic energy stimulating collisions or ionic interactions between substrate molecules and the enzyme active site (Fitter et al. 2001). Results show that pH and temperature can increase or decrease biocatalytic activities related to the effect they promote on the affinity of the catalytic site to the substrate.
Analysis of enzyme kinetics on native starch
The biocatalytic activity presented a gradual increase in glucose release with increasing substrate concentration, governed by a first-order exponential behavior, with subsequent inhibition at substrate concentrations above 70 mM. Kinetic parameters such as vmax and km were estimated from the Lineweaver-Burk method (Figure 2A, Table 2) due to a better regression fit (r2>0.90) compared to the Langmuir method. The results revealed that AAM and AMG presented lower km values with respect to BAM and PUL, thus showing a higher affinity of the catalytic sites of AAM and AMG on native cassava starch granules. This result is consistent with the morphological changes evidenced on the granular surface using SEM. It justifies the higher values in the releasing rate of reducing sugars over time (Figure 2B). The low catalytic activity of BAM is possibly associated with low adsorption capacity, characteristic of enzymatic polypeptides extracted from plant sources, which slow down catalytic activity processes (Lahmar et al. 2018). In addition, Zareian et al. (2010) report a decrease in enzymatic activity in bacterial pullulanase using soluble starch compared to pullulan; it is associated with the adsorption capacity of the enzyme to the substrate and the molecular size of the polymeric substance. Nevertheless, the estimated values for vmax and km are close to those reported in other studies using bacterial AAM (Torabizadeh et al. 2014), fungal AMG (Gupta et al. 2015), plant BAM (Vajravijayan et al. 2018), and bacterial PUL (Li et al. 2018; Zeng et al. 2019) biocatalysts in the presence of soluble starch as substrate. However, the values differ significantly from the kinetic parameters reported by other authors, who claim that such discrepancies depend on the type of substrate, the source, and the type of enzymatic extraction, as well as its purification process (Gupta et al. 2015; Chen et al. 2015; Liu et al. 2017; Wang et al. 2019).
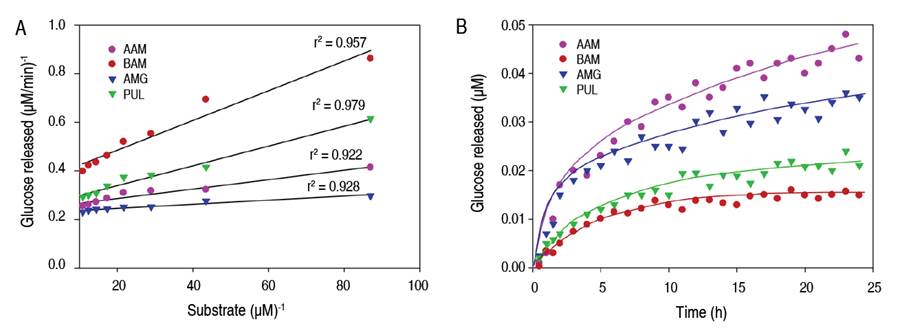
Figure 2 The behavior of enzyme kinetics on native cassava starch granules. Lineweaver-Burk linearization method (A), kinetics as a function of hydrolysis time (B). AAM: α-amylase; BAM: β-amylase; AMG: amyloglucosidase; PUL: pullulanase.
Figure 2B details the enzymatic hydrolysis behavior of native cassava starch, depending on the type of enzyme and based on the release of reducing sugars. The type of hydrolysis was biphasic, with a high rate of conversion in the first 5-6 h, followed by a progressive decrease in the degradation rate up to 24 h. The kinetics of the degradation rate were found to be similar to those of the enzyme. Similar depolymerization kinetics have been found in hydrolysis with α-amylase on waxy maize starch (Lin et al. 2016), amyloglucosidase on barley granules (Li et al. 2004), and β-amylase on soluble maize starch particles (Miao et al. 2015). This biphasic behavior is probably due to the type of substrate available -polymeric chains- in different areas of the granule, depending on their molecular structure and granular packing (Zhang et al. 2013). Thus, they explain that, initially, the enzymatic attack occurs on the linear, long, and unstable chains that make up the amorphous zone of the granule at a high conversion rate, followed by depolymerization on the short and molecularly stable polymeric chains that make up the crystalline region at a lower hydrolysis rate (Li et al. 2004). However, a decrease in substrate concentration and an occurrence of product inhibition due to the formation of oligosaccharides may also explain a lower digestion rate observed at longer times, according to the results found in the digestion of rice starch using different amylolytic enzymes evaluated by NMR spectroscopy (Dona et al. 2010). Hydrolysis with AAM and AMG is characterized by a high rate of conversion of starch into reducing sugars compared to the enzymatic treatments of BAM and PUL, this behavior agrees with the enzyme activity values and the affinity of the enzymes to the substrate analyzed by the Michaelis-Menten constant (Table 2). Also, enzymatic hydrolysis involves a polypeptide material in solution acting on a solid starch substrate. Therefore, the surface area accessible to the enzyme and the adsorption efficiency of the enzyme to the surface are critical kinetic parameters (Yang et al. 2019). They could explain the poor enzymatic activity of BAM on native cassava starch (Kim et al. 2008).
The behavior of the enzyme kinetics was adjusted to two-parameter nonlinear models, which presented a good fit with a coefficient of determination r2>0.93 and (P<0.05) and showed their suitability to describe and study polymeric degradation on native cassava starch (Table 3). In the hyperbolic model, AAM and AMG enzymes presented higher values in the maximum asymptote "A" and a longer time to reach 50% of maximum hydrolysis, indicating higher enzymatic activity and higher depolymerization of starch granules. In the enzymatic action of BAM and PUL, the values of the maximum asymptote "A" were lower, as well as the time "t", indicating a higher molecular resistance of cassava starch granules to this type of enzyme. This model has also been suitable for studying the degree of resistance of waxy maize starches to enzymatic digestion with amylases (Lin et al. 2016). In relation to the exponential model, the hydrolysis coefficients "a" were different for each enzyme, indicating a preferential and distinctive action on starch chains; these results are consistent with the behavior of the maximum asymptote values "A" and km. Lower values in the hydrolysis coefficient for BAM and PUL indicate a lower enzyme activity and a higher degree of molecular resistance of the starch granules. In addition, higher values in the hydrolysis constant "k" are consistent with the results of vmax estimated by the Michaelis-Menten model. The first-order exponential model was also used to evaluate biocatalysis processes on potato granules (Zhang et al. 2013), wheat, and waxy maize (Lin et al. 2016). In this study, the values of the maximum asymptote "A", the biocatalysis coefficient "a", and the constant km -which are characteristic parameters for each enzyme- presented a positive correlation with the decrease of amylose content after an enzymatic attack using amylolytic enzymes (AAM, BAM, and AMG) related to the preferential cleavage action on α-(1,4) glycosidic bonds.
Analysis of the enzymatic attack on the amorphous zones
Figure 3 shows the microphotographs of native and modified cassava starches. Native cassava starches (ANY by its Spanish acronym) exhibit smooth-surfaced spherical/oval geometric shapes with truncated ends associated with the grating process during extraction (Salcedo-Mendoza et al. 2018). As the granules undergo enzymatic treatment, significant changes in microstructure can be observed; the AAM starches presented significant morphological changes associated with the action of α-amylase that caused the appearance of pores, cracks, and exo-erosions on the granular surface. In addition, microphotographs of AAM starches evidenced the presence of fragmented granules, overlapping layers resembling starch growth rings can be identified inside them (Figure 3; AAM-B). The amylolytic erosion of starch granules occurs in two ways: one is radial, from the surface towards the center of the granule, and another is superficial and acts peripherally (Das and Kayastha 2019). Consequently, it could be assumed that the degradation mechanism of α-amylase on cassava granules starts with diffusion and adsorption processes, followed by a surface erosion phenomenon on overlapping layers, harshly attacking both weak and unstable zones (amorphous lamellae) composed of linear amylose chains, and slowing its enzymatic activity on ordered amylose regions (crystalline lamellae).
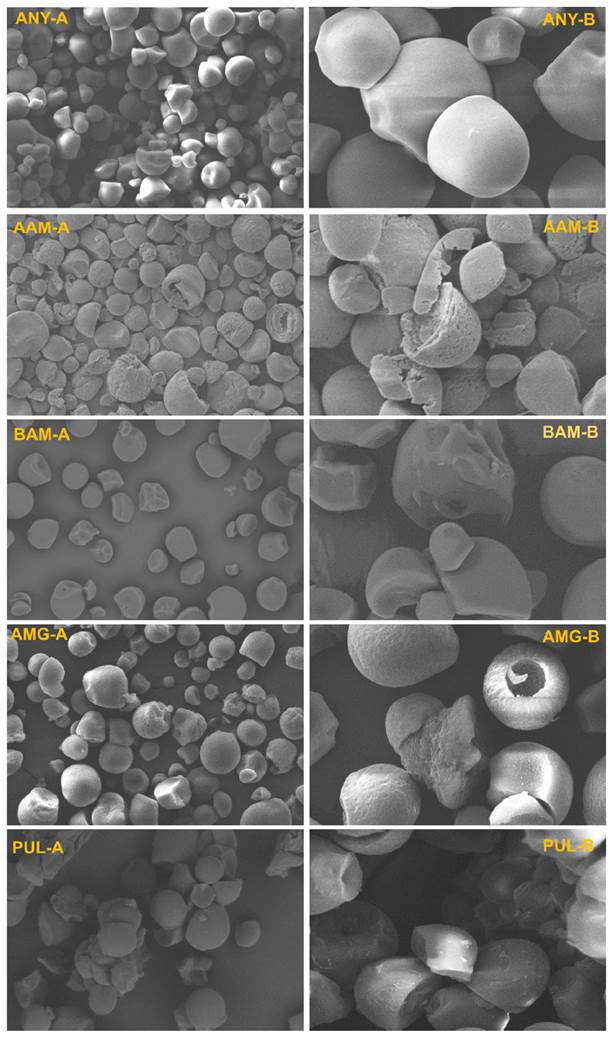
Figure 3 Morphological changes in the amorphous zones of starch after enzymatic hydrolysis. A) Magnification at 1,000X, B) Magnification at 3,000X. AAM: α-amylase; BAM: β-amylase; AMG: amyloglucosidase; PUL: pullulanase.
Similar morphological changes were observed in AMG starches, with a less severe effect on the exo-erosion phenomenon compared to AAM but causing a partial or total loss of the polymeric structure internally. This may imply that the action of amyloglucosidase shows a greater endogenous action on the amorphous and unstable zones, which is more noticeable in irregular granules that present damage associated with the extraction process and lead to obtaining slightly eroded fragmented granules with a hollow appearance (Figure 3, AMG-B). This result is congruent with Figueroa-Flórez et al. (2023), who argue that amyloglucosidase digestion on cassava starch was faster in the weakly packed inner region than in the densely packed peripheral zone, thus leaving granules with an empty/hollow structure. Meanwhile, BAM and PUL starches were characterized by the presence of slightly eroded and mostly fragmented granules, with a less severe effect in BAM starches; it could be associated with a lower affinity of the β-amylase catalytic sites for the polymeric chains of native cassava starch. The action of β-amylase and pullulanase on starch granules involves an exogenous action, whose hydrolytic processes start at the surface level in the amorphous zones and affect the amylose content with a subsequent stage that can lead to the fragmentation of granules into particles with noticeable morphological changes (Figure 3, BAM-B; PUL-B). Kim et al. (2008) hypothesized that enzyme-induced erosion of amorphous regions in starch granules leads to their fragmentation. Similar results have been reported on starches hydrolyzed from potatoes with plant β-amylase (Das and Kayastha 2019) and fungal pullulanase (Li et al. 2017), highlighting the presence of cracks and eroded areas on the surface after enzymatic action.
Moreover, the presence of undamaged granules is observed together with hydrolyzed granules as a result of non-homogeneous digestion. In this regard, Almeida et al. (2019) state that irregular granules with truncated areas are presumably more susceptible to enzymatic hydrolysis and, therefore, would show greater morphological changes with respect to whole granules of defined geometry. Likewise, in cereal starches hydrolyzed using various enzymes, the production of granules with porous structure stands out (Vajravijayan et al. 2018; Keeratiburana et al. 2020; Zhang et al. 2021; Davoudi et al. 2022; Semwal and Meera 2023); these results differ from those found in the present study. Cereal starches present numerous pores on the surface, which widen and increase the access of hydrolyzing enzymes to the interior of the granule (Blazek and Gilbert 2010; Zhang et al. 2021). However, microscopic analysis under different magnification foci reveals that ANY starches did not present surface holes or pores, which could restrict the access of amylolytic polypeptides and suggest that exogenous action governs the biocatalytic processes at the granular periphery, thus limiting the endo-erosion phenomenon.
Enzymatic action on cassava starch granules produced significant changes in amylose content (Table 4). The decrease in amylose content in AAM, BAM, and AMG treatments can be associated with depolymerization of the polymeric chains due to the cleavage of α-(1,4) and glycosidic bonds, which subsequently induced variations in both morphological characteristics and semi-crystalline behavior evaluated from X-ray diffractogram (XRD) and infrared spectra analysis by FTIR. Native cassava starch exhibited a typical type-A diffraction pattern, with close peaks at Bragg angles (2θ) at 15.1, 17.2, 18.0, and 22.9° (Figure 4). Type-A starches are characterized by arrangements of the amylopectin double helices as a closed monoclinic structure with low interhelical water content; this behavior is characteristic of tuber starches with low amylose content (<20% w/w) (Zhou et al. 2021; Salcedo-Mendoza et al. 2018). Hydrolysis with α- and β-amylase did not change the type-A diffraction pattern, which could indicate that hydrolysis occurred mainly in the amorphous region; results were congruent with SEM analyses. Meanwhile, the attack with AMG and PUL caused a significant decrease in diffraction intensity in the peaks at angles 17.2 and 18.0°, with partial loss of the peak at angle 18.0°. Studies have shown that peaks at angles (2θ) at 17.2 and 18.0° are characteristic of the amylopectin double helix arrangement (Wang et al. 2019). This may indicate that hydrolysis with AMG and PUL occurred mainly in the amorphous zone by surface action at the granular periphery, followed by degradation in the crystalline zones that affected morphological changes; for instance, the appearance of eroded granules of hollow structure using amyloglucosidase and eroded-fragmented granules after debranching action with pullulanase (Figure 3).
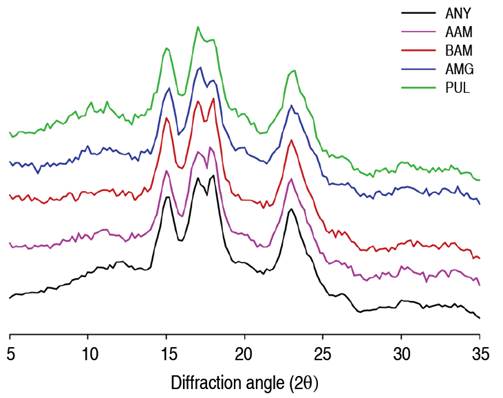
Figure 4 X-ray diffraction patterns of native and modified cassava starches. AAM: α-amylase; BAM: β-amylase; AMG: amyloglucosidase; PUL: pullulanase.
Despite there was no change in A-type patterns after AAM and BAM attack, it is presumed that hydrolysis did not affect the long-range crystalline structure but did affect crystal melting, possibly because water gained greater accessibility in the crystalline regions after the amorphous regions were partially degraded (Gui et al. 2021; Li et al. 2023). This could explain the variation in the crystallinity degree (CD) in AAM and BAM starches relative to the native counterpart (ANY) (Table 4). The CD1 was estimated by the deconvolution method under the definition of amorphous and crystalline zones in the X-ray diffractogram. A second method was proposed to estimate CD2 = [(Imax - Iam) / Imax] from the intensity of the highest crystalline peak, as a quick comparison between treatments due to the partial loss of the peak at the Bragg angle (2θ) at 18.0°. In the performed calculations, Imax corresponds to the intensity of the maximum peak in the diffractogram, and Iam corresponds to the highest relative intensity between the zones with the highest contribution by the amorphous part (19~22°) (Foresti et al. 2014).
Results showed that the CD variations by such techniques were low, although, for the maximum height method, the CD1 values in AAM and BAM starches increased concerning the ANY sample. This could corroborate the preferential action of amylases on the amorphous zones in native cassava starch. In turn, the decrease of CD2 in AMG and PUL starches presupposes a depolymerization in both amorphous and crystalline zones, thus causing a greater molecular disorganization in the granule, which is reflected in the morphological changes evaluated by SEM.
FTIR spectra in the mid-infrared region of native and enzymatically modified starches are presented in Figure 5. This method has been used to evaluate changes in starch structural organization at the short-range order molecular level (Gao et al. 2023). All the evaluated starches presented peaks at the 3,350 and 1,620 cm-1 bands designated to the stretching and angular deformation of O-H groups in tightly bound glucose units and water molecules, their width is attributed to the formation of inter- and intramolecular hydrogen bonds (Miao et al. 2015). The band at 2,940 cm-1 is related to the stretching of C-H groups in glucose units; consequently, its intensity varies with the ratio of amylose/amylopectin polymeric components (Almeida et al. 2019). The shifts and changes in band frequencies at 3,350, 2,940, and 1,620 cm-1 in the hydrolyzed cassava starches with respect to the native counterpart allow us to suggest a possible molecular reorganization in the amorphous and crystalline lamellae after enzymatic treatments. In agreement with Ma et al. (2019), a helical realignment within the crystalline lamellae in modified starches is suggested due to shifts in the bands of C-H and O-H functional groups, which are structurally associated with glycosidic bonds and water strongly bound to the starch structure.
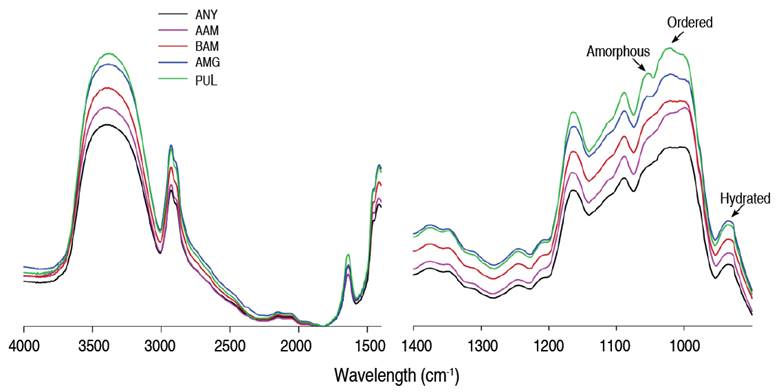
Figure 5 FTIR spectra of native and hydrolyzed cassava starches. AAM: α-amylase; BAM: β-amylase; AMG: amyloglucosidase; PUL: pullulanase.
The region between 1,200 and 900 cm-1 represents the fingerprint of starch, in which a crystalline region is characterized by absorption peaks at 1,047 and 995 cm-1, while the absorption peak at 1,022 cm-1 is linked to the amorphous region (Davoudi et al. 2022; Gao et al. 2023). In this region, the bands at 1,080 and 1,150 cm-1 have been assigned to the bending and stretching vibrations of the C-O ring of anhydroglucose molecules (Wang et al. 2019). In turn, the ratio between bands at 1,047/1,022 cm-1 were used to quantify the degree of molecular order (MO) in starch granules (Table 4). The MO values presented a decrease in AAM and BAM starches with respect to native cassava starch (ANY), but significantly increased in AMG and PUL starches. These results suggest irreversible changes in the semicrystalline order of granules after polymeric degradation. An increase in MO values is likely correlated to helical disruption within the crystalline lamellae (Ma et al. 2019), which may lead to disorganization at the molecular level, as evidenced in AMG and PUL starches where changes in crystalline peaks and CD1 were made based on X-ray diffraction spectra. From the amorphous and crystalline contributions defined by the ratio between the absorption peaks 1,047/1,022 cm-1, the maximum height method was applied assuming a baseline at the 1,065 cm-1 band to propose an alternative method to estimate and evaluate the degree of crystallinity by FTIR. The results show that the CD3 evaluated by FTIR were lower than those estimated by X-ray diffraction with differences of less than 15% (Table 4). Likewise, the results reveal that the CD behavior in the different enzymatic treatments concerning the native counterpart maintains a similar trend independent of the implemented method. Therefore, it can be assumed that the maximum height method applied to FTIR spectra is a good approach to evaluate the semicrystalline order of cassava starch granules during enzymatic modification processes.
Additionally, the band in the peak at 995 cm-1 has been assigned to the skeletal mode vibrations of the α-(1,4) (C-O-C) glycosidic bond, while the sharp peak at 1,022 cm-1 has been correlated to the stretching of C-O and C-O-C groups in α-(1,6) glycosidic bonds (Das and Kayastha 2019). The ratio between the above bands was used as an approach to evaluate the differential enzymatic action of enzymes on glycosidic bonds (OM2) (Table 4). OM2 values decreased after amylolytic processes with α- and β-amylase, indicating a possible higher activity on α-(1,4) glycosidic bonds. Also, a slight decrease in OM2 value in AMG starches suggests a presumed hydrolytic action on different glycosidic bonds in a random manner. However, Keeratiburana et al. (2020) state that amyloglucosidase is an enzyme with preferential action on linear chains, followed by action on branched sites. In contrast, a preferential action of pullulanase on α-(1,6)-glycosidic bonds can presumably be assigned due to increased OM2 values. Therefore, the behavior of OM2 values suggests that hydrolytic processes attacked linear and branched ones in native cassava starch, causing changes at the morphological and structural level, which have been previously verified through SEM and XRD analysis.
CONCLUSION
The enzymatic activity of β-amylase and pullulanase on native cassava starch granules was lower than that presented by the enzymatic polypeptides of α-amylase and amyloglucosidase, which had a higher rate of conversion to glucose, linked to a better biochemical affinity between the active site of the enzyme and the substrate. The morphological and structural results revealed that the hydrolysis was performed in two stages with different conversion rates depending on the polymeric packing and the crystallinity levels inside the granule. The correlation of structural analyses performed by XRD and FTIR suggest that α- and β-amylase present a preferential action in the amorphous zones by selectively attacking internal and external α-(1,4)-glycosidic bonds, respectively. Additionally, they lead to the formation of granules with an eroded or porous surface and a crystalline structure of higher molecular order. On the contrary, from a semicrystalline approach, the hydrolytic action of amyloglucosidase on polymeric chains seems to occur randomly, with a higher activity on truncated or previously eroded zones leading to fragmented granules of hollow structure. In turn, pullulanase presented a preferential action on the branched ends of amylopectin, decreasing the degree of crystallinity in eroded and highly fragmented granules.