INTRODUCTION
The bacterial resistance to antibiotics is a public health problem because of the high rates of mutations, selection of multi-resistant strains, and various mechanisms of adaptation that these organisms have as a defense against antimicrobial compounds (Benavides-Plascencia, Aldama-Ojeda, and Vázquez, 2005; Cavalieri et al, 2005; Fernández, López, Ponce and Machado, 2003). In recent years, large quantities of drugs have been produced, and the indiscriminate use of these contributes to antibacterial resistance (Cohen, 1992; World Health Organization, 2015, 2020). For this reason, the World Health Organization developed an action plan to combat antibiotic resistance by supporting new alternative treatments such as natural medicine (World Health Organization, 2015, 2020). Thus, institutions and researchers are oriented to find new sources of active compounds (Fernández et al, 2003). The antibacterial property is attributed to synthetic or natural compounds, products of the metabolism of plants and fungi (Nascimento, Locatelli, Freitas, and Silva, 2000; Singdevsachan, Patra, Tayung, and Thatoi, 2017).
Among the diversity of living organisms, fungi are an essential source of bioactive compounds with antibacterial characteristics (Singdevsachan et al, 2017). In fact, the extraction and analysis of compounds present in these organisms is currently essential (Castillo-Machalskis, D’Armas, Malaver, and Núñez, 2007). Macro fungi extracts, obtained through grinding, maceration, infusion, etc., possess a wide range of uses in traditional medicine (Milenge-Kamalebo et al, 2018; Ruan-Soto, 2017); they are described as having anti-tumor, anti-cancer, anti-fungal, anti-viral, anti-bacterial, immunomodulating, and cytostatic properties (Brizuela, García, Pérez, and Mansur, 1998; Sorimachi and Koge, 2008; Ureña, 2002; Wang, Wei, and Chou, 2008).
Some of the studied macro fungi with active ingredients are of the genus Agaricus. Natural extracts of species belonging to this genus are used as alternative medications and nutritional supplements (Wang et al, 2008). Among its extracts metabolites such as diazonium salt (Agaritin) and eugenol, which have inhibitory and bacteriostatic properties in the growth of fungi and bacteria (Gram-positive and gram-negative), were identified and analyzed through susceptibility tests (Dornberger, Lich, and Zureck, 1989; Schulzová et al, 2009; Soković and Van Griensven, 2006; Sun et al, 2014). In addition, it was determined that A. placomyces Peck 1878, A. bitorquis (Quél.) Sacc 1887, A. bisporus (J.E. Lange) Imbach 1946, A. brasiliensis Fr 1830, and A. blazei Murrill 1945 have antibacterial effects on Gram-negative and Gram-positive strains (Bernardshaw, Johnson, and Hetland, 2005; Jagadish, Venkata, Shenbhagaraman, and Kaviyarasan, 2009; Mazzutti et al, 2012). Meanwhile, A. essettei Bon 1983 and A. silvicola, updated in indexfungorum as A. silvicolae-similis Bohus and Locsmándi 1994, only have activity on Gram-positive strains (Barros, Cruz, Baptista, Estevinho, and Ferreira, 2008; Öztürk et al, 2011).
In Ecuador, the use of fungi in traditional medicine has been recorded, and this ethnomycological use is only surpassed by its employment in food (Gamboa-Trujillo et al, 2014; Singdevsachan et al, 2017). Agaricus pampeanus Speg 1880 known as “Kallamba de finados” is used in local communities; however, there are no recorded studies about its medicinal properties (Gamboa-Trujillo et al, 2014). In this context, the present study seeks to evaluate the antibacterial activity of A. pampeanus on ATCC strains of Enterococcus faecalis, Staphylococcus aureus, Escherichia coli and Pseudomonas aeruginosa.
MATERIALS AND METHODS
Study design
The present study is framed in the lines of a cross-sectional observational-descriptive research according to the study time.
Collection of fungal material
Approximately 200 g of fresh fruiting bodies of A. pampeanus were obtained at the César Chiriboga market located in Sangolquí, Cantón Rumiñahui. The samples were identified in the Laboratory of Applied Mycology of the Faculty of Chemical Engineering of the Central University of Ecuador.
Extract preparation
The impurities in the fruiting bodies were removed by washing them with distilled water. The fruiting bodies were dried with the help of a stove and pulverized in an electric mill. A total of 20 g of sample were obtained. The sample was placed in a paper filter cartridge and then in the body of the Soxhlet extractor. Then it was hydrated with 50 mL of 96 % ethanol and 100 mL of ethanol were placed in a round flask, which was boiled at a constant temperature of 78 °C for approximately one hour, by refluxing the extraction system and accomplishing three siphons (Saldaña-Sunción and García-Medina, 2016). Once the mother extract was obtained, it was stored in an amber bottle at -4 °C for its preservation.
Extract efficiency
To calculate the extract yield, the initial weight of the sample was taken versus the final weight (Martinez, Valencia, Jimenez, Mesa, and Galeano, 2003):
To determine the grams dissolved in the final liquid extract, the initial and final volume of the alcohol used, as well as the volume of the sample under extraction were considered.
Preparation of concentrations
From the mother extract, named ethanolic extract of A. pampeanus (APE), dilutions with distilled water were prepared. The initial extract, which was called APE1, was kept at a concentration of 100% with 50.32 mg/mL. The following extract, designated APE2, was diluted 80% with 40.24 mg/mL. The third extract, known as APE3, was prepared at a concentration of 60% with 30.16 mg/mL. Finally, the APE4 extract was at 40% of concentration with 20.12 mg/mL.
Activation of ATCC strains
The ATCC bacterial strains and culture medium were purchased from authorized commercial stores. The strains in Kwik-Stik presentation were activated in Trypticase Soja Agar (TSA). Subsequently, the young strains were inoculated into Brain Heart Infusion (BHI) liquid medium from which the bacterial suspension of 0.5 McFarland (1.5 X 10-8 CFU/mL) was done for the antibacterial analysis.
Analysis of antibacterial activity by Kirby-Bauer technique
Mueller Hinton Agar (MHA) solid culture medium was used for S. aureus ATCC® 25923™, E. coli ATCC® 25922™ and P. aeruginosa ATCC® 9027™, and Mueller Hinton blood agar was used for E. faecalis ATCC® 29212™. The four bacterial strains were inoculated in 15 cm diameter Petri dishes, four repetitions of each inoculation were performed and each test was triplicated. Then, disks loaded with 15 µL of each concentration of the ethanol extract were properly distributed in each Petri dish. In addition, as a positive control, 120 µg gentamicin disks were applied for E. faecalis ATCC® 29212™ and 10 µg gentamicin for S. aureus ATCC® 25923™, E. coli ATCC® 25922™ and P. aeruginosa ATCC® 9027™, while as a negative control disks with ethyl alcohol were applied. The incubation process was applied at 37 °C in a range of 18 to 24 hours (Cavalieri et al, 2005).
Inhibition zone measurement
Once the incubation time elapsed, the inhibition halos were measured in millimeters with the help of a calibrator and dark cardboard (Cavalieri et al, 2005).
Interpretation of inhibition zones
Reference points for each antibiotic used as a control were established taking into account the criteria established by the National Committee for Clinical Laboratory Standards (NCCLS), with this it was determined whether the bacteria in the study were sensitive, sensitive intermediate or resistant (Cavalieri et al, 2005; Clinical and Laboratory Standards Institute-CLSI, 2014). For the analysis of results, the arithmetic mean, standard deviation and variance of the set of repetitions applied for each of the extracts against each bacterium were calculated. With this data, tables and graphs were made.
RESULTS
After doing the quantitative and qualitative analysis of the ethanolic extract of A. pampeanus by gas chromatography coupled to mass spectrophotometry, six compounds were obtained, characterized and described (Table 1). Based on this, it was determined that the yield of the ethanolic extract of the fruiting bodies of A. pampeanus was 25.67%, equivalent to 5.536 g diluted in 110 mL of the ethanolic extract.
Table 1 Description of the compounds present in the ethanol extract of A. pampeanus
Compound name | Formula | Synonyms | Total (%) |
---|---|---|---|
Pyrimidine-2,4 (1H, 3H) -dione, 5-amino-6-nitroso | C4H4N4O3 | Hydrazoic acid | 37.526 |
Azoimide | |||
Diazoimide | |||
Acetic acid | C2H4O2 | Ethanoic acid | 13.448 |
p-Menth-8-en-3-ol | C10H18O | Cyclohexanol | 15.918 |
Eugenol | C10H12O2 | Phenol, 2-methoxy-4- (2-propenyl) - | 15.244 |
Eugenic acid | |||
Acid (1,1'-bicyclopropyl)-2-octanoic 2'-Hexyl, ester methyl. | C21H38O2 | Isopropyl Linoleate | 11.104 |
Linoleic acid | |||
Ethyl (9Z, 11E)-octadeca-9,11-dienoate | C20H36O2 | Ethyl linoleate | 6.760 |
Antibacterial activity of ethanolic extract of A. pampeanus by Kirby-Bauer technique
By exposing the APE against the target bacterial strains, it was possible to identify that it is more effective on Gram-positive strains of S. aureus and E. faecalis, on Gram-negative bacteria, the activity was verified only against P. aeruginosa, excluding E. coli. The latter was categorized as resistant since its growth was normal around the APE discs, however, the positive control showed sensitivity with an average halo of 22 mm in diameter, demonstrating the feasibility of the methodological application (Figure 1). Furthermore, the activity observed against P. aeruginosa is markedly decreased compared to Gram-positive bacteria.
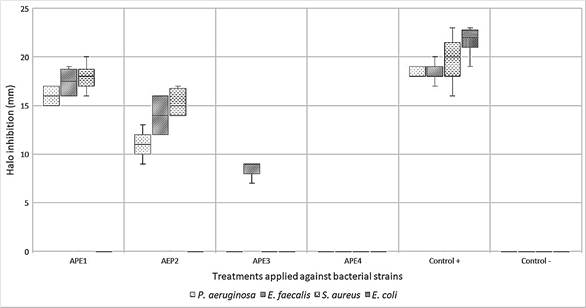
Figure 1 Antibacterial activity of the ethanolic extract of A. pampeanus in concentrations of APE1 (100%), APE2 (80%), APE3 (60%) and APE4 (40%) on the four bacterial strains. A) E. faecalis. B) S. aureus. C) P. aeruginosa. D) E. coli.
As a result, it was determined that APE1 was the most effective with a concentration of 50.32 mg/mL (100%) by showing greater diameter in the inhibition halos against S. aureus (18 mm), E. faecalis (17 mm), and P. aeruginosa (16 mm), followed by APE2 with a concentration of 40.24 mg/mL (80%) with halos of 15 mm, 14 mm and 11 mm respectively. Regarding APE3, with 30.16 mg/mL (60%), it was the only one that had an inhibitory effect against E. faecalis with an average halo of 8 mm. Finally, APE4 with 20.12 mg/mL (40%) does not have antibacterial activity in any case (Figure 2). Furthermore, it should be noted that the inhibition halos exhibited by the different concentrations of APE in the strains under study did not exceed those generated by the positive controls.
For the classification of antibacterial activity by the Kirby-Bauer method, the previously mentioned halos’ average was taken into account with the following criteria: R) resistant, I) intermediate, S) sensitive. The limits of the positive control Gentamicin (10 µg) ranged from < 12 (resistant), 13-14 (intermediate), > 15 (sensitive) and Gentamicin (120 µg), < 6 (resistant), 7-9 (intermediate) and > 10 (sensitive) (Table 2), in which APE4 was excluded due to not having any activity.
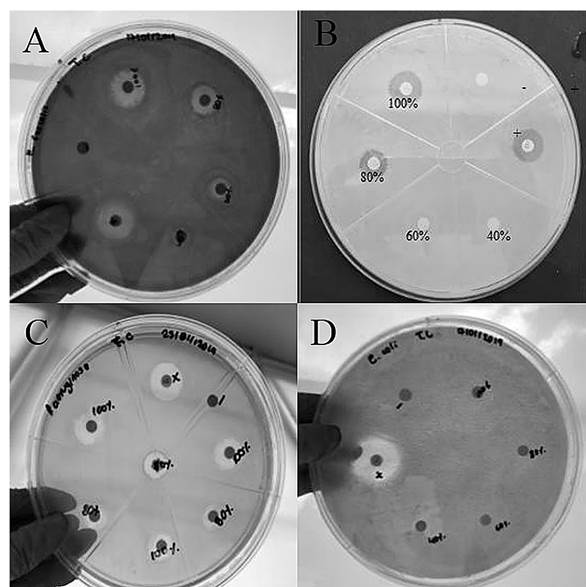
Figure 2 Mean of halos inhibition by bacterial strain against the treatments of ethanolic extract of A. pampeanus (APE1, APE2, APE3, APE4, Positive Control +, and Negative Control -).
DISCUSSION
The antibacterial activity of A. pampeanus was demonstrated from its ethanolic extract. This activity occurs in other species of the genus Agaricus throughout the world and provides an additional value other than the nutritional one (Barros et al, 2008; Gamboa-Trujillo et al, 2014; Öztürk et al, 2011; Singdevsachan et al, 2017). The antibacterial activity on strains of E. faecalis, S. aureus and P. aeruginosa is important since there are species of the same genus to which a similar inhibitory effect against other strains has been attributed; this has been previously demonstrated when extracts of A. bisporus are used against Bacillus subtilis, A. silvicolae-similis against B. cereus, B. subtilis and S. aureus (Barros et al, 2008). Likewise, the activity of A. bisporus against S. aureus, B. subtilis, K. pneumoniae and P. aeruginosa was verified (Jagadish et al, 2009). Furthermore, Mazzutti et al (2012) established the effectiveness of A. brasiliensis against S. aureus and B. subtilis. While Bernardshaw et al (2005) determined that extracts of A. blazei have the possibility of being used against Streptococcus pneumoniae in certain treatments. It should be noted that the effectiveness against Gram-negative bacteria has a lower spectrum when identifying only the inhibitory effect on E. coli using extracts of A. placomyces (Pérez-Silva, 1959). In turn, the activity of the genus against Gram-positive bacteria is verified better when exposing extracts of macro fungi such as A. bitorquis and A. essettei against strains of Micrococcus luteus, M. flavus, B. subtilis and B. cereus (Öztürk et al, 2011). Thus, the activity resulting from A. pampeanus on Gram-positive and Gram-negative bacteria is corroborated.
The antimicrobial activity presented by an extract is due to the initial uptake of the metabolites generated by the organisms studied; therefore, it is important to use a suitable solvent that allows these metabolites to be extracted. Mazzutti et al (2012), determines that the use of different solvents to obtain extracts of the same fungus, exhibits opposite results against the same bacterial strains, so when using A. brasiliensis against S. aureus, B. cereus, E. coli and P. aeruginosa different results were obtained. In the same way, the use of different solvents in extracts of A. bisporus differ in effectiveness compared to S. aureus (Barros et al, 2008; Jagadish et al, 2009). Therefore, the viability of extracts of A. pampeanus can be verified by using a solvent such as ethanol and obtain optimal results against three of the four bacterial strains evaluated.
Through the disk diffusion test applied in this study, the effectiveness of the extract was verified, it was observed that S. aureus was inhibited to a greater extent by APE1 (18 mm), characterized as sensitive. Likewise, Barros et al (2008) highlight positive results for methanolic extracts of highlight the positive results for methanolic extracts of A. silvicolae-similis, which shows effectiveness with halos greater than 9 mm, and the boiled ethanol extracts of A. bisporus have an average halo of 18 mm (Jagadish et al, 2009). While methanolic extracts of A. bitorquis and A. essettei presented halos of 12 and 11 mm respectively (Öztürk et al, 2011). The inhibition halos of the extract against E. faecalis (17 mm) and P. aeruginosa (16 mm) demonstrated the sensitivity that APE1 possesses against them, and are measures that approximate methanol extracts of A. bisporus, however, some authors differ from the results for the use of different solvents (Jagadish et al, 2009; Öztürk et al, 2011). Jagadish et al (2009) and Öztürk et al (2011), also characterized E. coli as resistant from extracts from species such as A. bisporus, A. bitorquis and A. essettei. However, Pérez-Silva (1959), in his study demonstrates that the crude extract of A. placomyces is able to inhibit the growth of E. coli with halos of 35 mm in diameter, and 25 mm in purified extracts, and mentions that bioactive fungal compounds can remain trapped during filtration processes for purification.
The extracts obtained showed the presence of Eugenol and acetic acid that reported antibacterial, antifungal and bacteriostatic properties, it should be noted that acetic acid was possibly generated by the oxidation of ethanol at the time of making the extract, so the primary compound already evaluated is Eugenol (NCBI, 2020). However, Dornberger et al (1989), mentions that the antibacterial activity in the genus Agaricus is due to the presence of the bioactive compound called Agaritin. Despite this, after analysis by gas chromatography said compound was not found in the extract, we can assume that it suffered a rupture or physical change in its structure by the high temperature employed during the analysis. For this reason, A. pampeanus could be considered a new source of active principles with antimicrobial action and should be taken into account for future studies against other microorganisms. Therefore, after the analysis of fungi such as A. blazei, A. bisporus and A. silvicolae-similis some studies express that these types of bioactive compounds can be used in medicine as prophylactic and therapeutic agents or simply be supplied in the diet of a person as nutraceuticals (Barros et al, 2008; Bernardshaw et al, 2005).
Finally, through this study it was determined that the ethanol extract of A. pampeanus has antibacterial activity against Gram-positive and Gram-negative bacteria. However, the effect against Gram-negative bacteria tends to decrease as in P. aeruginosa while with E. coli it lacks activity, possibly attributed to the difference in the constitution and complexity of the cell wall and some constituents such as phenols and flavonoids that could act differently in these organisms (Cavalieri et al, 2005; Jagadish et al, 2009; Öztürk et al, 2011; Soković and Van-Griensven, 2006). Based on the data reported in previous studies, it could be considered that eugenol favors the antibacterial activity of the extract.