Introduction
Chromium (Cr) is the twenty-first most abundant element in the earth's crust, occurring naturally in environments such as geological formations, especially in ultramafic and basaltic rocks. In addition, chromium plays a key role in various industrial activities, such as leather tanning, chrome plating, metallurgy, electroplating, pigment synthesis, chemical industries, and wood preservation, among other uses (Núñez et al., 2018). However, the extensive use of chromium has generated large amounts of waste containing this element, producing contamination in various environments, including surface water, groundwater, soil and aquatic sediments (Kholisa et al., 2021).
In the environment, chromium (Cr) is mainly found in two oxidation states: Cr (III) and Cr (VI). The Cr (III) state is an essential micronutrients in plants, which is moderately soluble in groundwater (He et al., 2019) and represents the most stable configuration of the element due to its marked tendency to form hexacoordinate complexes, which are kinetically inert towards water, organic acids, sulfate, ammonia, halides and urea (Qiu et al., 2020). Cr(VI), on the other hand, poses a significantly higher risk due to its solubility, permeability and mobility. This state of chromium is highly toxic, carcinogenic and mutagenic to living organisms, even when found in minimal concentrations in water (Liu et al., 2020).
Consequently, the United States Environmental Protection Agency (USEPA) has established permissible limits for Cr (VI) in domestic water, with a value of 0.05 mg/L, and for aquatic life, with a limit of 0.01 mg/L, regulations that have also been adopted in Colombia through Resolution 2115 of 2007. The USEPA, in turn, has included chromium in the list of seventeen chemicals that pose risks to human health (Tang et al., 2021). As a result of these regulations, various physicochemical alternatives have been implemented to mitigate negative impacts in chromium-contaminated environments, such as chemical reduction, ion exchange, electrochemical treatment, and membrane separation, among others (Kholisa et al., 2021). However, most of these technologies often prove to be ineffective and expensive, especially due to the low concentrations of metals present. In addition, the use of chemical reagents leads to the generation of large amounts of hazardous sludge, which requires additional treatment (Mtimunye and Chirwa, 2014).
Among the various innovative and economically viable strategies is bioremediation, which involves the use of microorganisms or their enzymes to reduce heavy metal levels. One particularly promising approach is the use of microorganisms isolated from chromium-contaminated areas, known as bioreduction with microorganisms. This method mimics natural detoxification processes and is characterized by being minimally invasive, making it an ecologically compatible option for contaminated areas and with reduced costs (Ma et al., 2019).
To date, most bioremediation studies applied with wastewater treatment have employed single cultures that focus on one or two specific contaminants, mainly chromium (Malaviya and Singh, 2016). However, since wastewater is often complex in nature and contains a variety of metals and organic pollutants, it is evident that the implementation of systems involving several microorganisms in the form of microbial consortia is preferable for bioremediation. This is because it is not feasible to find a single microorganism capable of effectively addressing a complex mixture of diverse contaminants (Leonard and Mishra, 2022). In addition, the use of indigenous microorganisms from contaminated sites in the formation of microbial consortia could increase the effectiveness in the treatment of contaminants, as these microorganisms have adapted to the specific conditions of that environment (Kholisa et al., 2021).
Thus, the implementation of indigenous microorganisms represents a widely applicable technology for the management and reduction of pollutants (Su et al., 2022), as these microorganisms form an innate microbial consortium that inhabits soil and living surfaces, and possess the ability to carry out biodegradation, bioleaching and biocomposting processes. Therefore, their use is presented as an intrinsically attractive and efficient alternative to preserve the environment (Kholisa et al., 2021). Due to this problem, the need arises to implement an in vitro microbial bioremediation method, with consortia from wastewater containing chromium in which microorganisms such as Raoultella sp., Serratia sp., Candida tropicalis and Candida famata are implemented for the reduction of heavy metals such as Cr (VI).
Materials And Methods
Obtaining microbial isolates
The microorganisms used in the present study were previously collected by the research group on Cardiovascular and Metabolic Diseases (GECAVYME) in wastewater contaminated with chromium from a tanning industry. These microorganisms were subsequently isolated using the agar streak depletion method and preserved at a temperature of -80 °C in the microorganism collection of the University of Quindío (CMIUQ), under the supervision of the research group in Basic Sciences and Education (GICBE) (López et al., 2017).
Identification and activation of microbial isolates
The activation process was performed by combining 7 mL of Brain Heart Infusion (BHI) broth with 200 µL of sample of the isolated microorganisms. Subsequently, these were incubated at 37.5 °C for 24 hours in the case of bacteria and at 35 °C for 48 hours to promote yeast growth (Argote-Vega et al., 2017). We then proceeded with the identification stage by seeding by depletion on plates using various culture media, such as MacConkey agar, blood agar, yeast peptone dextrose (YPD) and Sabouraud with chloramphenicol (Fontalvo, 2012), incubating for 24 hours in the case of bacteria and 48 hours for yeast. Isolated colonies were obtained with macroscopic characteristics with differences in color and texture (Bou et al., 2011) and microscopically, specifically for bacteria using the Gram staining technique (Tobón and Hoyos, 2012). In addition, biochemical tests were performed for bacteria, such as the catalase test, the oxidase test (Kovacs reagent), and Indole (Silva and Aguilera, 2020). For Gram-negative bacteria, the BD BBL Crystal ID system was used (Gashaw et al., 2021).
For yeasts, microscopic identification was performed using the lactophenol blue tape technique (Silva and Aguilera, 2020) and the API 20 C AUX biochemical test system (Alfonso et al., 2010). To differentiate species of the genus Candida spp. the germ tube test was used (Yazdanpanah and Khaithir, 2013).
Synergy and/or antagonism tests
The in vitro evaluation of antagonism between microorganisms was performed by determining growth inhibition between them, applying simultaneous inhibition tests by sensi-discs (Gajic et al., 2022) and agar diffusion (Balouiri et al., 2016). Each of these tests was run in triplicate to ensure the accuracy of the results. Likewise, the concentrations required for each microorganism were standardized using spectrophotometry. Inhibitory activity was evaluated by observing the formation of inhibition halos on the culture plates.
A simultaneous inhibition test was performed based on the Kirby-Bauer susceptibility test (Gajic et al., 2022). In this procedure, each isolated microorganism was mass seeded on BHI agar medium. Then, three 5 mm diameter filter paper discs were placed on the surface of each Petri dish, which were moistened with the microorganisms used as antagonists. The plates were incubated at 37.5 °C for 24 hours. For the application of the agar diffusion method (Balouiri et al., 2016), BHI agar media were prepared by individually inoculating 1 mL of each of the study microorganisms and mass seeding. Then, 10 mL of BHI agar was added to each plate and three holes of 6 to 8 mm in diameter were aseptically punched with a sterile punch. Into these holes, 20 µL of each organism used as an antagonist was added. The plates were incubated at 37.5 °C for 24 h (Balouiri et al., 2016). In total, 24 antagonism tests were performed in triplicate, i.e. 12 tests by simultaneous inhibition and 12 tests by agar diffusion. In both tests, the antagonistic effect of the four species of microorganisms was evaluated.
Establishment of growth curves
The establishment of growth curves allowed analyzing the growth of microbial isolates in an exponential process, where each generation represented a cell division cycle, and was calculated by plotting the increase of cells in a culture per unit of time. The growth of the microbial isolates C. famata, C. tropicalis, Raoultella and Serratia sp. was analyzed in a generational time (0-6 hours) detailing their growth phases (latency, exponential, stationary and cell death). Subsequently, the consortia combinations defined as follows were chosen: C. famata-Raoultella sp., Raoultella sp.-Serratia sp., C. tropicalis-C. famata, C. famata-Serratia sp., C. tropicalis-Raoultella sp. and C. tropicalis-Serratia sp.
The growth of the consortia was measured by absorbance in triplicate, at an optical density (OD) of 600 nm on the Epoch BioTek spectrophotometer (Winooski, USA) and Gen 5.06 software (Winooski, USA) (Dogan et al., 2011). The growth curves were plotted in OriginPro 8 software (Northampton, USA) taking into account the mean absorbances of isolates and consortia as a function of time.
Microbial consortium growth with Cr(VI)
Preparation of Cr (VI) stock solution
A stock solution was prepared at a concentration of 1000 mg/L Cr (VI), using 2.829 g of potassium dichromate (K2CrO27) per liter of distilled water. The solution was sterilized and calibrated in BHI medium, using its maximum concentration for subsequent use of small aliquots with minimal dilution of the culture medium (Avendaño-Flores, 2012).
Calibration curve
To quantify the Cr (VI) reduction of the microbial isolates and consortium, a calibration curve was performed by chemically analyzing seven standard solutions (0.15, 0.3, 0.45, 0.6, 0.75, 0.9 and 1.05) with the 1,5-diphenylcarbazide method; 0.9 and 1.05) with the 1,5-diphenylcarbazide method, considering the addition of 1 mL of the stock solution, 0.5 mL of 20% sulfuric acid (H2SO4), 1 mL of 80% phosphoric acid (H3PO4) and 1 mL of 85% 1,5-diphenylcarbazide solution dissolved in acetone. The absorbance of the standard solutions was measured at the wavelength of 543 nm, with 5 cm optical pitch cells (Ceballos et al., 2017).
Adaptation of microbial consortia to Cr(VI)
Once the microorganisms were identified and their synergy evaluated, seven microbial consortia were established: Serratia sp.-C. tropicalis, Serratia sp.-C. famata, Serratia spp.-Raoultella sp., Raoultella spp.-C. famata, Raoultella sp.-C. tropicalis, C. tropicalis-C. famata, and Serratia sp.-Raoultella sp.-C. famata-C. tropicalis. Each consortium was subjected to a stepwise adaptation process, initially inoculating 9 mL of stock solution medium in culture medium with Cr (VI) at 0.05 ppm and 1 mL of the microbial consortium previously activated in BHI medium (pH 7.4), incubated in a Thermo Scientific Shaker (Waltham, USA) at 100 rpm at 37 ºC for 10 hours.
The adaptation was developed by means of a staggering of eight concentrations of Cr (VI), starting with 0.5 ppm, once the consortia grew at this concentration, it was increased to 1.56 ppm; 3.12 ppm; 6.25 ppm; 12.5 ppm; 25 ppm; 50 ppm and 100 ppm Cr (VI), during a period of 10 hours, distributed in time ranges classified from 0 to 5, where 0 represents the beginning, and the numbers 1, 2, 3, 4 and 5 equal 2, 4, 6, 8 and 10 hours of adaptation, respectively. Each adaptation in the consortia was performed in triplicate. The Cr (VI) concentration ranges were established taking into account the regulations for Cr (VI) in water for human consumption, where the maximum concentration allowed is 0.05 mg/L according to Colombian Resolution 2115 of 2007 and the World Health Organization (WHO) (World Health Organization, 2021). The optical densities of each consortium were taken under the different Cr (VI) concentrations and the growth curves were compared. The optical density was measured in a GENESYS 10UV spectrophotometer (Waltham, USA) at a wavelength of 600 nm, using chromium-free BHI as blank. The graphs were made in OriginPro 8 software (Northampton, USA).
In addition, a reciprocal regression model (Y = a + b/ X) was performed to evaluate how growth rates vary in relation to the removal of Cr (VI) concentrations. This regression analyzed the relationship between an independent variable (X), representing the growth curves of the microbial consortia, and a dependent variable (Y), reflecting Cr (VI) removal. The model is based on a reciprocal function and seeks to understand how changes in the growth curves of the consortia affect Cr (VI) removal at different concentrations (0.5 ppm; 1.56 ppm; 3.12 ppm; 6.25 ppm; 12.5 ppm; 25 ppm; 50 ppm and 100 ppm), considering that the growth curves are in the denominator of the equation. The averages of the triplicate data were calculated and the values of p < 0.05, coefficient of determination (R²), correlation coefficient and autocorrelation of residuals with a lag of 1 (lag 1), measuring the temporal relationship of the residuals in the model, were evaluated. Positive and significant values indicate a positive correlation, which could suggest a lack of consideration of time dependence. Negative and significant values suggest a negative correlation, which could indicate an overestimation of time dependence or systematic errors. Values close to zero or not significant indicate an adequate capture of the temporal structure in the data by the model. The above analyses were performed in Statgraphics Centurion XVI software (Warrenton, USA).
Evaluation of the percentage removal of Cr (VI)
Each triplicate was centrifuged at 10,000 rpm for 5 minutes, the supernatant was taken and Cr (VI) removal was determined by the colorimetric method with 1,5-diphenylcarbazide, specific for Cr (VI) (American Public Health Association et al., 2012) on Epoch BioTek spectrophotometer (Winooski, USA) and Gen5 2.06 software (Winooski, USA) measured at a D.O. of 540 nm were used to perform Cr (VI) measurements. Cr (VI) concentrations were determined from a previously prepared calibration curve (Ceballos et al., 2017). Then, reduction curves were carried out in the presence of 1,5-diphenylcarbazide, where chromium reduction was evaluated and compared. These graphical representations were generated using OriginPro 8 software (Northampton, USA).
The quantification of total chromium was carried out using the atomic absorption (AA) spectrophotometry method. This process included the preparation of chromium solutions at a concentration of 100 ppm, as well as the creation of working standard solutions with concentrations of 1, 2, 3 and 5 ppm. Next, steps were carried out involving sample digestion, filtration, dilution and reading in a spectrophotometer at a wavelength of 357.9 nm. From the data obtained, a calibration curve was constructed by correlating the absorbance values, and by extrapolating the equation obtained, the amount of total chromium present was determined. To calculate the percentage of chromium removal, the difference between the percentage Cr (VI) minus the percentage of total Cr (% Cr removal = Chromium (VI)-Total chromium) was calculated (Soto-Rueda et al., 2017).
Results
Bacterial and fungal identification
The bacterium Serratia sp. was identified with colonies of round shape, white color, elevated with entire edge, superficial and with creamy appearance; gram negative, negative catalase and indole tests; confirmed by BBL Crystal test. On the other hand, the bacterium Raoultella sp., evidenced colonies with punctiform morphology, superficial, of acuminate elevation, entire edge, yellow color and creamy appearance, gram negative, negative indole and catalase test; confirmed by BBL Crystal test.
Additionally, the yeast-like microorganisms C. famata and C. tropicalis were also identified. C. famata with slightly domed morphology of buttery consistency, absence of pseudohyphae and blastoconidia. The yeast grew on glucose, glycerol, calcium 2-keto-gluconate, xylose, adonitol, xylitol, galactose, sorbitol, glucopyranoside, N-acetyl glucosamide, maltose, sucrose, trehalose and melezitose substrates which confirmed its identification. C. tropicalis showed a slightly domed morphology with a buttery consistency; extension of a filament called pseudohypha, and formation of blastoconidia. Finally, the yeast grew after 72 hours on the biochemical substrates glucose, calcium 2-keto-gluconate, xylose, adonitol, galactose, sorbitol, methyl glucopyranoside, N-acetyl glucosamine, maltose, sucrose, trehalose and melezitose, which supported its identification.
Antagonism tests
Simultaneous inhibition and agar diffusion tests showed growth of the species with an inhibition halo of 0 mm. This demonstrated a synergistic effect between the species C. tropicalis, C. famata, Serratia sp. and Raoutella sp. indicating positive interactions (Annexes 1 and 2).
Establishment of growth curves
In the individual growth curves of the microorganisms, the highest performance was demonstrated in C. famata with an absorption of 1.238 (D.O. 600 nm) in its exponential phase in the third hour (Figure 1A), followed by the bacterium Serratia sp. with a maximum absorption in its exponential phase of 1.2035 in the fourth hour (Figure 1A). With respect to the growth curves of the mixed consortia, the maximum absorption of 1.2940 (O.D. 600 nm) was found in the C. famata-Serratia sp. consortium, showing no evidence of inhibition or competition in microbial activity during this phase (Figure 1B). In the stationary phase after the third hour in all the consortia evaluated, they remained almost unchanged with no increase in the number of cells and a reduction in metabolic activity, implying a subsequent phase of cell death.
In comparison with the individual growth of the microorganisms and of the mixed consortia, a higher growth rate was evidenced in most of the consortia (Figure 1). According to the behavior identified in the curves, synergy between the microorganisms was demonstrated because in consortium they increased their growth rate and managed to remain in stationary phase at the same time as the curves of the individual organisms.
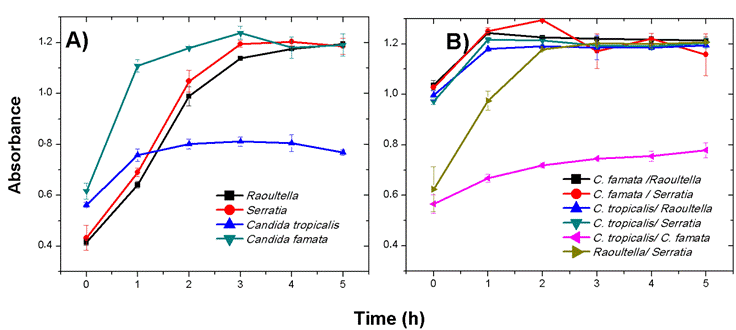
Figure 1 Growth curves at 600 nm absorbance of individual microorganisms (A): Raoultella sp., Serratia sp., C. tropicalis and C. famata and in mixed consortia (B) defined as follows: C. famata-Raoultella sp., C. famata-Serratia sp., C. tropicalis-Raoultella sp., C. tropicalis-Serratia sp., C. tropicalis-C. famata and Raoultella sp.-Serratia sp. The numbers 0, 1, 2, 3, 4 and 5 equal 0, 2, 4, 6, 8 and 10 hours of adaptation, respectively.
Adaptation and removal of consortia for Cr (VI) reduction
The calibration curve relates the Cr (VI) concentration of the seven standard solutions as a function of the absorbance measured at a wavelength of 543 nm, such measurements were performed in triplicate and averaged, considering as dependent variable the absorbance and as independent variable the Cr (VI) concentration (A = 0.0073 + 0.7005*[Cr]) obtaining an R2 of 98.67% (Figure 2) evidencing the linearity predicted by the Lambert-Beer law (Díaz et al., 2010).
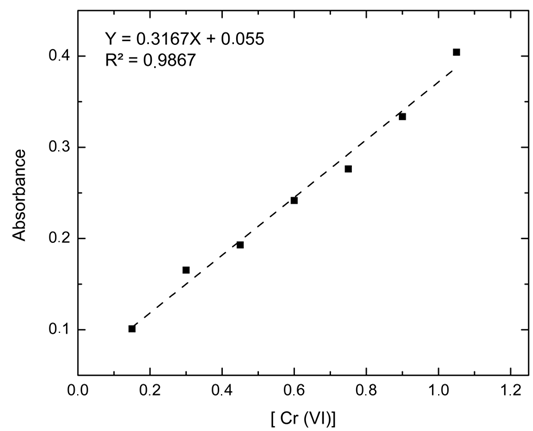
Figure 2 Calibration curve for Cr (VI) concentration mg/mL vs absorbance by colorimetric analysis of 1,5-diphenylcarbazide.
When evaluating the adaptation and removal capacity of the consortia at eight Cr (VI) concentrations, greater adaptation was identified in the Serratia spp.-C. tropicalis and Raoultella spp.-C. tropicalis consortia at times 4 and 5, which corresponded to 8 and 10 hours of exposure to Cr (VI), at all the respective concentrations (Annexes 3-6). Additionally, greater adaptation of the consortia was observed from the 6.25 ppm Cr (VI) concentration up to 100 ppm Cr (VI), given that as the Cr (VI) concentration increased, the consortia grew at higher uptake. However, they took longer to initiate their exponential phase (Figure 3).
The highest adaptation and removal in the consortia was at the highest Cr (VI) concentrations (25, 50 and 100 ppm). Specifically, the Serratia spp.-C. tropicalis consortium had the best removal and adaptation performances at five of the eight concentrations (3.12 ppm, 12.5 ppm, 25 ppm, 50 ppm and 100 ppm) (Annexes 3-6).
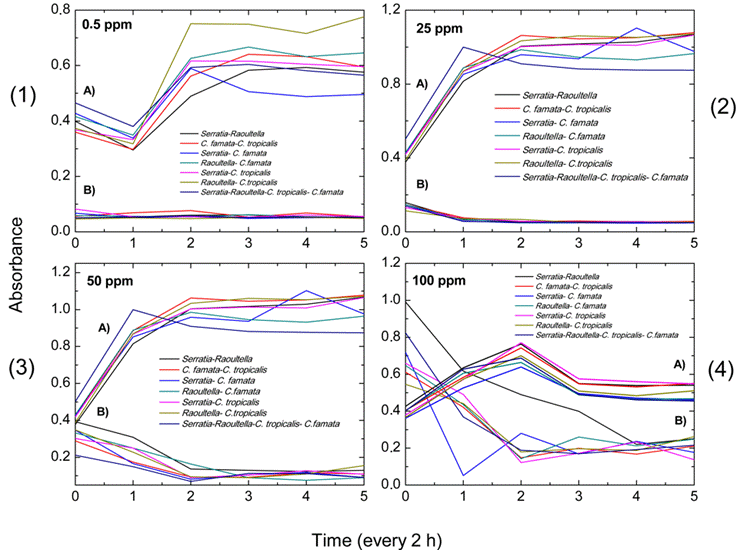
Figure 3 Absorbance values obtained for each of the established microbial consortia Serratia sp.-Raoultella sp.; C. famata- C. tropicalis; Serratia sp.- C. famata; Raoultella sp.-C. famata; Serratia- C. tropicalis; Raoultella sp.-C. tropicalis and Serratia sp.-Raoultella sp.-C. tropicalis-C. famata. A. represents the growth curves of each consortium. B. represents the adaptation to chromium (VI) in each consortium at different concentrations: 0.5 ppm (1), 25 ppm (2), 50 ppm (3) and 100 ppm (4). The numbers 0, 1, 2, 3, 4 and 5 are equivalent to 0, 2, 4, 6, 8 and 10 hours of adaptation, respectively.
With respect to the reciprocal regression model, significance was found for most of the consortia evaluated at concentrations higher than 12.5 ppm. The coefficients of determination indicated that the relationship of Cr (VI) removal at concentrations of 25 ppm versus the growth curves of the consortia C. tropicalis-Serratia sp., C. famata-Serratia sp. and C. tropicalis-C. famata, explained 97.32%, 97.47% and 98.35% of the values, respectively. The lagged residual autocorrelation (LRA) yielded negative values at Cr (VI) concentrations of 0.5 ppm, 1.56 ppm and 3.12 ppm (except for the Serratia sp.-Raoultella sp.-C. tropicalis-C. famata consortium), C. tropicalis-C. famata), 6.25 ppm, 12.5 ppm and 25 ppm (except Serratia sp.-Raoultella sp.-C. tropicalis-C. famata consortium) and 50 ppm, which indicated a negative correlation and possible overestimation in the model. However, the value close to 0 at the 100 ppm concentration in the C. tropicalis-Serratia sp. consortium (LRA= 0.27) showed an adequate capture of the temporal structure in the data by the model (Table 1).
Evaluation of the percentage removal of Cr (VI) in microbial consortia
The Cr (VI) reduction capacity was tested for each microbial consortium at a concentration ranging from 0.5 ppm to 100 ppm. The microbial consortium with the highest Cr (VI) reduction was consortium 5 (Serratia sp.-C. tropicalis) at concentrations of 100 ppm with 79.20% reduction, followed by consortium 3 (Serratia sp.- C. famata) with 73.68% at concentrations of 50 ppm (Table 2). The consortium with the best performance at most concentrations (0.5 ppm, 3.12 ppm, 6.25 ppm, 100 ppm) was 5 (Serratia sp.- C. tropicalis) (Table 2). However, none of the consortia removed 100% Cr (VI) over the 10-hour period.
Table 2 Percent reduction of Cr (VI) at concentrations from 0.5 ppm to 100 ppm in seven consortia.
Percentage of Cr (VI) reduction | |||||||
---|---|---|---|---|---|---|---|
Cr (VI) concentration (ppm) | Consortium 1 | Consortium 2 | Consortium 3 | Consortium 4 | Consortium 5 | Consortium 6 | Consortium 7 |
0.5 | 0.00 | 2.96 | 23.38 | 12.57 | 31.84 | 0.00 | 0.00 |
1.56 | 0.00 | 0.00 | 0.00 | 0.00 | 0.00 | 0.00 | 0.00 |
3.12 | 0.00 | 0.00 | 0.00 | 1.36 | 5.56 | 0.00 | 13.94 |
6.25 | 4.46 | 0.00 | 0.00 | 1.30 | 7.47 | 6.62 | 0.68 |
12.5 | 27.96 | 26.82 | 26.67 | 19.78 | 25.81 | 15.66 | 31.58 |
25 | 65.75 | 62.08 | 67.42 | 66.59 | 59.80 | 53.96 | 64.52 |
50 | 67.01 | 61.98 | 73.68 | 72.85 | 63.83 | 55.35 | 57.23 |
100 | 74.75 | 65.43 | 75.46 | 61.59 | 79.20 | 51.93 | 73.82 |
consortium 1: Serratia sp.-Raoultella sp.; consortium 2: C. famata-C tropicalis; consortium 3: Serratia sp.-C. famata; consortium 4: Raoultella sp.-C. famata; consortium 5: Serratia sp.-C. tropicalis; consortium 6: Raoultella sp.-C. tropicalis; consortium 7: Serratia sp.-Raoultella sp.-C. tropicalis- C. famata.
Total chromium quantification and removal
Consortium 5 (Serratia sp.-C. tropicalis) at a concentration of 100 ppm was selected for the evaluation of total chromium quantification and removal, because it presented the best performance compared to the other consortia and concentrations evaluated, as well as for its LRA close to 0, which indicated an adequate representation of the model in its temporal relationship. Therefore, the calibration curve of the absorbance values showed a linear fit of R2 =0.997 with initial total chromium concentration (T0) from 1.07 mg/L to 0.85 mg/L at time T5, evidencing a reduction in the total chromium concentration at the final time (Figure 4). In addition, the data obtained for Cr (VI) and total Cr to determine the reduction percentage showed a chromium removal of 31.12 % for this consortium. A sample of the calculation is shown below.
% removal Cr= Chromium 𝑉𝐼 −𝑇𝑜𝑡𝑎𝑙 𝑐ℎ𝑟𝑜𝑚𝑖𝑢𝑚
% 𝑟𝑒𝑚𝑜𝑣𝑎𝑙 𝐶𝑟=79.20−48.079
% 𝑟𝑒𝑚𝑜𝑣𝑎𝑙 𝐶𝑟= 31.12%
Discussion
The use of microbial consortia for the removal of Cr (VI) has been little addressed. However, in the present study, it was found that microbial consortia can be efficient in carrying out this reduction, since synergistic microbial interactions were evidenced in the five consortia used (Raoultella sp.-C. tropicalis, Raoultella sp.-C. famata, Serratia sp.-C. tropicalis, Serratia sp.-C. famata, Raoultella sp.-Serratia sp.-C. tropicalis-C. famata, showing a greater synergy and Cr (VI) reduction capacity in the C. tropicalis-Serratia sp. consortium, which reached removal percentages of 79.20% at a concentration of 100 ppm.
According to Zhang et al. (2018), the removal behavior of microorganisms may be due to various Cr (VI) reduction mechanisms, which include reduction by DT-diaphorase, aldehyde oxidase in the cell cytoplasm, chromium reductase, cytochrome P450 in the cell membrane and nitroreductase, mechanisms that, according to the authors, are activated when microorganisms are induced to an adaptation phase with heavy metals, because in this process the responses are usually generated by the activation or modification of some metabolic pathways, in order to cope with these toxic substances.
Likewise, Singh et al. (2019) expounded that synergistic effects may be due to increased metabolic activity of interacting microorganisms as a result of a positive association between them. This synergy in the consortia offers benefits such as producing metabolites during biodegradation processes that can be utilized by other strains (Khanpour and Partovinia, 2021). In this regard, in the five microbial consortia (Raoultella sp.-C. tropicalis, Raoultella sp.-C. famata, Serratia sp.-C. tropicalis, Serratia sp.-C. famata, Raoultella sp.-Serratia sp, C. tropicalis-C. famata), synergistic microbial interactions were evidenced, indicating that there is no antibiosis effect, competition for space or nutrients, and direct interactions that prevented the free development of the species.
In this sense, the role of bacteria and fungi in the transformation of heavy metals through their previous adaptation has been reported (Rodríguez and Quezada 2020). In particular, bacteria perform a more enzymatic process, where the ChrA protein constitutes a determinant of resistance to Cr (VI) functioning as an expelling pump of the ion. The ability for Cr (VI) to Cr (III) reduction has been found in many bacterial genera such as Pseudomonas, Micrococcus, Bacillus, Achromobacter, Microbacterium, Corynebacterium and Arthrobacter (Sharma and Adholeya, 2012) and Serratia spp. (He et al., 2018). The latter was evaluated by He et al, (2018) where they reported that chromate metabolism in Serratia sp. presents a high potential for Cr (VI) resistance and removal through a complex system of related genes ChrA, ChrB, and ChrAB, where, ChrA and ChrAB proteins expressed high resistance to Cr (VI) while ChrB gene could have a positive regulatory role.
On the other hand, fungi have also been used in bioremediation and biosorption processes of heavy metals, such as Cr (VI), performing a balance between the incorporation, expulsion, and capture of the metal (Vélez-Zuluaga, 2018). The capture of chromium on the surface of filamentous fungi and yeasts has been described as the result of its binding to cell wall polysaccharides, such as glucans, chitin and chitosan, which may be associated with proteins and other minor components such as lipids and melanins (Vélez-Zuluaga, 2018). This mechanism is used by C. tropicalis, which has been reported to have biosorption capacity, efficiently removing 80% of chromium from the medium in 12 days of incubation, due to the number of heavy metal binding sites in its cell walls, which allows it to absorb harmful metal ions in the medium and reduce them to less harmful and insoluble Cr (III) by means of the enzyme chromate reductase (Elahi et al., 2020).
The microorganisms Serratia sp. and C. tropicalis have been used in previous studies. However, both microorganisms have not been previously used in consortium. Heredia et al, (2022), identified a microbial consortium composed of species such as Staphylococcus xylosus, Saccharomyces cerevisiae and C. tropicalis, capable of reducing 98% of chromium (VI) at 10 mg/L and 67% of total chromium in textile wastewater. While, Serratia spp. has been used in bacterial consortia together with Arthrobacter sp. with an efficiency of 82.1 ± 1.22% removal of 30 mg/L Cr (VI) (Ge et al., 2021).
Finally, it is concluded that the positive association and synergy of the microorganisms evaluated, their beneficial and optimal growth in solid media, potentiates the growth of the microbial consortia established. These consortia demonstrated their resistance to chromium, with greater adaptation and removal in the C. tropicalis-Serratia spp. consortium, especially at concentrations of 100 ppm Cr (VI), with a total removal of 31.12%.
This may be due to the fact that bioactive substances such as hormones and enzymes produced by yeasts (Rodriguez and Quezada 2020) such as C. tropicalis have promoted active cell and radical division, generating substrates that were used by bacteria (Rodriguez and Quezada 2020) such as Serratia sp. that can enhance the growth of the consortium. This shows that both C. tropicalis and Serratia spp. in consortium present a positive interaction with a greater metabolic capacity for reduction with respect to the other consortia evaluated. Finally, the importance of this research is highlighted because it is the first study to report this autochthonous microbial consortium with high adaptation and reduction to Cr (VI) and with possible positive impacts in future studies of bioremediation of wastewater containing chromium.