INTRODUCTION
The expansion of water during freezing process and its shrinking during thawing process inside rock mass is the primary factor for freezing-thawing damage in underground engineering projects in cold area (Wen, 2013). The temperature differences caused by the alternation of seasons induce repeating freeze-thaw cycles of water in rock mass, lead to enormous volume expansion force and result in the damage of rock mass (Javier, 2013). The macroscopic brittle failure and strength damage of rock under freeze-thaw cycles are caused by the germination, expansion, growth and connection of many micro pore structures in the rock (Rostasy, 1980; Li, 2013). Many laboratory experiments and detection results show that the damage of rock is caused by the continuous development of microscopic pore structure in rock mass, and rock mass experiences damages during the process in which the large pore volume increases and small pore volume decreases (Mutlutürk et al, 2004). Therefore, the detection and analysis of gradual deterioration progress of rock microstructures are of great significance to both the construction of correct macroscopic constitutive relation and the accurate measurement of rock strength characteristics (Yan, 2015). The research on the relationship between the macroscopic mechanical characteristics and the gradual deterioration progress of rock mass microstructures under freeze-thaw cycles can greatly benefit and facilitate underground engineering projects.
Over the years many researchers developed the understanding through engineering practice that the effects of freeze-thaw cycle of water in rock pores change the microscopic pore structure of rock mass and cause variations in physical and mechanical properties, e.g. elastic modulus, strength and Poisson's ratio of rock mass. Through a thermal expansion straining test under single freeze-thaw cycle, Yamabe (2001) studied the relationship between the mechanical characteristics of rock and freeze-thaw cycle. The test proved that a freeze-thaw cycle causes the sample to deform elastically in axial direction and the saturated sample to deform plastically. They also found that there existed strong effect of freeze-thaw cycles on the damage of saturated rock. De Argandona (1999) detected the damage of dolomite using X-Ray, found serious weathering of rocks in a few short cycles, and assessed the damage according to the experimental results. Nicholson (2000) performed the freeze-thaw cycle test for ten types of rock, and studied the impacts of lithology on the deterioration degree of rock during freeze-thaw cycles. Zhang (2008) summarized the factors that cause the damage and deterioration of rock during freeze-thaw cycles, and studied the corresponding mechanism and mechanical properties of rock mass during freeze-thaw cycles. Liu (2014) held the view that the elastic modulus of intact rocks decreases with the increase of freeze-thaw cycles, and through laboratory test they comparatively analyzed the freeze-thaw damage change rule of joint rock mass. The strength loss of limestone in cold regions subject to freeze-thaw recycles was studied by Fatih (2012).
From the studies above, the indoor freeze-thaw test demonstrates significant effects of freeze-thaw cycles on the macroscopic mechanical characteristic of the rock. The macroscopic strength decrease of rock mass has a close relation with the gradual deterioration of micro pore structure of rocks. There are many crisscross microscopic pore structures of various sizes within the rocks. The temperature differences caused by the alternation of seasons induce repeated freeze-thaw cycles of water in rock mass, and then lead to the germination, expansion, growth and connection of many micro pore structures in rocks, and ultimately lead to macroscopic brittle failure and even strength damage of rocks.
Under different hydration conditions, the relationships between damage and deterioration of microstructure and macroscopic mechanical characteristics of rock have drawn great attention from engineers. The recent research on rock freeze-thaw mainly concentrates on the laboratory test, but the combination of laboratory findings to practical engineering projects is still scarce. The freeze-thaw cycle test and the detection of micro pore structure of rock samples after the recycle were closely linked to headrace tunneling practice. In the present work the freeze-thaw tests were carried out for two groups of andesite sample rocks in different states under the conditions of the lowest freezing temperature of -40 °C and the thawing temperature of 20 °C. Damage detection was performed by magnetic resonance imaging for the interior microstructure of rock samples subject to different freeze-thaw circles, and the relationship between the mechanical properties and the gradual deterioration of microstructures of rock mass was also discussed. The damage and deterioration mechanism of micro pore structure and macroscopic mechanical characteristic of rocks under different hydration conditions were analyzed. The results provide theoretical reference for the construction and engineering safety in cold regions.
Test of rock microstructure gradual deterioration subject to freeze-thaw cycles
Selection of samples
Andesite samples were taken from a headrace tunnel project of a hydropower station in Xinjiang and these sample lumps had dark gray surfaces and regular structures. Most rock fracture had closed and fresh surfaces with a few with slight color-fade. Occurrence was 270° ~ 285° NE <70° ~ 75° and < 275° ~ 285° NE <25° ~ 30°, smooth straight sliding surface, filled quartz vein and calcium. The physical mechanical properties of rock samples are shown in Table 1.
The headrace tunnel of the hydropower station is deeply buried, which allowed most of the tunnel surrounding rocks embedded in fresh rock mass. The onsite engineers tested the in-situ deformation of rock mass and obtained the field data of its mechanical characteristics, which provided references for the excavation of surrounding rocks and the design of lining structure. The deformation and elastic modulus of the tunnel rock in different states of stress were measured as shown in Table 2.
Table 2 In-situ deformation of tunnel rock in the headrace hydropower station.
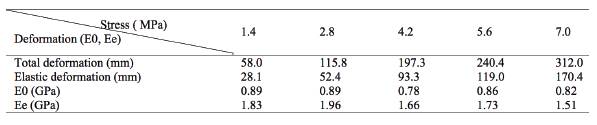
Note: The elastic modulus surface is located at a depth of 9.0 m in tunnel PD6. The rock was andesite with a smooth surface, and it was saturated for 7 days. The force on the elastic modulus surface was perpendicular to the bottom of tunnel.
Experiment Program
To understand the gradual deterioration progress of micro pore structure of rock mass under freeze-thaw cycles, the damage caused to rock samples was detected by magnetic resonance imaging. The results allowed us to understand how the micro pore structure of rock mass changes and to analyze the gradual deterioration of microstructure of rock mass under freezing-thawing cycles. The test procedure is stated as follows.
The rock samples were generated by water drilling which drilled the cores along the direction perpendicular to the bedding plane of rock mass. After cutting and further processing, ten standard cylindrical rock mass samples were made which had a height-to-diameter ratio of 2: 1, a diameter of 50 ± 2 mm and a height of 100 mm, according to the rock mechanics test regulations. In addition, the mass and size of the specimens were measured and each rock sample was visually inspected, as shown in Figure 1.
(b) The samples were divided into two groups with five samples in each group. The first group (A1, A2, A3, A4, A5) were in natural saturation state, while the second group (B1, B2, B3, B4, B5) reached the saturation state with the aid of vacuum saturating device at 0.1 MPa vacuum pressure for 4 h of pumping. Then both groups of samples were soaked in distilled water for 24 h.
(c) Based on the climate conditions at the construction site and the operating procedure of the freeze-thaw cycle test of rock mass, the freezing temperature was set at - 40 °C and the freezing time was 4 hours. Then the samples were thawed in water at temperature of 20 °C for 4 hours. This constituted a freeze-thaw cycle, and the cycle was repeated. The two groups of rock samples were subjected to repeating freeze-thaw cycles, and data were acquired at the cycle number of 10, 20, 30 and 40. TDS-300 freeze-thaw cycle machine was used for the repeating cycle test.
(d) Upon the completion of 10, 20, 30 and 40 cycles, the rock samples were measured by nuclear magnetic resonance relaxometry and nuclear magnetic resonance (NMR) system. The distribution curves of T2 spectrum of rock samples were obtained for two states and various freeze-thaw cycles. According to the area difference of sample T2 spectrum before and after the designated cycles, the rock porosity and its change rate were analyzed for different freeze-thaw cycles.
(e) The freeze-thaw deterioration process of rock samples was observed and recorded, and the test results were analyzed.
Analysis of test results
During the freezing process, the moisture in rock mass was frozen to ice. The accompanying volume expansion pushed and pressed the pore surface, which led to the extension of pore structure in rock and the generation of new pores (Matsuoka, 2001). Under saturation condition, pore structures in rock were filled with moisture. Therefore the change of pore structure in rock mass can be characterized by the signal strength of water indicated by the nuclear magnetic resonance (NMR) system, and the relationship between NMR T2 spectrum distribution and pore size was established. NMR T2 spectrum also reflected the distribution of pores. In the T2 spectrum distribution, the peak position related to pore-size, and the peak area related to the quantity of pore with corresponding aperture (Li, 2012).
The samples were imaged by nuclear magnetic resonance, and the transversal relaxation time of T2 spectrum distributions was obtained. The relaxation time of T2 is proportional to the pore diameter, while the signal strength reflects the quantity of pore with different apertures (Zhou, 2014). By observing the changes in T2 spectrum distribution, the change of pore structure in rock can be analyzed. The T2 spectrum distributions of rock samples subject to different freeze-thaw cycles are shown in Figures 2 and 3.
As shown in Figures. 2 and 3, after the freeze-thaw cycles, the T2 spectrum distribution of the rock samples exhibited two primary peaks. The morphological distribution of T2 spectrum shifted to the upper-left with the increase of freeze-thaw cycles, which indicates the enhancement in the T2 spectrum signal of small pores and the generation of new micro-pore structures in rock under the freeze-thaw cycles. Therefore, the freeze-thaw cycles gradually deteriorated the rock microstructure.
By analyzing the T2 spectrum distribution in Figure 2 and 3, it was found that the first peak in the T2 spectrum distribution is significantly higher than the second for all the samples tested. The trend shows that new micropore structures were generated in rock samples. During freeze-thaw cycles, the moisture migrated to new pore structure, and the T2 spectrum signal strength was enhanced. The results show that under the freeze-thaw cycles, pore structures of all sizes expanded inside rock mass. Not only were new pore structures generated, but also the original micro-pore structures extended, with a growth in the size of pore structure. This caused further damage to the rock microstructure.
The peak of T2 spectrum distribution for naturally saturated samples was obviously higher than that of the samples saturated by vacuum. This indicates that naturally saturated rock samples had many micro-pore structures generated after the freeze-thaw cycles, and had faster generation and expansion speeds of fracture structure. These results explain the further deterioration of rock microstructure.
Table 3 shows the rock sample porosity and its change rate after 10, 20, 30 and 40 freeze-thaw cycles. As shown in Table 3, the porosity of the rock increased with the freeze-thaw cycles and there was small variation of amplitude among the samples of each group. For naturally saturated samples, their porosity increased by 42.56% ~ 72.72% after 20 freeze-thaw cycles, 145.45% ~ 103.81% after 30 cycles and 146.71% ~ 180.24% after 40 cycles. For samples saturated by vacuum, the porosity increased by 38.46% ~ 67.74% after 20 freeze-thaw cycles, 99.67% ~ 142.12% after 30 cycles and 149.0% ~ 188.97% after 40 cycles. It is noted that the number of freeze-thaw cycles has essential impact on the porosity of rock sample. The increase of freeze-thaw cycles resulted in faster generation and expansion speeds of fracture structure, as well as more evident change of porosity and gradual deterioration of rock microstructure.
Macroscopic mechanical characteristics of the rock mass subject to freeze-thaw cycles
Test method and scheme
To establish the relationship between macroscopic mechanical characteristics and gradual deterioration of rock mass microstructure, the quality of rock samples subject to freeze-thaw cycles was measured by nuclear magnetic resonance (NMR) technique in order to investigate the rapid non-destructive detection characteristics. The samples subject to 0, 10, 20, 30, 40 cycles were tested for compression strength. The relationship between microscopic gradual deterioration and macroscopic mechanical characteristics of rock samples was analyzed for different freeze-thaw cycles.
After the rock samples were detected by nuclear magnetic resonance (NMR), compression strength test and elastic modulus measurement were immediately performed using a universal QW-W300 microcomputer-controlled low-temperature electron test equipment. The data in each group were recorded and the results were analyzed systematically.
The analysis of uniaxial compression characteristics
Due to the difference in lithology, mineral composition, porosity and water content, etc., the damage and degradation patterns in rocks subject to freeze-thaw cycles are also different. During the freeze-thaw cycles, the respective strength damage laws of rock also varied. Table 4 lists the uniaxial compression strength and elastic modulus of andesite subject to different freeze-thaw cycles.
Figure 4 and Figure 5 show the strength damage curves and elastic modulus curves of two groups of andesite under different freeze-thaw cycles. As shown in Figure 4, with fewer freeze-thaw cycles, the compression strength of andesite is stronger. The increase of freeze-thaw cycles results in decreasing compression strength. The detection of microscopic pore structure of the rock samples uncovered that new microscopic pore structures were generated with the increase of freeze-thaw cycles. During freeze-thaw cycles, moistures migrated to new pore structures. Continuous freeze-thaw cycles resulted in gradual deterioration of microstructure and larger compression strength of the rock samples.
During the test, no pore structure change was observed after small number of freeze-thaw cycles. After 10 ~ 20 cycles, the original micropore structures began to extend. In the process of freeze-thaw cycles, the newly generated micro-pore structures were observed through nuclear magnetic resonance (NMR) detection. During freeze-thaw cycles, the damage kept deepening and the cracks extended with the increase of cycles.
The test data and Figure 4 show that, the strength of andesite rock samples decreased by different amount under various freeze-thaw cycles. The strength of naturally saturated rock samples decreased by 7.66% (equivalent to 13.5 MPa) after 10 cycles, 16.5% (equivalent to 29.1 MPa) after 20 cycles, 30.17% (equivalent to 53.2 MPa) after 30 cycles and 39.4% (equivalent to 69.4 MPa) after 40 cycles, respectively. For rock samples saturated by vacuum, the strength decreased by 7.03% (equivalent to 11.1 MPa) after 10 cycles, 14.88% (equivalent to 23.5 MPa) after 20 cycles, 25.84% (equivalent to 40.8 MPa) after 30 cycles and 36.86% (equivalent to 58.2 MPa) after 40 cycles.
Based on the test data and Figure 5, the elastic modulus of naturally saturated rock samples decreased by 21.77% (equivalent to 1.5 GPa) after 10 cycles. The reduction continued for more cycles, which is 31.35% (equivalent to 2.16 GPa) after 20 cycles, 41.51% (equivalent to 2.86 GPa) after 30 cycles and 47.46% (equivalent to 3.27 GPa) after 40 cycles. For rock samples saturated by vacuum, the reductions of elastic modulus are 18.54% (equivalent to 1.17 GPa) after 10 cycles, 29.95% (equivalent to 1.89 GPa) after 20 cycles, 38.67% (equivalent to 2.44 GPa) after 30 cycles and 44.85% (equivalent to 2.83 GP) after 40 cycles, respectively.
Conclusions
The macroscopic mechanical properties of andesite deteriorate gradually with the increase of freeze-thaw cycles. The deterioration mainly shows that, both the strength and the elastic modulus decrease gradually with the increase of freeze-thaw cycles. The macroscopic strength damage of rock mass is closely related to the gradual deterioration of its microstructure. The microscopic pore structures in rocks continuously develop and expand with the increase of freeze-thaw cycles, accompanied by the migration of moisture. Continuous freeze-thaw cycles cause the gradual deterioration of microstructure and larger strength damage of the rock samples.
(2) The gradual deterioration test of micro-structure of rock samples under freeze-thaw cycles suggests that the development and expansion speed of pores vary in different freeze-thaw stages. The nuclear magnetic resonance area of each rock sample increases with the freeze-thaw cycles. There are evident and distinct changes of nuclear magnetic resonance areas of all rock samples after 10, 20 and 30 cycles, which demonstrates different rates of freeze-thaw damage in different stages.
(3) The influence of freeze-thaw cycles on the mechanical properties of andesite rock samples under different conditions is substantial. With the increase of freeze-thaw cycles, the uniaxial compression strength and elasticity modulus of rock present a clear reduction trend. The strength of naturally saturated rock samples decreased by 39.4% (equivalent to 69.4 MPa) and the elasticity modulus decreased by 47.46% (equivalent to 3.27 GPa) after 40 cycles. In contrast, the strength of rock samples saturated by vacuum decreased by 36.86% (equivalent to 58.2 MPa) and the elasticity modulus decreased by 44.85% (equivalent to 2.83 GPa) after 40 cycles. The results demonstrate evident influence of freeze-thaw cycle on the damage and deterioration of internal structure in andesite.
It should be noted that the strength damage and even deterioration caused by freeze-thaw cycles in cold regions is a complicated process in rock engineering, and this is a complex THM multi-physics problem at low temperature. The phase change of pore water and ice in the rock mass is the key factor of rock freeze-thaw damage. By microscopic detection, the relationship between the macroscopic mechanical characteristics and the gradual deterioration of microstructure of rock mass under freeze-thaw cycles was investigated in this work. The study was based on the microscopic dimensions of the gradual degradation of rock microstructure under freeze-thaw cycles, and then extended to the effect of freeze-thaw cycles on macroscopic mechanical characteristics of rock mass. The present work contributed to discovering the damage mechanism of frozen rocks. The present work suggests that further study on rock freeze-thaw cycle damage should be based on the microscopic dimensions, extend to the effect of freeze-thaw cycles on the development of rock mass fracture network, and focus on the macro mechanical characteristics of rock.