INTRODUCTION
Among the orange varieties cultivated worldwide, the orange var. Valencia belongs to the group of the most important ones, reaching 80% volume in producing countries such as Brazil. In the Colombian Orinoquia, this volume reaches 90% (Auleret al., 2008; Cunha et al., 2019).
Environmental conditions, especially agroclimatic ones, affect the ecophysiology of plants, where the influence is specific for each crop and each variety of a species; plants have adaptive strategies to quickly adapt to environmental changes (Mooney et al., 2001; Agustí, 2003; Abdelghany, 2015; Cleves-Leguízamo, 2018a; Nissi et al., 2021).
The level of connectivity between the minor agroecosystems (lots) and the larger ones (farms) and between the agroecosystems and the landscape determined the Main Agroecological Structure (MAS) (Cleves-Leguízamo et al., 2020), giving the productive systems a greater response capacity (resilience) against the occurrence of varying disturbances, including microclimatic regulations, with an increase in the functional biodiversity, which is expressed as a direct relationship with productivity and as an inverse relationship with the number of phytosanitary controls per year (Cleves-Leguízamo et al., 2017; León et al., 2018; Cleves-Leguízamo, 2018b).
According to studies by Granda et al. (2009), Montgomery and Peck (2002), and Cleves-Leguízamo and Jarma (2014) that used Multivariate Analysis techniques (Principal Components), meteorological conditions have a strong relationship with and influence on citrus crop production, finding that the lowest degree of fruit maturity (high content of acidity, low index of maturity and percentage of juice) was related to meteorological conditions that included high temperatures (maximum and average), increases in reference evapotranspiration, strong wind speed, low precipitation volumes, and low relative humidity and insolation (solar brightness).
The hydrophysical parameter evaluations carried out by Orduz and Fischer (2007) in Oxisol soils in the Piedemonte Llanero indicated that usable water for plants (difference between field capacity and permanent wilting point) corresponds to about 3.5% of the water in the first twenty centimeters of the soil, a volume that only meets up to three days of the water requirements of citrus crops.
Since Oxisols predominate the study area, citrus cultivars need to be permanently supplied with water. Oxisols are characterized by their low moisture retention, high toxicity for iron and aluminium, and deficiencies of phosphorus and calcium that limit root development and adsorption of water and nutrients (Cleves-Leguízamo et al., 2019).
Fischer et al. (2016) pointed out that environmental modifications influenced by climate change act upon the physiological processes of fruit plants, affecting the growth, development, yield, and quality of fruits differently. Excess solar radiation, together with higher temperatures, can cause photoinhibition and burn injuries in plant parts exposed during drought events.
Increased temperatures can accelerate crops, plants and seed cycles in cultivars at higher altitudes but would also increase the harmful effects of water stress and high radiation and would modify the behavior of pests, diseases, and weeds (Cleves-Leguízamo et al., 2016).
The citrus agroecosystems in the production area of the foothills of the Department of Meta (Colombia) have an adequate water supply in terms of volume and quality during a large part of the year (Cleves-Leguízamo et al., 2018b).
These conditions have made the design and implementation of irrigation systems unnecessary in adult plants given the annual water deficit necessary for the induction of flowering (Nissi et al., 2021), while the onset of rains facilitates flowering and provides enough rainfall until the harvest season (Orduz and Fischer, 2007; Orduz et al., 2010).
Nevertheless, the objective was to analyze the incidence of the most important climatological variables (temperature and precipitation) for the productivity of ‘Valencia’ orange agroecosystems (Citrus sinensis L. Osbeck) under the conditions of the Colombian low tropics to optimize cultural management practices with an empirical model.
MATERIALS AND METHODS
The present study used climatic information that was collected between 2013 and 2015, including precipitation variables (PPT) in millimeters (mm), maximum temperature (Tmax) and minimum temperature (Tmin) in degrees Celsius (°C), relative humidity (RH) in percentage (%), and solar brightness (S.Br) in light hours per day (h d-1), which were recorded monthly by meteorological stations of the Colombian national network, administered by the Instituto de Hidrología, Meteorología y Medio Ambiente of Colombia (IDEAM) and located in the study area (Tab. 1).
Table 1. Climatological stations located in the study area.
Code | Type of station | Station | Municipality | Latitude N | Longitude W | Altitude (m a.s.l.) |
---|---|---|---|---|---|---|
3502502 | CP* | Libertad La | Villavicencio | 4°3'26.5" | 73°28'4.5" | 336 |
3207504 | CO** | Holanda La | Granada | 3°30'58.8" | 73°42'57.7" | 360 |
3501004 | PM*** | Caño Hondo | Guamal | 3°55'.0" | 73°48'.0" | 800 |
3501007 | PM*** | Guamal | Guamal | 3°52'.0" | 73°45'.0" | 525 |
3206501 | CO** | Lejanías | Lejanías | 3°31'46.3" | 74°1'38.5" | 680 |
*CP: Main climatological station; **CO: Ordinary climatological station; ***PM: Pluviographic gauge station.
The evapotranspiration of the crop in reference (ETo) in mm month-1was estimated.The evapotranspiration of the crop, (ETo) mm month-1 , was estimated with the FAO Penman Monteith method (Allen et al., 2006) and the climatic variables Tmax, Tmin, HR, and S.Br on a monthly scale, and the variable of wind speed, taken constantly, with a value of 2 m s-1.
Under the climatic conditions of the foothills in Meta Department, Colombia, flowering occurs in the last week of March or the first week of April and indicates the main harvest, which begins in December for the Valencia variety (nine months after anthesis).
During 2013, 2014 and 2015, semi-structured interviews were carried out with citrus farmers, identifying the months when the phenological phases of fruit formation, fruit setting and development normally occur. This information was corroborated by Orduz and Garzón (2012). The results indicate that floral induction occurs during the hydrological deficit that begins in December and lasts until the first half of March (Orduz, 2007).
Open flowers appear in the first week of April, and the stages of anthesis, fruit set and fruit growth occur from this date until months September or October, depending on the incidence of climatic variability towards the beginning of flowering. Oranges become ripe for consumption in the first week of December, with a ratio of 9 (° Brix / % acidity).
The variables PPT, ETo, Tmax, and Tmin are used for the agroclimatic indices in the periods in each phase of formation and development of ‘Valencia’ oranges (Citrus sinensis L. Osbeck). The agroclimatic indices are presented below.
Moisture content
The deficit or total excess water that was available for the citrus crop in the phenological phases of fruit growth was determined (DNP and IDB, 2014) (Eq. 1 )
where,
Accumulated degree days index
This index is calculated considering the fact that fruit development depends on temperature.
The method of Owens and Moore (1974) was used for this index (2):
where,
The value of 2526 is constant and represents the annual cumulative degrees (oC) on an annual average in the study area.
Anomaly of the ‘Valencia’ orange crop yield
where,
The empirical model (Eq. 4), developed according to Penning de Vries et al. (1989), is also called a descriptive or black box model since it reflects the causal mechanisms of a system’s behavior. This model diagnoses the relationship between climatic variables and yield variations in ‘Valencia’ orange crops. The agroclimatic indices described above (Eq. 1, 2 and 3) were used for the development of the model.
Prior to modeling, the data were analyzed and processed for quality control. STATGRAPHICS Centurion XVI v. 16.2.04 was used to build the model and to perform a multiple regression analysis with non-linear adjustment, using the non-linear least squares method (Rivas et al., 1993; Díaz, 2012).
The coefficient of determination (R 2) was used to explain the variability of the adjusted model of agricultural performance based on the agroclimatic variables. In addition, an analysis of variance (ANOVA) was performed to estimate the statistical relationships of significance between the analyzed variables at a 95% confidence interval, which rejected the null hypothesis at a probability greater than P>0.05.
Additionally, other statistical indicators were calculated to evaluate the developed model. These included the standard error of the estimate, which shows the standard deviation of the residuals; the mean absolute error (MAE), which is the average value of the residuals; and the Durbin-Watson statistic (DW), which examines residuals to determine significant correlations based on the order in which the data are presented. The DW indicates the absence of serial autocorrelation in residuals with P<0.05, at the 95% confidence level (Díaz, 2012).
RESULTS AND DISCUSSION
As a result of the relationship between the agroclimatic indices (Eq. 1 and 2) and the anomaly index of the ‘Valencia’ orange crop yield (Eq. 3) equation 4 was modeled, which showed a significant non-linear relationship (P<0.05), based on the climatic variables PPT, ETo, Tmax, and Tmin, as presented below (Eq. 4).
where,
It should be noted that k takes values of 1 in the pre-flowering phase between the months of January and February, 2 during flowering in the months of March and April, and 3 in the phase of fruit growth from May to August.
The model of equation 4 (Fig. 1) explained 49% (P=0.0233) of the variability in the ‘Valencia’ orange crop yield in the study area, according to the agroclimatic indexes (Eq. 1 and 2), which depended on precipitation, ETo, and air temperature on a monthly time scale. This model had a standard error of the estimated or standard deviation of the residuals of 7%.
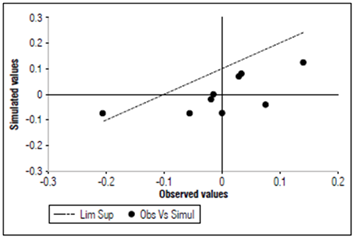
Figure 1. Anomaly of the ‘Valencia’ orange crop yield observed in the field and simulated with the empirical model (Eq. 4).
Figure 1 shows the anomalies or variations for the average value between 2013 and 2015, and for the simulated values (Eq. 4) for the same period. The simulated values were similar to and concorded with the values that were observed or measured in the field, between the limit of ±10%.
According to the perception on 92% of the farmers, changes have occurred in the climate during the last 10 years. These changes have been reflected in increases in air temperature, mainly in the season with little rainfall (January-February, and part of March) and in variations in the distribution and volume of rainfall. These changes in the climate have had negative effects on the cultivation of citrus (in the opinion of the producers), associated with erratic rainfall in dry periods, which coincide with floral induction and differentiation.
Untimely rain promotes anticipated blooms that, followed by water deficit, cause excessive and premature fall of flower buds, affecting the main crop. The main decrease in yield takes place in crops with low levels of technology, particularly with low levels of corrective fertilizers, and with low leaf area indexes (LAI) and deficiencies in mineral nutrition (Orduz and Abella, 2008; Cleves-Leguízamo et al., 2012).
This perception of local farmers was corroborated by the results of the model in equation 4, which indicated that increases in rainfall in the pre-flowering and flowering phases have an impact on decreased production. This was confirmed in studies that were carried out by Southwick et al. (1995) and Davenport (1990), who found that, to induce floral differentiation, citrus crops require between 60 and 90 d with deficit water conditions. Fischer et al. (2016) indicated that extreme climatic conditions associated with climate change and variability can cause negative impacts on crops, depending on the phenological phase.In the equatorial zone, the main climatic factor that influences growth and development of citrus fruits is the occurrence, volume, and distribution of rainfall (Orduz, 2007).
In figures 2 and 3, it is evident that, under conditions of deficient rainfall (<10 mm) in the pre-flowering and flowering phases, precipitation determined the intensity, duration and distribution of flowering, conditions that are favorable for the yield of ‘Valencia’ oranges. These results agree with reports by Reuther and Ríos (1969), Stover et al. (2002) and Aguilar et al. (2010).
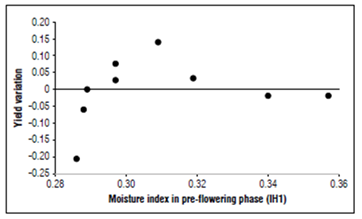
Figure 2. Variation in yield of the ‘Valencia’ orange crop, versus the moisture index in the pre-flowering phase (January and February).
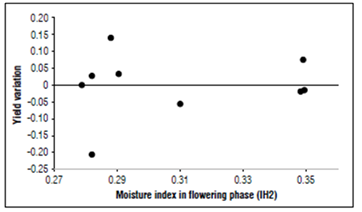
Figure 3. Variation in yield of the ‘Valencia’ orange crop, versus the moisture index in the flowering phase (March and April).
It should be noted that, during the evaluation, favorable water conditions were present in the fruit set-growth phase. That is, there were no variations in the soil moisture regime, which consequently did not affect the average yield of the area (Fig. 4 and 5).
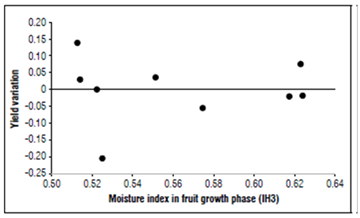
Figure 4. Yield variation of the ‘Valencia’ orange crop, versus the moisture index in the fruit growth phase (May and August).
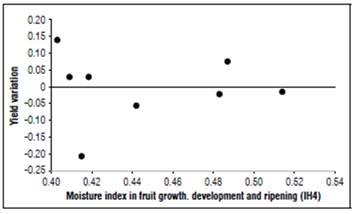
Figure 5. Yield variation of the ‘Valencia’ orange crop, versus moisture index in the fruit growth, development, and ripening phase (September and December).
According to Orduz and Fischer (2007), in the foothills of Meta Department, citrus fruits demand 1,046 mm year-1, (typical requirement for the region), equivalent to 77% of the evapotranspiration of the annual crop, which is 1,357 mm year-1, demonstrating the broad adaptation of citrus species to the environmental conditions expressed in the efficient use of water.
There was no water deficit in the soil; therefore, there were no limitations for fruit development. The water deficit in this stage was linked to the decrease in the yield components (number of fruits and size), as reported by Garzón et al. (2013), Orduz and Fischer (2007) and Nissi et al. (2021).
The greatest demand for water resources takes place in the phases of flowering, fruit set, and fruit growth; the fall of petals (anthesis) up to the end of the fruit growth stage is the critical phase, which had sufficient rainfall for the crops in the evaluated years, as shown in the graphics. On the other hand, a water deficit is required in the phase of floral differentiation.
The annual average temperature in the study area oscillates between 26 and 27ºC, which results in 4,948 units of heat/year (HU). Equation 4 shows that temperature increases in the flowering and pre-flowering phases favor crop yield, but, if the increase appears in the fruit setting phase with a water deficit, they could negatively affect fruit production, particularly in crops with nutritional or sanitary management deficiencies.
However, the average temperature in the fruit development phase during the study period (September to November) ranged between 25 and 26oC, with thermal gradients (difference between day and night temperatures) of less than 10oC on average; therefore, no significant influence was seen in the yield variation, as reported by Orduz (2007) and Orduz and Avella (2008).
The results for the studied years should be discussed and analyzed. In order to analyse the influence of climate change on the frequency, duration, and intensity of the maximum, minimum and average extreme temperatures, along with the impact on crops, more complex methodologies should be used.
The studies by Reuther (1973) indicated that the average temperature in the growth phase of fruits accelerates the physiological process of fruits, increasing volume and reducing the time required until harvest. The results indicate that there was a relationship between temperature and the growth phase of the fruits, and this relationship was non-linear.
CONCLUSIONS
Yield variations in ‘Valencia’ orange crops (Citrus sinensis L. Osbeck)in the Department of Meta are highly influenced by climatic conditions, mainly by those related to the occurrence and volume of precipitation.Increases in average air temperature and water deficits in the pre-flowering and flowering phase positively favor floral induction and productivity in citrus crops. Increases in temperature in the flowering-curd phase negatively affect production.
The proposed model takes into account the agroecological attributes (ecosystem and cultural) implemented by the citrus growers.The model explained the variability of the ‘Valencia’ orange crop yield, depending on the availability of water in the soil and the occurrence of temperatures for the months during the critical phases of the crop (pre-flowering, flowering, and fruit set).
The design and implementation of adaptive measures are recommended to reduce negative effects on the cultivation of ‘Valencia’ oranges from the threat of droughts, excess rain, or inadequate distribution of rain with respect to crop needs, which are associated with both variability and climate change. Adaptive measures must include adaptation of local irrigation infrastructures, adequate management of water resources, and use of materials with tolerance to conditions of water and/or thermal stress. It is important to note that, in the study area, especially in the municipality of Lejanías, irrigation systems have been implemented, mainly in the Tangelo Minneola (C. reticulata Blanco×C. paradisi Macf) technical crops. These systems seek to modify the date of flowering and harvest of this high-value fruit.