INTRODUCTION
Quinoa (Chenopodium quinoa Willd.) is a plant that belongs to the Amaranthaceae family and is an American Andes native species which has expanded throughout all continents during the last decades (Jacobsen, 2003; Bazile et al., 2016). The average yield ranges from 2 to 5 t ha-1 (Melo, 2016; García-Parra and Plazas-Leguizamón, 2018) with fertilizer applications ranging between 60 and 150 kg ha-1 of N, 25 and 100 kg ha-1 of P2O5, and 80 and 20 kg ha-1 of K, as recommended by Geren (2015); Gómez and Aguilar (2016); Hussain et al. (2018), and Nishukawa (2012). Approximately 70% of the nitrogen and phosphorus applied through fertilization is not absorbed and results in runoff which causes eutrophication in nearby water sources (Schindler et al., 2008).
Globally, the use of chemical products that increase yields are where most spending is used, this leading to decreased incomes for producers. In organic production systems, mineral sources are not permitted and the mineralization of organic fertilizers is very slow (Baldi and Toselli, 2014). Additionally, small amounts of these fertilizers are used particularly in short-cycle crops.
Improving the physiological and productive parameters of quinoa in addition to assessing its ability to tolerate adverse edaphoclimatic factors such as modification of diurnal and nocturnal temperatures, photoperiod, water deficit, edaphic salinization levels, and CO2 concentration are of great importance (Christiansen et al., 2010; Lesjak and Calderini, 2017; Hinojosa et al., 2018; Sanabria and Lazo, 2018; Issa-Ali et al., 2019). These protocols must be supplemented by other strategies that guarantee successful crop production, such as the association of beneficial edaphic microorganisms (Pérez-Moncada et al., 2015; García-Parra et al., 2022).
For this reason, the presence or use of edaphic microorganisms associated with plant roots has a fundamental role in crop production, mainly growth promoting bacteria, whose results show an improvement in agronomic and productive parameters, such as those studied in corn (Zea mays L.) (Mehnaz et al., 2010), soybeans (Glycine max [L.] Merrill) (Ku et al., 2018), rice (Oryza sativa L.) (Gholamalizadeh et al., 2017), and olive trees (Olea europaea L.) (Bello et al., 2016).
Nevertheless, several studies indicate the importance of edaphic microorganisms in quinoa plants which improve agronomic parameters and resistance to extreme conditions (Ortuño et al., 2013; Yang et al., 2016; García-Parra et al., 2022). Even so, information on the benefits of the inoculation of quinoa with growth-promoting microorganisms is limited. As far as we are concerned, the growth and development of quinoa plants is determined by the presence of microorganisms in the rhizosphere zone. Hence, the hypothesis of this study establishes that the plant growth promoting bacteria inoculation favors the growth of quinoa plants. In this context, the aim of this study was to evaluate the effect of the inoculation with plant growth promoting bacteria on the phenological performance of Amarilla Maranganí quinoa cultivar using six bacteria strains recognized by their ability to promote plant growth.
MATERIALS AND METHODS
The study was conducted at the Universidad Pedagógica y Tecnológica de Colombia - UPTC in Tunja at an altitude of 2,690 m a.s.l. and coordinates of 5°33'10" N and 73°21'23" W. The study took place in January 2017 with an average temperature of 18.6°C, relative humidity of 72.3%, and an average daily illumination of 689 ± 395 µmol m-2 s-1.
Microorganisms
The microorganisms used for the treatments came from bacterial isolates developed by the Biología Ambiental Research Group of the Universidad Pedagógica y Tecnológica de Colombia and were obtained from the study by Ávila-Martínez et al. (2015). These microorganisms are characterized by having characteristics associated with high nitrogen fixation, high pathogenicity in crops of commercial interest, and some with little importance in soil biology (Sousa et al., 2015).
Plant material
The quinoa cultivar (Chenopodium quinoa Willd.) used was Amarilla Maranganí with varietal origin from Peru (Torres et al., 2000) and donated by the Germplasm Center from the government of Boyacá. Seeds were germinated under controlled conditions of a relative humidity and temperature in Petri boxes and suspended on cotton plates. Germinated seedlings were selected to achieve homogeneity and vigor mainly in relation to the dimensions of the stem, leaves or radicle, and with the absence of malformations.
Microorganism inoculation
Each characterized species was prepared in suspension (Yeast Extract Calcium Carbonate Glucose Agar, Oxoid), at a concentration of 1·10-9 UFC/mL. The bacteria were grouped together under the same concentration to be applied to each quinoa seedling 10 days after germination (DAG). Subsequently, each seedling was planted in the field, under open conditions, and in a soil with inseptisol characteristics with 102 mm of precipitation during the trial and an average temperature of 13.4 °C.
Experimental design
The experimental design used in the study was a randomized block, with three replicates, and 10 plants considered as experimental units. Nine treatments were evaluated: Bacillus macerans; Bacillus laterosporus; Bacillus licheniformis; Bacillus cereus; Actinobacillus; Pseudomona aeruginosa; Consortia; DIPEL®, and control without inoculation.
Variables evaluated
Seedling length, panicle length and plant height: Seedling length was determined 30 DAG, using a rigid flex meter from the base of the stem to the terminal bud. Likewise, the length of the panicle was measured at the time of the physiological maturity of the plant (140 DAG), taking the measurement from the base of the first floral pedicel to the apex of the main inflorescence. The height of the plant was measured the same way as for the seedling length.
Number of panicles: The total number of biggest secondary pedicels from the main stem, which were characterized by the presence of inflorescence in the terminal part.
Dry weight of the plant: This was determined by taking plants at the time of physiological maturity and placed in a muffle (CE_Standard 180°C - Madrid, Spain) at 60 °C for 10 h and then at 30°C for 14 h.
Seed production per treatment: Plants harvested individually from each treatment were tested, collected in the physiological maturity phase, and harvested manually following the methodology proposed by Lesjak and Calderini (2017).
Statistical analysis
The data was analyzed by reviewing the assumptions of normality and homogeneity through the Shapiro Willk and Barttlet test. The data from the different variables were subjected to an analysis of variance and a test of means comparison (Tukey P<0.05). Additionally, some main components were analyzed by principal component analysis to describe the behavior of the variables. A grouping analysis that determined the similarity between treatments was also performed which used the statistical program R version 3.6.1.
RESULTS AND DISCUSSION
Seedling length, plant and panicle
According to the analysis of variance, it was observed that the height of the seedling at the beginning of the vegetative phases (30 DAG) and the height of the plant at the time of physiological maturity, presented significant differences between treatments. Conversely, the length of the panicle did not show any significant differences. A greater growth of seedlings and plants was observed in the inoculation treatment with B. licheniformis while in the length of the panicle there were no statistical differences (Tab. 1).
Table 1. Height of seedlings, plant height, and panicle height of the Amarilla Marangani quinoa variety subjected to inoculation by different microorganisms
Treatment | Seedling height (cm) | Plant height (cm) | Panicle height (cm) |
---|---|---|---|
B. macerans | 8.7 c | 219 a | 28.3 a |
B.laterosporus | 10.95 bc | 211.9 b | 31.6 a |
B.licheniformis | 14.6 a | 223.7 a | 34 a |
B.cereus | 12.8 ab | 191.9 b | 28 a |
Actinobacillus | 10.7 bc | 194.3 b | 30.2 a |
P. aeruginosa | 13.1 ab | 194.1 b | 34.4 a |
Consortia | 9.15 c | 159.5 c | 29.9 a |
DIPEL® | 10.7 bc | 164.8 c | 30.9 a |
Control | 11 bc | 156.5 c | 29.1 a |
Means with different letters indicate significant statistic differences according to the Tukey test (P<0.05).
The availability of nutrients is a determining factor in the physiological and phenological behavior of quinoa which is why elements such as nitrogen favor multiple metabolic activities. Among these activities is the synthesis of phytohormones and the subsequent division and cellular elongation and in which bacteria play an important role (Taiz and Zeiger, 2006). Particularly for quinoa, Ortuño et al. (2013), recognized the benefit of the application of microorganisms through biofertilizers, favoring agronomic parameters of the crop such as the production of stems and leaves as well as recognizing the use of this biological strategy as a favorable alternative in adapting to the effects of climate change.
According to Hinojosa et al. (2018), quinoa benefits from different groups of bacteria when it is under agro climatic stress. This allows plants to have the minerals and water available in order to tolerate prolonged physiological stress periods. However, the use of rhizosphere microorganisms in crops of C. quinoa has taken relevance mainly for the promotion of leaf area indices, growth rates, and plant development rates, taking into account the role that nitrogen, phosphorus, and potassium availability have in these variables. Results from Kansomjet et al. (2017) are consistent with the results obtained in this study where inoculated bacterium such as Bacillus licheniformis, favor nitrogen fixation and in some cases tolerance to salinity, as reported in grasses (Singh and Jha, 2016).
Number of panicles
The phenotypic features of the inflorescence, largely describe the genetic qualities of the species and the agro climatic characteristics in which they grew in. According to the analysis of variance, significant differences were found in the number of branches in the quinoa plants exposed to different plant growth promoting microorganisms. Inoculation with Actinobacillus favored the number of panicles in a variety of Amarilla Maranganí plants (Fig. 1).
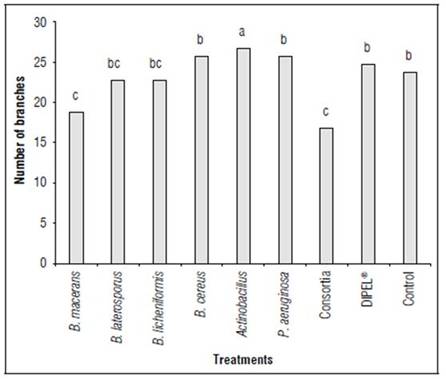
Figure 1. Number of branches in quinoa plants, exposed to different plant growth-promoting microorganisms. Different letters indicate significant differences according to the Tukey test (P<0.05).
According to García-Parra et al. (2019), the branching in quinoa plants is the result of the architecture and growth according to the water, nutrient availability, and agro-climatic conditions. Nevertheless, this variable has not been considered when inoculating microorganisms that promote plant growth in previous studies. According to Cameron et al. (2012), bacteria such as Actinobacillus have the ability to fix and mobilize nitrogen under extreme conditions which would become an alternative to inoculating bacteria that favor the availability of elements in plants while also increasing the ability to tolerate extreme weather and soil conditions.
Seed production
The inoculation of different growth promoting bacteria influenced the productivity of quinoa plants according to the analysis of variance. Thus, it was possible to identify that the presence of P. aeruginosa favored 52.8 % of seed production compared to the control treatment (Fig. 2).
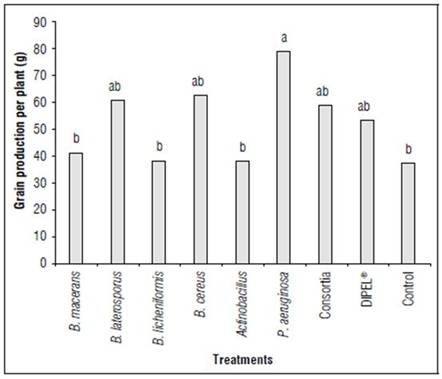
Figure 2. Grain production of quinoa plants, exposed to different plant growth-promoting microorganisms. Different letters indicate significant differences according to the Tukey test (P<0.05).
Based on the evaluation of García-Parra et al. (2019), the application of organic fertilizers favors seed production in quinoa plants due to the microbiota present in biofertilizers produced from organic waste (García, 2006). It may be suggested that the presence of diazotrophic microorganisms favor crop nutrition compared to mineral fertilization.
Pseudomona aeruginosa stood out for their high prevalence at the rhizosphere level (Steindler et al., 2009). Among their mechanisms of action in vegetables, it has been found that they favor access to water over some nutrients as well as producing phytohormones and acting as pathogen regulators. Furthermore, it has been found that this bacterium provides some amino acids and fatty acids of low molecular weight that in some cases are absorbed by plants and translocated to landfill organs (Linu et al., 2019).
According to the principal component analysis (Fig. 3) the cumulative variance of the two first components was 61.3% (CP). It was possible to determine that the treatments that promoted the highest height of the plants were B. macerans, B. laterosporus, and B. licheniformis. Those that improved the length of the panicles were B. laterosporus, B. licheniformis, and P. aeruginosa. The treatment with P. aeruginosa was the only one to increase both seed weight and the number of panicles. Therefore, the importance of these bacteria at the rhizosphere level in quinoa plants is seen and agrees with various studies that affirm the diversity of microorganisms favoring the expression of certain phenological and productive aspects of crops (Sharifi and Ryu, 2018). It is also important to note that rather than a specific species of bacteria being involved in the phenotypic variability of plants, various species of bacteria are responsible for plant growth (Glick, 2012).
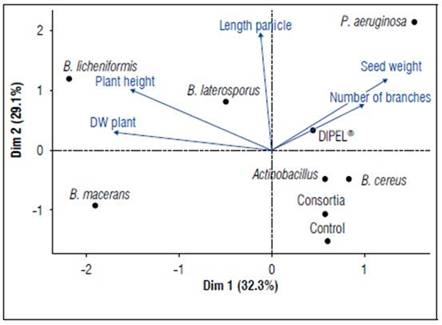
Figure 3. Principal component analysis (PCA) biplot from the application of growth promotingbacterias on Amarilla Marangani.
A bootstrap analysis allowed us to determine the characteristics that the plants exposed to the different treatments used shared. This allowed the bacteria to be grouped according to the evaluated variables. Figure 4 shows two groups that were found to share a similarity between treatments and dissimilarity between treatment groups. Those were Group A (B. licheniformis and B. macerans, characterized by having a longer plant length and a greater dry weight) and Group B (Control, Actinobacillus sp., DIPEL®, B. laterosporus, B. cereus, Consortia, and P. aeruginosa, characterized by having lower dry weight and shorter plant length). At the same time, Group B contains 2 subgroups. B1 consists of P. aeruginosa and is characterized by having greater panicle length. B2 is composed of the Control, Actinobacillus sp., DIPEL®, Bacillus laterosporus, Bacillus cereus, and Consortia which were characterized by having a shorter panicle length. B2 can be further subdivided into two groups in which the first corresponds to treatment with Consortia and fewer panicles, while the second group contains Control, Actinobacillus sp., Dipel, Bacillus laterosporus, and Bacillus cereus treatments characterized by having a greater number of panicles.
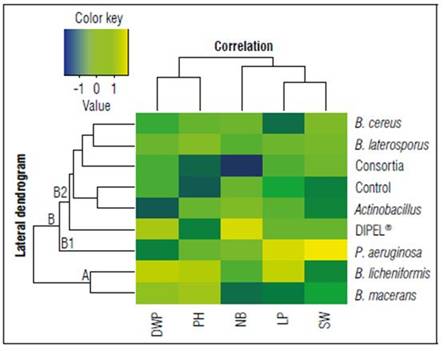
Figure 4. Bootstrap-based clustering analysis with Manhattan distance from the application of growth promoting bacterias on Amarilla Marangani quinoa cultivar. DWP: Dry weight of the plant, PH: plant height, NB: Number of branches, LP: Length panicle, SW: Seed weight.
Each subgroup is differentiated by the characteristics that suit it, Group A shares characteristics such as greater dry weight of the plant and greater length of the plant whereas Group B shares characteristics such as a greater number of panicles and greater grain yield per plant.
CONCLUSION
The use of plant growth promoting bacteria in the cultivation of quinoa favors different growth parameters. These include the length of structures such as stems and inflorescences, but also increases in the production of panicles and therefore the yield of grain.
When implementing the use of biofertilizers based on plant growth promoting bacteria in quinoa crops, (Chenopodium quinoa W.) the yellow variety of Maranganí, Bacillus licheniformis and Pseudomonas aeruginosa were found to be the most efficient in the growth and production of quinoa.
This response was obtained in quinoa plants established under the edaphoclimatic conditions in Boyacá which proposes the use of edaphic microorganisms for the improvement of the agronomic parameters of the species.