1. Introduction
In recent years, consumers have been demanding healthier foods, with high amounts of nutrients and functional components. For the manufacturing of cereal-based foods, such as those called ready-to-eat foods, there are now biofortified cereal grains, especially maize and rice. Biofortification is a method for developing a crop with a substantial increase in its nutritional value. It is characterized by the use of traditional best practices of improvement to obtain crops with high nutritional levels [1]. For the production of processed cereals, quality protein maize (QPM) and rice, both Zn-biofortified, are now available. Grain threshing residues, called bran, which are an important source of fiber, protein and lipids, can also be used in various applications in the development of these foods. In the threshing of grains such as corn and rice, many nutrients are lost, but biofortification of these crops increases the concentration of essential nutrients in the endosperm of their grains, thus compensating the loss of these nutrients in the aleurone layers removed in these processes. However, in the development of a new food, such as a ready-to-eat cereal, the addition of bran, due to its high fiber content, can affect the textural characteristics of the product.
Quality protein maize (QPM) was developed with higher levels of lysine and tryptophan and it is claimed that the content of those aminoacids can be twice as much as they are in conventional maize. Maize protein contains 1.6% of lysine and 0.47% of tryptophan, while QPM contains an average of 3.1% of lysine and 1.0% of tryptophan [2,3]. The target content of Zn in biofortified maize is 38 mg/kg in the whole grain and in biofortified rice 28 mg/kg in polished grain [1].
For the processing of various foods, such as cereals, extrusion cooking is now of the utmost importance. It is a continuous processing technique that allows for high versatility in terms of the moisture content of the processed material, its composition, the working temperature, the range of pressures used, among other operating conditions [4,5,6]. Extrusion cooking is a process carried out at a high temperature and in a short period of time. The use of bran generated in the threshing of biofortified rice and maize was studied for the production of a food by extrusion with a mixture of flour from these two cereals. Due to its short processing time, this technology can minimize the loss of nutrients.
Extrusion processing of fiber contained in cereal bran, either alone or as an extruded blend, has been suggested as a possibility in the development of new ready-to-eat cereals [7]. The fiber can impart a gritty texture to the product, so the maximum quantity to apply in a cereal blend must be determined. Research studies by Mendonca et al [8] showed that the content of maize bran used in extruded blends significantly affects the texture and appearance and general acceptability of expanded snacks. Chassagne-Berces et al. [9] showed that the incorporation of wheat and oat bran affected the final texture of extruded bran, increasing the breaking strength of the samples with the highest content of this bran. Yanniotis et al. [10] evaluated different concentrations of wheat fiber in combination with pectins in extruded formulations, finding that the greater the concentration of fibers present, the greater the radius of expansion and the lower the porosity, while increasing hardness. Robin et al. [11] found that the addition of dietary fiber to extruded cereals impairs product quality by reducing product expansion, increasing density and hardness and decreasing crispness.
2.1. Raw material and equipment
Bran and meals from white QPM and rice, both biofortified with, Zn were used as samples. The cereals, provided by the CLAYUCA Corporation, were grown at the Tropical Agriculture Center-CIAT. The processing equipment was a co-rotating, intermeshing twin-screw Jinan SAIXIN extruder, model DS 32-II (Jinan Saixin Machinery Co, China). The extruder was equipped with a PLC system to program and control the temperature, the rotation speed of the screws and the feeding rate. The temperature can be programmed in three zones inside the barrel, at the entering location of the material into the barrel, at the middle and at the end of the barrel before the exit of the extruded product. In order to obtain the maize bran, the whole grain was dehulled and degermed in a semi-industrial degerminator for 10 min. In the case of rice, the grain was husked and polished in laboratory-scale equipment to separate the husk and bran from the endosperm, respectively. The threshed corn grains were reduced in size and sieved in an industrial mill and classifier, obtaining products with different granulometry. The polished or white rice was milled in a hammer mill on a laboratory scale, where two products with different granulometries were obtained.
The bran was treated to control its rancidity process due to its oil content; this treatment was performed on the extruder operated at 130 °C, at a 200 rpm screw rotation speed [12].
The maize and rice flour and bran were separately conditioned to a moisture content of approximately 15 % (wb), prior to the preparation of the bran and flour mixtures designed for the extrusion experiments. The moisture content of the materials was determined by the kiln method at 105 °C for 24 h [13].
2.2. Extrusion process
Following the extruder usage protocol established by Albán and Montero [14], the extruder was programmed at a screw rotation speed of 200 rpm and a temperature inside the extruder barrel of 160 °C, which were parameters established from preliminary tests.
Mixtures were prepared at 15% moisture content of maize flour (MF), rice flour (RF), rice bran (RB) and maize bran (MB), with total bran in the mixture formulated at two levels, 25 and 30 %. As a result, the total flour in the mixture was 75 and 70 %, respectively. Flours were formulated with 40 % maize flour and 35 % rice flour, when the total bran was 25 %, and 40 % maize flour with 30 % rice flour, when the total bran was 30 %. Within bran, the proportion of rice bran was tested at levels ranging from 0 to 10 %.
The extruded product was cooled to room temperature as soon as it was obtained. The moisture content of the extruded materials was then adjusted to approximately 4 % (wb) in an oven set at 50 °C for 24 hours.
Other extrusion trials were made at 170 °C and 180 °C at the end of the barrel with a formulation of maize and rice flours - bran selected from the results of the previous designed tests.
2.3. Physical and nutritional characterization of raw and extruded materials
A granulometric analysis of the raw materials was performed using a set of Tyler series sieves. Likewise, the amounts of protein, total lipids, ash, crude fiber, total carbohydrates, zinc and amino acid profile of the raw materials and extruded materials obtained from the experimental treatments were determined. These analyses, except for the amino acid profile, were carried out in the Analytical Services Laboratory of the International Center for Tropical Agriculture (CIAT), where the standard AOAC methods were used [13], the amino acid profile was determined by high performance liquid chromatography using a Prominence Equipment with a Shimadzu (Japan) UV detector, a column ACF® C18-AR, for which samples were analyzed at a specialized private laboratory.
Pieces, 20 mm long, of the expanded extrudates were analyzed to determine their physical characteristics of interest to the extrusion process, the expansion ratio (ER), the particle density kg/m3 and the indicator of crispness (here in referred to as crispness work, WC, N.mm). The equations for each of these parameters are presented below [15].
Where De = Extrudate diameter (mm) and Dd = Die hole diameter (5,2 mm)
For the determination of Wc, extrudates were punctured using a EZ Test Shimadzu Texture Analyzer, fitted with a 500 N cell load and a 4 mm diameter cylindrical flat-faced probe, with an advance speed of the probe set at 25 mm/min. All samples were punctured for a distant of 3 mm lengthwise, and a minimum of 10 measurements were carried out for each sample.
From a force-deformation curve, as shown in Fig. 1, the following parameters were determined:
Where F is the average puncturing force, A is area under the force-deformation and d the length of punction.
Where Nsr is the number of spatial ruptures, No is the total number of peaks. This methodology has been widely used by other researchers with good results.
For the execution of the experimental extrusion tests, a factor design was used, with a level of significance of 95 %. Statistical analyses were performed using Minitab 17 software.
3. Results and discussion
Maize was received from the field already shelled (150 kg), in the milling process, 75 % was dehulled and degermed grain, the rest of the material was called maize bran. The bran was immediately stored at 10-12 °C to prevent rancidity. Rice grains were received as paddy rice (60 kg). After husk removing about 81 % was converted into brown rice. The rice brand resulted from whitening and polishing the brown rice; the main product was the white milled rice. Rice brand was also stored at low temperature to slow lipases activity.
Maize and rice bran contain a significant amount of lipids, so there is a possibility of hydrolytic rancidity in these components, which can significantly reduce the shelf life of the cereal. Therefore, the bran was treated by applying heat to the extrusion equipment, according to the conditions established in previous studies by Guevara and Fernández [12].
The Tyler sieve analysis of particle size distribution of raw materials yielded the following: 82 % MF with particle size between 16 mesh and 20 mesh size sieves, 80 % RF with particle size between 20 mesh sieve and 100 mesh sieve, 83 % MB with particle size between 16 mesh and 100 mesh size sieves and 83 % RB with particle size between 30 mesh sieve and 70 mesh sieve. Then, the coarser material was the maize flour with a particle size distribution quite close to that specified for maize grits in the production of snacks of a cruncher texture [5]. The other cereal products had wider particle size distributions.
The nutritional content of rice and maize and of the bran obtained is shown in Tables 1 and 2. Analysis of rice shows that rice bran has a higher crude fiber and protein content. In addition, it had a high lipid content, which justifies the importance of taking the bran to a heat treatment stabilization process.
Table 2 Content of Lysine and Tryptophan in the protein of milled products from maize and rice.
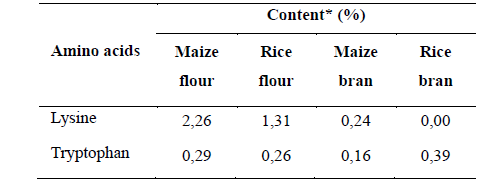
*Percentage based in the content of protein.
Source: The Authors.
The zinc content, an important nutrient in cereal biofortification, was the highest in corn bran.
Figs. 2 and 3 shows the range of mechanical responses (force-deformation plot) exhibited by extruded samples. Significant differences in the shapes and amplitudes of the force-deformation curves can be observed, evidencing the response of a crispy texture as compared to hard texture.
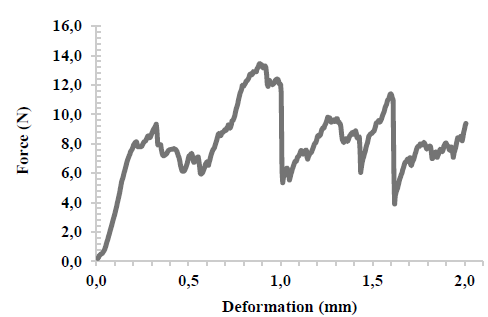
Source: The Authors
Figure 2 Force-deformation curves for extrudates of blends of maize and rice flours and bran in a crispy product.
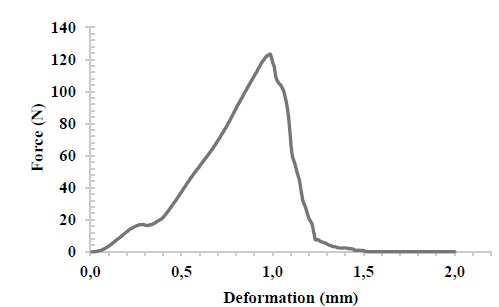
Source: The Authors.
Figure 3 Force-deformation curves for extrudates of blends of maize and rice flours and bran in a hard product.
Figs. 3 and 4 shows the evolution of the calculated expansion ratio (ER) and crispness work (WC) as the rice bran content was increased in the extrudates. The measured densities of extrudates were excluded from this results due to the high variance in the means values. But the general tendency was an increase in the extrudate density as the rice bran was increased in the blend.
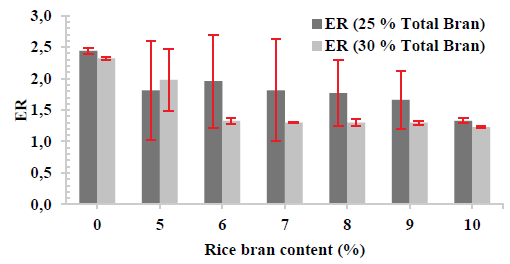
Source: The Authors.
Figure 4 Expansion ratio (ER) of extrudates as a function of the bran contents.
From these results, it can be directly inferred that the higher the content of rice bran the extrudates presented, the greater crispness work. This phenomenon can be attributed to the fact that the lipids present in the rice bran exert a lubricating effect inside the extruder barrel. This may influence dough flow properties, preventing that dough under processing are mechanically sheared with sufficient intensity between the screws, preventing the complete gelatinization of the starches present, and consequently the expansion of the extrudate. In addition, the presence of fiber in bran has a deleterious effect in the radial expansion, rupturing the walls of the air cell as well as the external surface of extrudates, thereby preventing the full expansion of the gas bubbles [8,16].
An increase in the WC means a harder product, associated with a reduction in the expansion capacity of the extruded product and an increase in its density.
In the extrusion trials, a control sample was processed, without the addition of bran, which turned out as expected with the lowest values of crispness work (WC = 1,43 ± 0,03 N.mm) and the highest expansion ratio (ER = 2,92 ± 0,04).
Based mainly on the desirable profile of expansion, density, crispness and general acceptability, as is in the control sample, the blend with addition of rice bran which was selected as the best was the formulation with 40 % of maize meal flour, 35 % of rice flour, 20 % of maize bran and 5 % of rice bran.
Taking into account the results of the variance analysis (ANOVA), the amount of rice bran and total bran had no significant effect on the ER (value p>0.05), i.e. there was no difference between the mean values of the treatments. On the other hand, the quantity of total bran had a significant effect on density (p<0.05), so there are differences between the mean values of the treatments. For WC, the amount of rice bran and the interaction between this factor and the amount of total bran had a significant effect (value p<0.05).
In subsequent extrusion studies with the selected mixture, at extrusion temperatures at 180, 170 and 160 °C at the barrel outlet, a descriptive sensory analysis of the extruded products was performed by a group of panelists trained in the evaluation of texture descriptors such as cohesiveness, crunchiness and granularity, in order to qualify these sensory characteristics. The extruded 170 °C sample was rated with the closest texture profile to the control sample [17].
Since biofortified cereals were used in this study, whether the extruded end product contained an acceptable amount of the nutrients lysine, tryptophan and Zn was verified. The extruded samples at the temperatures specified above were analyzed. The results of these analyses are shown in Table 3.
Table 3 Contents of Zn, Lysine and Tryptophan in extruded blends formulated with 40% of maize flour, 20% of rice flour, 20% of maize bran and 5% rice bran and processed under the specified conditions
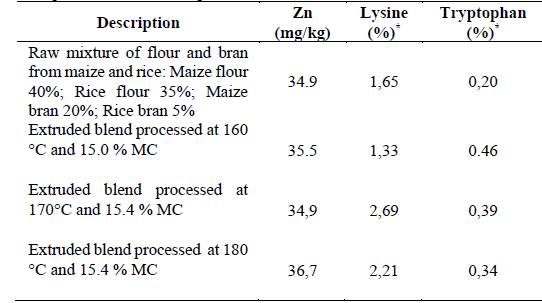
Source: The Authors.
According to the results shown in Table 3, the amount of lysine in unprocessed formulations processed at 160 - 180 °C with a moisture content of approximately 15 % meets the minimum consumption recommendations of the FAO [18]. Regarding the results obtained for Zinc, its concentration is around the values established as a goal in maize biofortification programs and above the goals set for rice [1].
4. Conclusions
The inclusion of maize and rice bran affected the expansion, texture and general appearance of products in the ready-to-eat cereal category processed by twin-screw extrusion with grain meal from biofortified corn and rice crops. The higher the bran content, the harder the product became; the less crunchy, denser/the less, and less expanded it became. The best desirable texture and expansion profile in the product was obtained by mixing 40 % maize flour, 35 % rice flour, 20 % maize bran and 5% rice bran. The ready-to-eat cereal product made from biofortified maize bran and maize flour and rice flour is believed to have a good fiber content and the nutrients lysine, tryptophan and zinc.