1. Introduction
Polymer flooding is one of the EOR techniques most employed at a commercial scale, with more than 865 projects reported worldwide [1,2]. The most successful projects have been developed in countries like China [3,4] and Canada [5-7], with incremental oil recoveries between 5 and 20%.
HPAM polymers, which are copolymers composed of AMD (Amide) and AA (Acrylic Acid), are the most common substances used in EOR due to two main implications: the volumetric sweep efficiency improvement and the residual oil mobilization by viscoelastic effects [8-12]. However, the latter mechanism is questioned by some authors who suggest that reductions in the residual oil saturation are caused by wettability alteration [13]. HPAM polymers have limitations related to high temperature [14], high salinity, and hardness brine environments [15,16]; contrary to modified AM-ATBS copolymers which present higher resistance to reservoir temperature and brine salinity [17]. It is worth considering that the success of a polymer flooding application, beyond the molecular structure of the polymer itself, relies on the understanding of additional concepts like retention [18], inaccessible pore volume [19], and permeability and mobility reduction [20].
Polymer retention has been identified as one of the most relevant variables in a polymer flooding process. It includes three complex mechanisms, which are difficult to calculate at porous media level: (1) Mechanical entrapment, (2) Hydrodynamic retention, and (3) Dynamic adsorption [21,22]. Adsorption is the most important mechanism. It occurs by chemical and physical interaction between the polymer backbone and the rock surface [23], such as Van der Waals forces, electrostatic interactions, and hydrogen bonds [21,22]. Nevertheless, besides adsorption on the rock surface, the polymer can be retained at pore structure by other phenomena like mechanical entrapment and hydrodynamic retention. At the laboratory level, it is hard to identify the dominant mechanism in polymer retention. Several scientific works have shown that dynamic adsorption is the main process responsible for retention in high permeability rock samples [24], while mechanical entrapment dominates dynamic adsorption in low permeability samples [24-27]. Nevertheless, few authors acknowledge the specific retention mechanism, and, in general, it is referred to as polymer's dynamic adsorption. This work will refer to polymer adsorption as a total retention mechanism.
In HPAM and modified HPAM copolymers, adsorption is considered an irreversible process [28-31] that likely to vary with injected polymer's concentration [32,33]. Furthermore, other variables affecting polymer adsorption like polymer type [16], MW [18,34], hydrolysis, polymer concentration, rock's mineral content, brine salinity and hardness [35,36], and rock permeability [20] have been studied given their importance to design efficient polymer flooding processes [34].
In sum, current literature refer to the dependence of HPAM polymer retention on variables like brine salinity, polymer hydrolysis, polymer concentration, and rock permeability. However, few studies consider AM-ATBS polymers to determine the correlation of these variables on polymer's adsorption. The purpose of this work was to perform experimental evaluations of HPAM and AM-ATBS copolymers to assess the effect of variables such as molecular weight, hydrolysis, brine salinity, brine hardness, and porous medium permeability on dynamic adsorption. This paper is aimed at improving understanding of this topic; and providing better guides to select the suitable polymer for a specific application, minimizing polymer losses and preventing possible blockage at pore scale.
2. Experimental development
2.1 Fluids
2.1.1 Brine
The synthetic brines were prepared by dissolving sodium chloride (NaCl), calcium chloride dihydrate (CaCl2*2H2O), potassium chloride (KC1), and magnesium chloride hexahydrate (MgCl2*6H20) in de-ionized water. Brines were filtered through a 0.45-micron cellulose-like membrane. The properties used to differentiate the brines were the amount of total dissolved solids (TDS, in mg/L) and hardness (R+), defined as the molar ratio of divalent cations and the total number of moles of cations in the brine (eq 1):
where;
[Cdiv]: number of moles of divalent cations in the brine.
[Cmono]: number of moles of monovalent cations in the brine. Table 1 lists the brines used in this research, where hardness values range from 0 to 11.6%.
Table 1 Brines
Brine | Na+ (mg/L) | K+ (mg/L) | Ca++ (mg/L) | Mg++ (mg/L) | TDS (mg/L) | Cl- (mg/L) | R+ (%) |
---|---|---|---|---|---|---|---|
S1 | 0.2 | 0.1 | 0 | 0 | 100 | 6 | 0 |
S2 | 5239 | 29.2 | 270 | 37.2 | 14000 | 9021 | 2.9 |
S3 | 400 | 16.8 | 29.8 | 6.5 | 1400 | 140 | 4.1 |
S4 | 306 | 17.0 | 30 | 3 | 1000 | 279 | 5.3 |
S5 | 2733 | 59.9 | 276 | 61.6 | 9000 | 4809 | 5.5 |
S6 | 630 | 50.7 | 77.9 | 14.6 | 2000 | 949 | 6.6 |
S7 | 64.1 | 1.2 | 12.8 | 0.8 | 300 | 32 | 10.2 |
S8 | 140 | 12.1 | 32.0 | 3.0 | 596 | 217 | 11.6 |
Source: The Authors
2.1.2 Polymers
Powder partially hydrolyzed polyacrylamides (HPAM) and HPAM polymers modified with Acrylamide-Terbutyl-Sulfonated units (ATBS) were used. Table 2 shows the polymer molecules used and some chemical characteristics.
Table 2 Polymer molecules.
Sample ID | Polymer type | Composition | Hydrolysis (%) | Molecular Weight (106 MDa) |
---|---|---|---|---|
1 | HPAM | AMD/AA | 30.0 | 5.0 |
2 | AMD/AA | 25.0 | 6.0 | |
3 | AMD/AA | 30.0 | 9.0 | |
4 | AMD/AA | 28.0 | 9.0 | |
5 | AMD/AA | 30.0 | 10.0 | |
6 | AMD/AA | 30.0 | 20.0 | |
7 | HPAM MODIFIED | AMD/AA/ATBS | 25.0 | 6.0 |
8 | AMD/AA/ATBS | 12.0 | 8.0 | |
9 | AMD/AA/ATBS | 25.0 | 8.0 | |
10 | AMD/ATBS | 25.0 | 8.0 | |
11 | AMD/ATBS | 32.0 | 8.0 | |
12 | AMD/ATBS | 25.0 | 13.0 | |
13 | AMD/AA/ATBS | 20.0 | 25.0 |
Source: The Authors
2.2 Polymeric solution preparation
Stock polymer solutions of 5,000 ppm were prepared according to API RP63 (American Petroleum Institute, 1990) [37]. The polymer powder was slowly added to the vortex formed by the preparation waters (Table 1) under stirring mechanical conditions. Stirring is maintained until complete dissolution of the polymer is achieved. The final solution used is obtained from diluting the stock solution with the preparation water at concentrations defined in Table 4.
Table 3 Porous media characteristics.
Permeability (mD) | Porosity (%) | Total Rock Composition | Less than 2 μm fraction composition | |||||
---|---|---|---|---|---|---|---|---|
Scenarios | Quartz (%) | Clays (%) | Illite (%) | Smectite (%) | Kaolinite (%) | Others (%) | ||
Low | 30 - 500 | 10-20 | 90 | 10 | 24 | 29 | 42 | 5 |
Intermediate | 500 - 1000 | 15-25 | 90 | 5 | 18 | ND* | 62 | 20 |
High | >1000 | 15-25 | 88 | 5 | <6 | ND* | 66 | 18 |
ND*: Not Detected.
Source: The Authors
Table 4 Summary of adsorption studies in porous media
Test | Polymer type | Sample ID | Fluid content | Brine | Polymer concentration (ppm) | Test temperature (°C) | Viscosity @ test temperatura (cP) | Permeability (mD) |
---|---|---|---|---|---|---|---|---|
1 | HPAM | 1 | Sw 100% | S1 | 300 | 50 | 8.6 | 184 |
2 | 5 | S1 | 300 | 50 | 9.9 | 147 | ||
3 | 3 | S2 | 500 | 60 | 5.4 | 95 | ||
4 | 6 | S3 | 1000 | 60 | 45.1 | 3710 | ||
5 | 1 | S5 | 1000 | 60 | 4.3 | 38 | ||
6 | 2 | S5 | 1000 | 60 | 3.7 | 21 | ||
7 | 4 | S5 | 1000 | 60 | 5.4 | 47 | ||
8 | MODIFIED HPAM | 9 | Sw 100% | S4 | 750 | 100 | 18.0 | 758 |
9 | 11 | S6 | 1500 | 100 | 24.7 | 3565 | ||
10 | 7 | S7 | 300 | 50 | 11.4 | 140 | ||
11 | 9 | S8 | 1000 | 90 | 40.0 | 2555 | ||
12 | 12 | S8 | 1000 | 90 | 35.8 | 2978 | ||
13 | 13 | S8 | 750 | 90 | 37.6 | 2569 | ||
14 | HPAM | 1 | Sor | S1 | 300 | 50 | 8.6 | 420 |
15 | 5 | S1 | 300 | 50 | 9.9 | 147 | ||
16 | 3 | S2 | 500 | 60 | 5.4 | 119 | ||
17 | 1 | S5 | 1000 | 60 | 4.3 | 38 | ||
18 | MODIFIED HPAM | 7 | Sor | S7 | 300 | 50 | 11.4 | 79 |
19 | 8 | S2 | 500 | 60 | 3.7 | 93 | ||
20 | 9 | S4 | 750 | 100 | 18.0 | 696 | ||
21 | 10 | S6 | 1500 | 100 | 22.6 | 3364 | ||
22 | 12 | S6 | 1000 | 100 | 35.8 | 3063 | ||
23 | 9 | S8 | 1000 | 90 | 40.0 | 4693 | ||
24 | 12 | S8 | 1000 | 90 | 35.8 | 4302 | ||
25 | 13 | S8 | 750 | 100 | 37.6 | 3179 |
Source: The Authors
2.3 Viscosity measurement
Polymer solution viscosities were measured at 30°C and at 7.3 s-1 using the Brookfield LVT viscosimeter with ULA 0 or Din 86 adapter. Each measurement was performed 4 times and the mean value of the measurements was calculated and reported on the graphs.
2.4 Porous reservoir media
Table 3 shows the petrophysical characteristics of the porous media. They are classified as low, medium, and high permeability porous media. The dimensions of the samples were 1.5 inches in diameter and 2.36 inches in length approximately. The porous media used correspond to rock samples from consolidated sandstone-type deposits characterized mainly with quartz mineralogy (90%) with variable clay mineral ratios. The high and intermediate permeability samples have lower clay content (5%), with Kaolinite being the mineral with the highest and Illite with the lowest proportions. In contrast, low permeability samples have a higher clay content (10%), and there is a large amount of Smectite-type mineral in addition to Kaolinite and Illite. Fig. 1, photographs of the pore space of the media used with low, medium, and high permeability.
Thirteen experimental evaluations were carried out with clean porous media and a single fluid in the saturation phase (100% brine, Sw 100%). Other assessments were carried out with porous media with restored wettability at residual oil saturation (Sor).
2.5 Adsorption experiments in displacement equipment
The experiments were performed in core flooding devices using an ISCO-type injection pump at a constant flow rate. The equipment has a core holder and four differential pressure sensors of different ranges to determine the pressure drop through the porous medium (Fig. 2). The core holder has a heating jacket to bring the porous media and injection fluids to the desired temperature. The temperature for the experiments was set from 50°C to 100°C.
Twenty-five displacement tests were carried out to obtain information on dynamic adsorption of HPAM and modified HPAM polymer solutions in porous media under the experimental conditions described in Table 4. In the first thirteen experiments, adsorption in porous media without restoration of wettability and a single fluid as the saturating phase (100% water).
The other twelve experiments evaluate adsorption on porous media with restored wettability. For restored wettability, the porous medium was first saturated with brine (solvent of the polymer solution to be injected in the medium). Next, the permeability brine was measured at two different injection rates. Then, crude oil was injected and the permeability at crude oil was measured and the porous medium was aged at reservoir temperature for sufficient time to establish adsorption equilibrium. Finally, the brine was injected until reaching residual oil saturation condition.
The adsorption evaluation was carried out by injecting the polymeric solution together with a tracer in the porous medium until the concentrations of polymer and tracer produced were equal to the injected concentrations. Then, the brine injection was carried out until the non-production of polymer and tracer in the effluent was evident. Finally, the second batch of polymer and tracer solution was injected until the exit concentrations were equal to those at the inlet.
2.6 Determination of adsorption
The method used to calculate polymer adsorption was the concentration profile method, which requites breakthrough curves. This method indicates that adsorption and IPV can be calculated directly with the values corresponding to the normalized concentration point (Ce / Cinj) at 0.5 [24,38,39]. Adsorption is estimated as the difference between the 0.5 normalized concentration value of the first and second polymer slug (eq. 2).
3. Results and discussion
3.1 Polymer adsorption
3.1.1 Polymer adsorption test 2 (300 ppm - HPAM polymer)
Fig. 3 shows a typical example of the raw experimental data used to estimate the reported adsorption for the second experiment. The results show that the first polymer slug is delayed and exits at 1.2 VP and the second slug is ahead and exits at 0.45 VP. HPAM adsorption was calculated with eq. (2), obtaining a value of 255.4 lb/acre-ft (41.2 μg / g of rock) was obtained. This procedure was realized for the twenty-five displacement tests carried out in the different porous media.
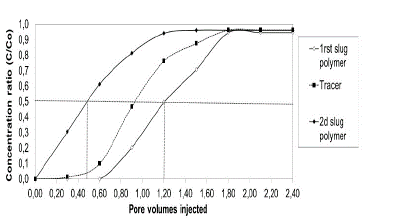
Source: The Authors
Figure 3 Example polymers adsorption in porous media saturated with brine to Sw 100%, test 2. (See details of the experiments in Table 4).
3.2 Effects of the type ofpolymer
Fig. 4 shows the adsorption results obtained for HPAM and HPAM polymers modified with sulfonated groups (ATBS) in porous media, 100% saturated with brine; Fig. 5 shows the results in porous media under residual oil saturation conditions. Results demonstrate that polymers HPAM present the highest adsorption in either saturation conditions evaluated (Sw 100% and Sor), reaching values of 1288 lb/acre-ft (224 μg/g of rock) when the superficial area of the porous media is fully exposed to the polymer (Sw 100%). This adsorption is reduced to values close to 600 lb/acre-ft (100 μg/g of rock) when the porous media carried out a wettability restoration process and is assessed under the condition of residual oil trapped in the pores. Otherwise, modified HPAM polymers show lower adsorption values (<100 lb/acre-ft or 17.5 μg/g of rock) under the most critical condition (Sw 100%). Its adsorption is further reduced in Sor scenarios with values lower than 40 lb/acre-ft (7 μg/g of rock).
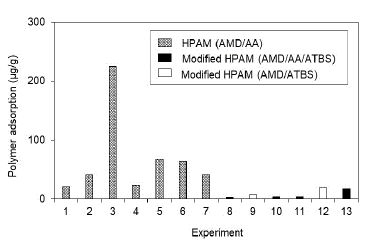
Source: The Authors
Figure 4 Polymers adsorption for different polymers in porous media saturated with brine to Sw 100% (See details of the experiments in Table 4).
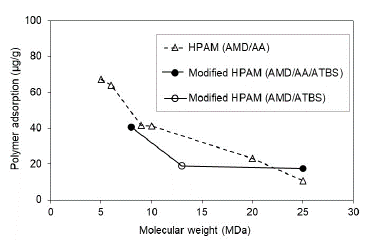
Source: The Authors
Figure 5 Polymers adsorption for different polymers in porous media with residual oil saturation (Sor). See details of the experiments in Table 4.
It is worth to highlight that the porous media used for evaluating HPAM polymers at Sw 100% and Sor are very high as compared to modified HPAM, particularly in experiment #3. This is because these experiments used low and intermediate permeability porous media, where the possible mineralogy (Illite and Smectite) and pore structure are a relevant components in the mechanical entrapment phenomenon. Moreover, the results conclude that wettability in porous media affects the polymer adsorption, and possibly the adsorption values reported in porous media without oil are not representative.
On the other hand, the experimental results show that the dynamic adsorption of HPAM polymers under Sw 100% conditions is twice the adsorption in Sor condition; this same trend was observed for modified HPAM.
Our results in the reservoir rock coincide with Szabo (1975). This study reported that modified polymers with ATBS showed lower adsorption than HPAM using Berea core samples with similar properties. The experimental data presented adsorption values ranging from 35 lb/acre-ft (6. μg/g of rock) to 72 lb/acre-ft (12 μg/g of rock) for modified HPAM polymer and between 88 lb/acre-ft (15 μg/g of rock) and 196 lb/acre-ft (34 μg/g of rock) [25].
3.3 Effects of the molecular weight in the polymer adsorption
Experimental evaluation results suggest that the adsorption hydrolyzed polyacrylamide (HPAM) decreases with the molecular weight increasing in both saturation conditions evaluated at Sw 100% (Fig. 6) and Sor (Fig. 7). Results are coherent, considering that the higher MW does not access the smallest porous because of the hydrodynamics radio and, therefore, there was less adsorption.
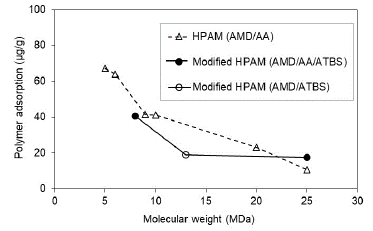
Source: The Authors
Figure 6 Polymer adsorption according to the molecular weight in porous media saturated with brine to Sw 100%.
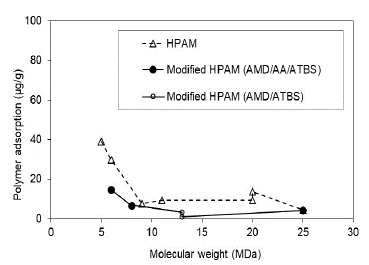
Source: The Authors
Figure 7 Polymer adsorption according to the molecular weight in porous media with residual oil saturation (Sor).
Experimentally, HPAM adsorption values evidence decreases up to 10 times with five times the increase in the molecular weight of the polymer under the condition of Sw 100% (Fig. 6). Otherwise, at Sor condition, for MW higher than 9 MDa, there is no representative change in the adsorption value (Fig. 7). These results suggest that a critical point of molecular weight exists above which the adsorption stabilizes when no mobile oil is present in the porous media. It is possible that in the smallest pores of the reservoir rock at Sor conditions, the oil is trapped due to capillary effects. There is no evidence of adsorption change in the largest pores with the increase of polymer molecular weights.
Literature reports the highest levels of dynamic adsorption as the MW of polymers increase [40-42]; this is consistent with the theory that a high molecular weight polymer could form a thicker polymer layer when the polymer is adsorbed on the surface [43].
The modified HPAM with sulfonated groups present adsorption reductions with the increase of polymer MW in both saturation conditions (Sw and Sor). However, it is less pronounced compared to the HPAM polymers. It is observed that the sulfonated group reduces adsorption values and molecular weights greater than 13 MDa. There are no significant changes in the adsorption, which could mean that it stabilizes after this molecular weight.
3.4 Effect of the polymer hydrolysis
The literature has shown that HPAM adsorption presents an inverse relation with the hydrolysis percentage, concluding that the hydrolysis degree and the solution electrolytes concentration influence more in the adsorption than the molecular weight [44]. These research experimentation results are consistent with previous results; while the HPAM polymer hydrolysis degree increase, the adsorption on the porous media in either saturation evaluated decreases (Sw 100% and Sor), where the adsorption is so much lower when residual oil is present in the porous media. This result is because a higher hydrolysis degree gives rise to greater electrostatic repulsion between the carboxyl group charge of the polymer (negative charge) and the rock surface (negative charge) and consequently decreases adsorption.
However, there is a minimum adsorption value that does not depend on the hydrolysis degree. This minimal adsorption depends on other interactions (Van der Waals and electrostatic forces, and hydrogen bonds).
The modified HPAM polymer with ATBS units is observed with the same trend of adsorption decrease according to the hydrolysis grade; however, it should be noted that modified HPAM polymers present lower adsorption than HPAM polymers in both scenarios assessed (Fig. 8 and Fig. 9). Possibly, the sulfonation grade of modified HPAM polymer increases the negatives charges in the polymer structure and, therefore, the adsorption decreased in the porous media. Szabo (1979) found a similar trend and concluded that the HPAM polymers are adsorbed more than the modified HPAM polymers [27].
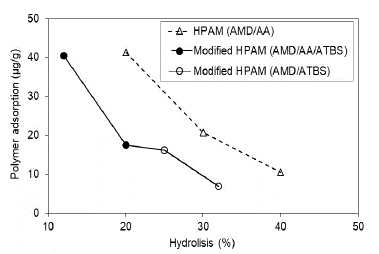
Source: The Authors
Figure 8 Polymer adsorption as a function of the polymer hydrolysis degree in porous media saturated with brine to Sw 100%.
3.5 Salinity effect on the adsorption
The increase of water salinity used for preparing the polymer increase the adsorption level. Salinity is one of the significant factors that directly affect the polymer's chemical, mechanical and thermal stability [14,16,45]. The type of charge (positive, negative, or neutral) of the rock surface changes with the salinity, causing a different behavior on the rock surface. A low concentration of Ca+2 ions promotes the adsorption of HPAM polymer on silica due to divalent ions compressing the flexibles HPAM molecules' size and reducing the static repulsion between the silica surface and the polymer's carboxyl groups [46]. Additionally, Smith's results showed that the dynamic adsorption of polymer could be six times higher with a change in the water salinity used for preparing the polymer solution from 1% to 10% NaCl.
The modified polymers with a high sulfonation degree decrease the adsorption in with high salinity and content of divalent ions scenarios[44]. However, the efficiency of these polymers depends mainly on their manufacturing process. Similarly, a polymer manufactured through a post-hydrolysis process is more sensitive to divalent ions content than a polymer obtained through copolymerization.
This research shows that HPAM polymer and modified HPAM polymer adsorption is directly related to the polymer's preparation water salinity for both conditions, Sw 100% (Fig. 10) and Sor (Fig. 11) being lower for the state of residual oil saturation. The adsorption values are reduced up to 5.5 times when used modified HPAM polymer with sulfonated groups in the more critical scenario (Sw 100%) and approximately 18 times in the residual oil saturation scenario at the highest salinity condition of 14000 ppm. In terms of salinity, trends of modified polymers corroborate that polymers with sulfonated groups (AtBS) are resistant in adverse high salinity conditions in some reservoirs, and possibly, these molecules aid the polymer flooding processes in high salinity reservoirs.
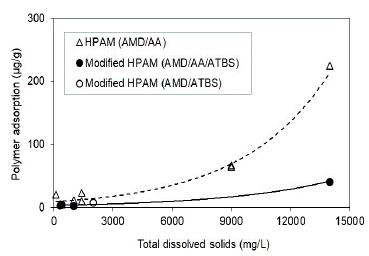
Source: The Authors
Figure 10 Polymer adsorption as a function of the salinity in porous media saturated with brine to Sw 100%.
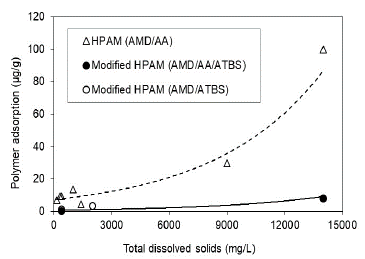
Source: The Authors
Figure 11 Polymer adsorption as a function of the salinity in the porous media with residual oil saturation (Sor).
With respect to water hardness, just as for the salinity, it is evidenced that the adsorption increases when increasing the polymer's preparation water hardness (Fig. 12). HPAM polymers with hardness values below 6% have lower adsorption than the modified polymers with sulfonated groups, possibly caused by the modified polymers' manufacturing process. In spite of their chemical and thermal resistance, they continue having operation limits for reservoirs with high salinity, hardness, and temperature. Additionally, the adsorption results below 6% of hardness show quite low adsorption values for both molecule type assessed. It may be concluded that, below this hardness value, the adsorption in sandstones is less than 7 μg/g of rock.
3.6 Permeability effect
Polymer retention and adsorption are reduced according to permeability increase due to the decrease of phenomena such as the polymer mechanic entrapment on the smaller pore throat. Another variable that has a significant influence on polymer retention is the clay content. Therefore, rock samples with low permeability and high content clay raise the retention values.
In this research work, the rock samples are sandstone with Kaolinite as the major clay mineral. The results are consistent with those reported in the literature (for both scenarios assessed), whereas there is higher permeability and, lower polymer adsorption. In the case of Sw 100% (Fig. 13), higher adsorption values were obtained due to higher surface contact of the polymer solution with the porous media.
4. Conclusions
This research compared the results of dynamic adsorption of HPAM polymers and modified HPAM polymers according to molecular weight, hydrolysis degree, salinity, water hardness, and permeability in two different saturation scenarios: Sw 100% and Sor. Based on the analysis of the results, it may be concluded that:
The chemical structure of the polymers has a significant influence on the dynamic adsorption; polymers with modified structure (ATBS) show adsorption values below than 10 μg/g at low permeability and Sor conditions.
It is a common practice in the industry to perform adsorption tests with 100 % water saturation. However, the results show that this is not a representative condition for oil reservoirs. Polymer adsorptions strongly depend on the saturation condition of porous media.
The HPAM and the modified HPAM polymer's molecular weight presents an inverse relationship with the dynamic adsorption. The polymers with higher MW should face constrained access in the porous media.
When the hydrolysis degree of the HPAM polymers increases, the adsorption goes down; the adsorption is also low when the porous media have a residual oil saturation. This behavior can be explained because higher polymer hydrolysis can generate a more significant electrostatic repulsion between the carboxyl group's charge (negative charge) and the rock surface (negative charge). However, there is a minimum adsorption value that doesn't depend on the hydrolysis degree.
Modified HPAM polymers with ATBS units show the same tendency that HPAM polymers, although with lower adsorption values. The sulfonation degree of modified HPAM increases negative charges in the polymer structure, decreasing the adsorption on the porous media.
The salinity and hardness of water show a direct relationship with adsorption for the two types of molecules (HPAM and modified HPAM), but modified HPAM's are more resistant to high salinity and hardness conditions. Modified HPAM evidence 50% less adsorption at the same conditions compared with HPAM.
The increase of the porous medias's permeability reduces the HPAM and modified HPAM adsorption.
The dynamic adsorption of HPAM polymers under Sw 100% conditions is twice the adsorption under Sor condition; modified HPAM show the same behavior.