1. Introduction
Worldwide, sweet potato (Ipomoea potatoes L.) is a traditional and nutrient-rich staple food due to the high content of vitamins, minerals, dietary fibers, and various bioactive compounds, including anthocyanins, flavonoids, and carotenoids. Despite its wide production, especially in subtropical and temperate regions [20], the technical knowledge on this crop is still limited, mainly regarding root handling procedure and postharvest storage.
Handling and storage procedures are critical to the maintenance of sweet potato roots overall quality. These practices are performed to reduce physiological disorders and diseases, ensuring by the end a continuum food root supply throughout the year [5]. One of the main problems associated with an inadequate storage condition is a higher loss of fresh weight, due to increased transpiration, as the temperature above 15 °C induces sprouting incidence, accompanied by several biochemical changes in metabolism, such as increased activity of antioxidant enzymes, changes in the plasma membrane, production of reactive oxygen species and physiological disturbance caused by the significant increase in reducing sugars glucose and fructose (sweetening induced by cold).
Cold storage is effective in maintaining the postharvest quality of vegetables by reducing the overall metabolism, resulting in decreased both water loss and respiratory activity, besides the development of diseases during storage [16]. However, temperatures below 10 °C are associated with chilling injury, leading to the deterioration of sweet potato roots [19].
One of the strategies to reduce the effects of chilling injury is the use of the low-temperature conditioning (LTC) technique, which is defined by the storage at mild temperatures before being submitted to lower temperatures. Under the effect of LTC, plant tissues are induced to increase cold tolerance before placed at below critical temperatures [14]. In fruits such as mango, pear, and peach, LTC's effectiveness in reducing cold injury symptoms has been demonstrated elsewhere [21]. However, the effects of LTC on vegetable products, such as sweet potatoes and especially on Brazilian cultivars, remain little explored.
Therefore, this study aimed to evaluate the ability of LTC in mitigating symptoms of chilling injury in the BRS Cuia and BRS Rubissol cultivars.
2. Material and methods
2.1. Plant material and planting
Sweet potato seedlings of cvs. BRS Rubissol and BRS Cuia were purchased from the Frutplan LTDA company and cultivated on ridges (1.0 x 0.4 m) at the Experimental Facilities from Department of Crop Science, Universidade Federal de Viçosa (UFV), Viçosa, Minas Gerais, Brazil (20o 45' 20'' S and 42° 52' 40'' W, 651 m altitude), by September 2017 to February 2018 timeframe, namely as spring planting.
2.2 Healing process
The harvest was performed at 130 d after planting, when the roots weighing about 300 to 600 g - free of diseases and damage - were selected and transported in plastic boxes to the Postharvest Physiology Laboratory, UFV. At the laboratory, the roots were submitted to the curing process according to described by [1], by storing them under 30 °C and relative humidity 90%, for 7 d in a manufactured BOD incubator (Thermolab Scientific Equipments).
2.3 Experimental design
After the curing period, roots were submitted to storage at 13 °C (control), or 6 °C, as well as low-temperature conditioning (previous storage at 10 °C for 7 d, followed by storage at 6 °C) in a cold room (GALLANT CMR2). The experiment was performed with four replications containing two roots each. For each treatment, samples were collected at 0, 15, 30, 45, and 60 d of storage.
2.4 Estimation of the Cold Injury Index (CI)
Symptoms of chilling injury (CI) were characterized based on the appearance of either superficial pitting or internal tissue browning, or both, as described by [19] and [13]. The CI index was evaluated in six roots for each replication, where scores ranging from 0 to 4 were attributed. 0, as the absence of injuries; 1, as very mild injury; 2, as minor injuries; 3, as moderate injuries and, 4, as serious injuries. The CI index was calculated by using Eq. 1.
A represents the obtained rating (0-4); B represents the number of roots at the level; T represents the number of roots evaluated in the treatment. The CI index regarding each treatment was presented as the mean ± standard deviation of four repetitions.
2.5 Determination of Membrane Permeability and Malondialdehyde Content (MDA)
The membrane permeability was determined by using electrolyte leakage (EL) assessment, adapted from [15]. Discs from sweet potato pulp were previously washed and incubated in 20 mL of deionized water at room temperature. The initial electrical conductivity (L1) of the suspension liquid was measured in a conductivity meter (Digimed, model DM-31) after incubation for 6 h; while the final electrical conductivity (L2) was obtained after placing the flasks containing the discs in an oven at 90 °C for 2 h. The membrane permeability was calculated as EL% = (L1/L2) x 100.
Lipid peroxidation was determined by quantifying levels of malondialdehyde (MDA), according to [10]. The content of MDA was expressed in Limol kg-1 FM based on the extinction coefficient of 155 mM-1 cm-1 of fresh matter (FM).
2.6 Proline concentration (PRO)
Proline content was assessed as described by [3], in which 0.3 g of root tissues were macerated in liquid N2 and homogenized (POLYTRON® - model PT 2500 E) in 10 mL of sulfosalicylic acid. The remained supernatant reacted with 3% glacial acetic acid and ninhydrin acid at 95 °C for 1 h, then cooled and mixed with an equal volume of toluene. The toluene layer was removed and the absorbance at 520 nm was measured based on a standard curve with L-proline. The proline content was expressed as mol kg-1 FM.
2.7 Extraction and determination of the content ofphenolic compounds (PHEN)
The total soluble phenolics were extracted from 5 g of fresh pulp homogenized (POLYTRON® - model PT 2500 E) in 80% ethanol. The total phenolic content was quantified with a Foolin-Ciocalteu reagent as previously reported by [8], with gallic acid as a standard solution. The reading was made on a spectrophotometer (BEL ENGINEERING® - model UV-Vis 200-1000NM) at 760 nm, and the content of phenolics expressed as mg 100 g-1 FM.
2.8 Enzymatic assays of catalase, peroxidase, ascorbate peroxidase and phenylalanine ammonium lyase
The enzymatic extract was prepared with the homogenate of 0.2 g pulp in 2 mL of extraction buffer containing 100 mM potassium phosphate (pH 7.0), 0.1 mM EDTA, 1 mM phenylmethylsulfonyl fluoride (PMSF) and 1% (w/v) polyvinylpyrrolidone (PVP). Subsequently, the homogenate was centrifuged at 14,000 g in centrifuge (NOVATECNICA - model NT806) for 15 min at 4 °C, and the supernatant fraction was used to determine the activities of catalase, ascorbate peroxidase, and peroxidase.
Catalase activity (CAT) was determined as described by [9]. The reaction buffer was composed of 50 mM potassium phosphate (pH 7.0) and 12.5 mM H2O2. The reaction started with the addition of enzyme extract. The activity was determined by decreasing the absorbance at 240 nm for 1 min. CAT activity was expressed as mmol s-1 kg-1 of protein, based on the extinction coefficient of 36 μM-1 m-1.
The activity of ascorbate peroxidase (APX) was quantified according to [17] method. The reaction medium containing 50 mM potassium phosphate (pH 7.8), 0.25 mM of 0.3 mM ascorbic acid, and H2O2 was used. The activity was assessed by reducing the absorbance at 290 nm for 1 min.
APX activity was expressed as mmol s-1 kg-1 protein, using the molar extinction coefficient of 2.8 μM-1 m-1.
Peroxidase activity (POD) was accomplished by using [12] method. The reaction buffer contained 25 mM potassium phosphate (pH 6.5), 20 mM guaiacol, and 20 mM H2O2. Enzymatic activity was measured by increasing the absorbance at 470 nm. It was expressed as mmol s-1 kg-1 protein, by using the molar extinction coefficient of 26.6 μM- m-1
The activity of phenylalanine ammonium lyase (PAL) was evaluated as previously described by [4] in which approximately 0.2 g of pulp was homogenized (POLYTRON® - model PT 2500 E) in an extraction medium containing 50 mM borate buffer (pH 8.5), 10 mM 2 -mercaptoethanol and 1% PVP (w/v). The homogenate was centrifuged at 14000 g for 15 min at 4 °C. The reaction medium was composed of 100 mM potassium phosphate buffer (pH 8.5) and 100 mM phenylalanine. Afterward, the enzyme extract was incubated at 40 °C for 40 min. The reaction was stopped with 5 N HCl. The samples were read at 290 nm using a trans-cinnamic acid as a curve standard. PAL activity was expressed as mmol s-1 kg-1 protein.
The experiment was conducted in a completely randomized design, arranged in a split-plot scheme, with a 2 x 3 factorial in the plot: two cultivars of sweet potato (BRS Rubissol and BRS Cuia) and three storage conditions (13 °C, control; 6 °C and LTC (root maintenance at 10 °C for 7 d followed by storage at 6 °C); and five storage periods (0, 15, 30, 45 and 60 days) in the subplot. Overall, five repetitions per treatment were used by considering three root as an experimental unit.
2.9 Statistical analysis
The data were submitted to analysis of variance and the means were compared by the Tukey test, at 5% probability by using the SAS software [6]. Descriptive statistics of means with standard deviation were also used. The graphs were plotted by Sigma Plot 10.0. software. Pearson's correlations between variables were performed with R 'corrplot' package [23]. The statistical design was a completely randomized distribution.
3. Results and discussion
Storage at both 6 °C and LTC led to the occurrence of chilling injury symptoms (Fig. 1). Such responses were accompanied by the incidence of surface pitting, especially in cv. BRS Cuia. Otherwise, cv. BRS Rubissol did not present any incidence of the external lesion during storage (Fig. 1), indicating that this cultivar is likely more tolerant to chilling injury than its counterpart. One of the hypotheses for the appearance of external lesions in the cv. BRS Cuia is that the chilling injury has triggered cell oxidative stresses, leading to the development of symptoms associated with increased lipid peroxidation. Therefore, since the increase in both lipid peroxidation and electrolyte leakage indicate disintegration of cell membranes, the tissue browning observed in the present study is closely related to chilling injury [22,2].
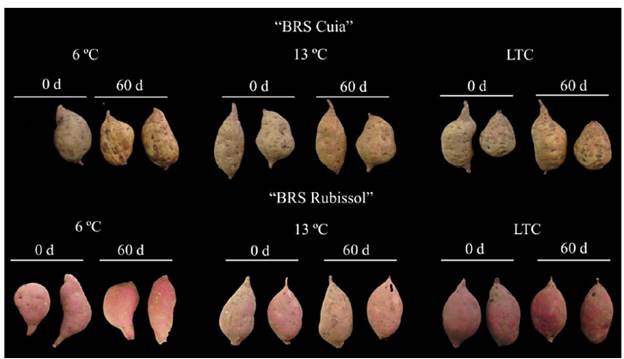
Source: The autors.
Figure 1 External appearance of sweet potato roots cv. BRS Cuia and BRS Rubissol stored at 6, 13 °C (control), and LTC (10 °C for 7 d, followed by storage at 6 °C).
Regarding internal injuries, storage at 6 °C led to the incidence of tissue browning after 30 d of storage in both sweet potato cultivars, thereby causing a reduction in their overall quality. Storage at 13 °C and LTC did not provoke internal tissue browning. In sweet potato roots, internal lesions are symptoms that reflect membrane damage induced by lipid peroxidation. Moreover, internal tissue browning was likely caused by either synthesis of chlorogenic acid or oxidation of other phenolic compounds, commonly correlated with such responses in sweet potato roots [19,12].
These results corroborate with those observed by [12], who found that sweet potato Yanshu 25 stored at 4 °C showed substantial morphological changes, including internal tissue browning after 8 d of storage. [24] also found that sweet potato roots cv. Xushu 18 stored at 4 °C for 5 weeks showed internal tissue browning. Otherwise, at 14 °C, sweet potato roots were not affected.
As expected, the storage at 13 °C did not lead to chilling injury in sweet potato roots of both cultivars. The sweet potato roots cv. BRS Cuia stored at 6 °C showed symptoms of chilling injury (Figs. 1 and 2) after 30 d, reaching an index 3.37 times higher than that observed in LTC roots at the end of the storage period (Fig. 3A).
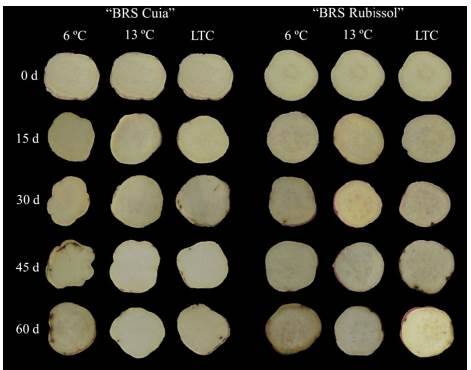
Source: The autors.
Figure 2 Internal appearance of sweet potato roots cv. BRS Cuia and BRS Rubissol stored at 6, 13 °C (control), and LTC (10 °C for 7 d, followed by storage at 6 °C).
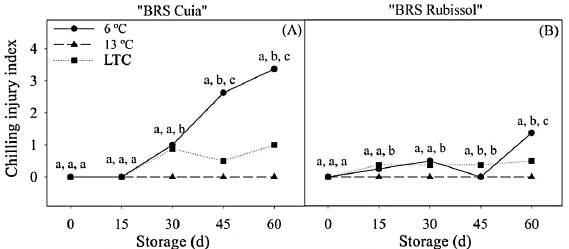
Source: The autors.
Figure 3 Chilling injury index in sweet potato BRS Cuia (A) and BRS Rubissol (B) cultivars stored at 6, 13 °C (control), and LTC (10 °C for 7 d, followed by storage at 6 °C). The data represent the mean ± standard deviation (n=5). Lower case letters indicate differences between temperatures in each storage period, respectively, based on the Tukey test at 5% probability.
The cv. BRS Rubissol exhibited slight internal tissue-browning (Fig. 2), with a lower CI index as compared to cv. BRS Cuia. In roots stored at 6 °C, CI index slightly increased up to 30 d, with a subsequent decrease by 45 d, followed by a marked increase at the end of the storage period, which was 2.74 times higher than observed in LTC roots (Fig. 3B). In addition, the conditioned roots showed a CI index lower than observed in the 6 °C roots, indicating that the conditioning can alleviate chilling injury occurrence.
These data are in agreement with previous results reported by [13], in which sweet potato roots cv. 'Xinxiang' stored at 4 °C and LTC showed symptoms of chilling injury associated with a higher CI index. However, roots stored at 6 °C presented a CI index 2.4 times higher than observed in LTC roots. [18] also observed that the roots of cv. 'Longshu No. 9' stored at 5 °C developed superficial pitting and the CI index quickly increased with the progress of storage time.
For cv. BRS Cuia, it was observed an increase in the percentage of electrolyte leakage (from 9.5 to 46.0%) in roots stored at 6 °C; while from 9.50 to 45.83% in LTC roots at the end of the storage period. The roots stored at 13 °C had the slightest increase, changing from 9.50% to 19.27% at the end of storage, also presenting a lower level of electrolyte leakage (Fig. 4A). In BRS Rubissol, the highest percentages of electrolyte leakage were observed in both LTC and 6 °C roots, with percentages of 41.56% and 38.45%, respectively, at the end of the storage period (Fig. 4B).
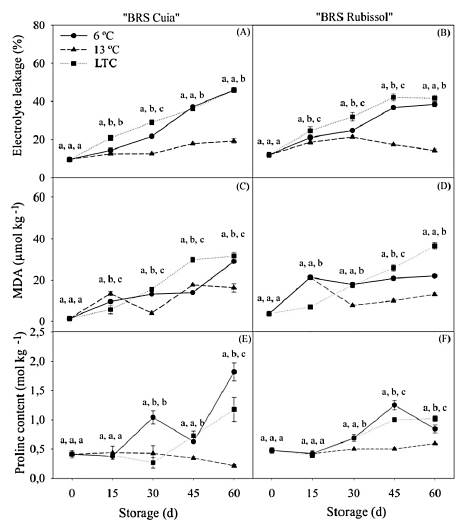
Source: The autors.
Figure 4 Electrolyte leakage, malonaldehyde (MDA) and proline content in sweet potato roots cv. BRS Cuia (A, C, and E) and BRS Rubissol (B, D, and F) stored at 6, 13 ° C (control) and LTC (10 ° C for 7 d, followed by storage at 6 ° C). The data represent the mean ± standard deviation (n = 5). Lower case letters indicate differences between temperatures in each storage period, based on the Tukey test at 5% probability.
The MDA content was higher in BRS Cuia at the end of the storage period, with 30.70 and 29.07 μmol kg-1 in both LTC and 6 °C roots, respectively. Otherwise, storage at 13 °C led to the lowest value, namely 16.27 μmol kg-1 (Fig. 4C). This behavior was also observed in BRS Rubissol at the same storage period, which obtained 30.57 μmol kg-1 in the LTC roots and 21.96 μmol kg-1 in roots stored at 6 °C (Fig. 4D).
Electrolyte leakage and MDA content are the main indicators of oxidative stress, degree of cell membrane stability and consequently chilling injury [13;18]. These results suggest that sweet potato roots stored at both 6 °C and LTC were affected by low temperatures, leading to the incidence of chilling injury and excessive generation of reactive oxygen species (ROS), thereby causing lipid peroxidation, leakage of electrolytes, and MDA accumulation [13].
[13] also observed that both electrolyte leakage and MDA content quickly increase in sweet potato roots cv. 'Xinxiang' when stored at both 4 and 10 °C for 5 d. [22] observed similar behavior when sweet potato roots were stored at 6 °C, when also presented a higher MDA content.
For proline levels, it was observed that roots of cv. BRS Cuia stored at 6 °C presented values 1.55 and 8.65 times higher than observed in LTC and 13 °C roots, respectively, at the end of the storage period (Fig. 4E). For cv. BRS Rubissol, there was no difference until 15 d, however, storage at 6 °C and LTC led to a higher concentration of proline after 45 d, exceeding 2.55 and 2.05 times the value observed in the roots at 13 °C, respectively (Fig. 4F). Increases in proline levels of sweet potato roots stored at low temperatures were also observed by [22], who verified a gradual increase in such compound in both Xushu 32 and Yanshu 25 cultivars after storage at 4 °C. Otherwise, at 16 °C, the values were lower than its counterpart, as observed in the present study for 13 °C.
Storage at low temperatures leads to biochemical shifts in sweet potatoes, in a manner that may respond in many different ways. One of the most common responses includes the accumulation of low molecular weight nitrogen compounds, such as proline, and the activation of ROS scavenge enzyme system. At a steady state, proline keeps reactive oxygen species (ROS's) at low levels, as they are osmotic regulators, playing an important role in protecting against injuries caused by cold. Proline acts as a cellular osmolyte on the stabilization of membrane, subcellular structures, protection of cells against oxidation, and on the stabilization of ROS's scavenging enzymes, thereby protecting membrane integrity [7].
The content of total soluble phenolics increased in cv. BRS Cuia during storage (Fig. 5A). The roots at 6 °C showed values 1.51 and 1.35 times higher than observed in LTC and 13 °C roots, respectively, at 45 days. However, at the end of the storage period, the total soluble phenolic content was 1.17 and 1.32 times higher in LTC roots than in roots at both 6 and 13 °C, respectively. For cv. BRS Rubissol, there was a gradual growth, in which the roots submitted to LTC exhibited the highest total soluble phenolic contents at both 45 and 60 d, with values 1.40 and 1.94 times higher than in 6 °C e 13 °C roots, respectively (Figura 5B).
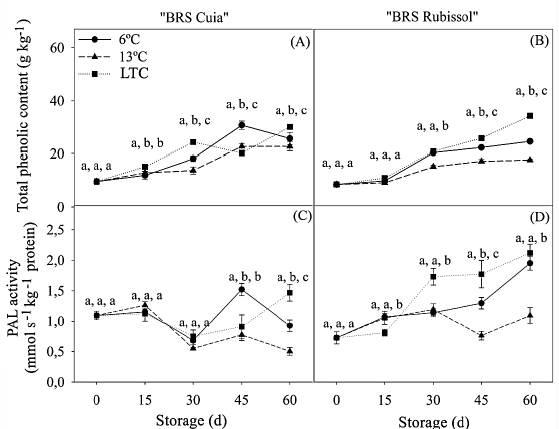
Source: The autors.
Figure 6 Total phenolic content and phenylalanine ammonium lyase (PAL) activity in sweet potato roots cv. BRS Cuia (A and C) and BRS Rubissol (B and D) stored at 6, 13 °C (control), and LTC (10 °C for 7 days, followed by storage at 6 °C). The data represent the mean ± standard deviation (n = 5). Lower case letters indicate differences between temperatures in each storage period, respectively, based on the Tukey test at 5% probability.
Increases in total soluble phenolic content were also observed by [22] in sweet potato roots cv. Xushu 32 and Yanshu 25 at 4 °C. At 16 °C, otherwise, the values were lower than those observed in the present study. [11] also found that the highest content of total phenolics was evidenced in sweet potato roots at 4 °C, reaching 2.56 mg g-1 after 4 weeks of storage. However, sweet potato roots at 13 °C showed the lowest levels (2.12 mg/g) by considering the same period of storage.
At 15 and 30 d of storage, PAL activity did not differ among the treatments for cv. BRS Cuia, but at 45 d it was markedly induced at 6 °C, reaching thus a maximum activity with values 1.69 and 1.98 times higher than that observed in LTC e 13 °C roots, respectively. On the other hand, at 60 days, the PAL activity in roots conditioned at 10 °C was significantly higher than that observed in 6 °C roots (Fig. 5C). In cv. BRS Rubissol, PAL activity was higher in LTC roots at 30 and 45 d. However, at the end of the storage period, the PAL activity in roots at 6 °C did not differ from each other, but the value was 1.95 times higher than observed in 13 °C roots (Fig. 5D).
It is well-known that cold stress may accelerate phenolic metabolism and increase PAL activity [24,22]. As demonstrated in the present study, the highest content of total soluble phenolics and the highest PAL activity were observed in LTC and 6 °C roots, for both sweet potato cultivars (Fig. 6). Total soluble phenolics play a crucial role in plants, as they are mechanisms of defense and scavenge of oxidizing molecules. The previous study has also shown that the amount of phenolics in sweet potatoes influences the natural chemical defense against cold stress [11].
Chilling injury provokes many biochemical changes in vegetables, including increased PAL activity, which may play a vital role in alleviating such negative responses [11,24]. The results observed in the present study corroborate with those found by [22] in sweet potato roots cv. Xushu 32 at 4 °C after 8 d of storage. The authors demonstrated that the PAL activity in cv. Xushu 32 was 1.5 times higher than observed in cv. Yanshu 25 (tolerant cultivar).
The CAT activity in BRS Cuia cultivar varied as a function of temperatures. However, storage at 6 °C led to a higher activity after 60 d as compared to the other temperatures (Fig. 7A). The cv. BRS Rubissol showed similar behavior, with the highest CAT activity occurring in both LTC and 6 °C roots after 60 d, respectively (Fig. 7B).
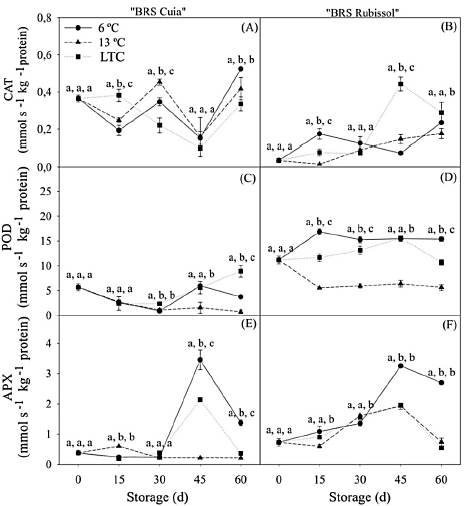
Source: The autors.
Figure 7 Catalase, peroxidase, and ascorbate peroxidase activity in sweet potato roots cv. BRS Cuia (A, C, and E) and BRS Rubissol (B, D, and F) stored at 6, 13 °C (control) and LTC (10 °C for 7 days, followed by storage at 6 °C). The data represent the mean ± standard deviation (n = 5). Lower case letters indicate differences between temperatures in each storage period, respectively, based on the Tukey test at 5% probability.
In cv. BRS Cuia, POD activity did not differ among evaluated treatments until 15 d. Nevertheless, at 30 and 45 d, the activity increased in both LTC and 6 °C roots. At the end of the period of storage, LTC led to an increase in POD activity, with values 12.38 and 2.39 higher than those observed in 13 and 6 °C roots (Fig. 7C). The cv. BRS Rubissol showed an increase in POD activity after 15 d in LTC and 6 °C roots; however, at 45 d, the activity did not differ among treatments. At the end of the storage period, the activity was 1.69 and 6.22 times higher in the roots at 6 °C, as compared to LTC and 13 °C stored roots, respectively. In roots maintained at 13 °C, the POD activity decreased at the beginning of storage and remained stable over after (Fig. 7D).
The roots of cv. BRS Cuia stored both at 6 °C and LTC showed an increase in APX activity after 45 d of storage, with subsequent reduction at the end of the storage period. While roots stored at 6 °C presented the highest activity, the roots stored at 13 °C kept low the APX activity during storage (Fig. 7E). BRS Rubissol showed higher activity when stored at 6 °C after 45 d, with values 5 and 3.7 times higher than observed in LTC and 13 °C roots, respectively (Fig. 7F).
The first response to chilling injury involves cellular and membrane structure modification as a consequence of changes in the composition of structural lipids. In this study, sweet potato roots at 6 °C and LTC showed higher electrolyte leakage and MDA content, thus corroborating with the hypothesis that low temperatures affect components of the cell membrane, namely lipids. These changes may lead to an increase and accumulation in ROS's levels, promoting oxidative stress, which may trigger enzymatic and non-enzymatic antioxidant defense systems to alleviate negative consequences.
The antioxidant enzymes showed higher activity in roots stored at both 6 °C and LTC (Fig. 7). These results suggest that the antioxidant defense mechanism was activated through catalase (CAT), peroxidase (POD), and ascorbate peroxidase (APX) activities.
The increase in CAT, POD, and APX activities was also observed in sweet potato roots cv. Xushu 32 after four d of storage at 4 °C [22], as observed in the present study. Such CAT activity, which varied during the period of storage, was also observed by [18] in sweet potato roots cv. "Longshu No.9".
Although the antioxidant defense system has been demonstrated as efficient in dealing with oxidative stress and chilling injury, oxidative stress can still occur during storage at low temperatures due to the gradual depletion of ROS-scavenging enzymes. In the present study, the activity of POD in cv. BRS Cuia at 6 °C (Fig. 7C) and cv. BRS Rubissol at the LTC (Fig. 7D) was reduced at the end of the storage period. The same occurred for APX, in which the activity was reduced in roots of cv. BRS Cuia (Fig. 7E) and BRS Rubissol (Fig. 7F) after 60 d at both 6 °C and LTC, which suggests a depletion of ROS scavenging-related enzymes. This corroborates with the results reported by [22], who also found that the activity of APX and CAT decreased dramatically at the end of storage of sweet potatoes at 4 °C.
Pearson's correlation coefficients are represented in the heatmap (Fig. 8). For cv. BRS Cuia, CAT activity was negatively correlated with the most variables, but showed a positive correlation with PROL (r = 0.52; P = 1%); the MDA content was positively correlated with EL (r = 0.80; P = 0.01%), PHEN (r = 0.78; P = 0.01%), PROL (r = 0.65; P = 5%). In turn, the EL had a positive correlation with PHEN (r = 0.85; P = 0.01%), PROL (r = 0.79; P = 0.01%) and CI (r = 0.57 ; P = 0.01%). POD was also positively correlated with PAL (r = 0.99; P = 0.01%). For cv. BRS Rubissol, there was no negative correlation between the variables, however, the PHEN had highly positive correlations, with EL (r = 0.87; P = 0.01%); the same was verified for EL, which correlated positively with PROL (r = 0.70; P = 0.01%).
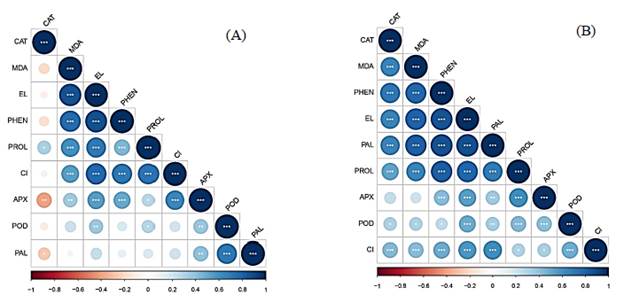
Source: The autors.
Figure 8 Pearson's correlation heatmap regarding electrolyte leakage (EL), malonialdehyde content (MDA), proline level (PROL), total phenolic compounds content (PHEN), catalase activity (CAT), peroxidase (POD), ascorbate peroxidase (APX), phenylalanine ammonium lyase (PAL) and chilling injury index (CI) in cvs. of sweet potatoes BRS Cuia (A) and BRS Rubissol (B). The colors of the bars indicate the nature of the correlation, where 1 consists of a perfectly positive correlation (dark blue) and -1 mean a perfectly negative correlation (dark red). Strong correlations are indicated by large circles, while weak correlations are indicated by small circles. Asterisks within the circle are P-values. (***, **, and * indicate significance at 0.01, 1 and 5%, respectively).
4. Conclusion
LTC has induced chilling injury tolerance in studied sweet potato roots on cv. BRS Rubissol by the activation of the antioxidant system, which was associated with a higher content of total soluble phenolics, PAL activity, and proline levels. Moreover, LTC roots showed no external or internal injuries, maintaining the broad quality of potato roots for further processing. Overall, such results indicate that the LTC is effective in mitigating chilling injury in Brazilians sweet potato cultivars.