Introduction
Methionine (Met) is the second or third most limiting amino acid (AA) in conventional diets for growing pigs (NRC, 1998; Chen et al., 2014). This sulfur-containing AA plays a role in methylation processes, intestinal oxidative status, maintenance of intestinal mucosal growth and gut function, and is involved in the biosynthesis of creatine, epinephrine, carnitine, taurine, lecithin, choline, melatonin (Bauchart- Thevret et al., 2009; Tesseraud et al., 2009) and cysteine (NRC, 2012). Therefore, a Met deficiency could reduce its availability for protein synthesis and limit animal growth.
Cysteine (Cys) is included in the structure of many proteins, linking polypeptide chains as a precursor of taurine and glutathione (Baker, 1991). Most Cys must be obtained from dietary Met since many feed ingredients lack of adequate Cys levels (Santos et al., 2011). Thus, Met should be added into pig diets to meet the methionine+cysteine (Met+Cys) complex requirement. If the Met level in the diet is insufficient, Cys requirements may not be fulfilled.
According to some reports, low dietary Met+Cys levels improve or have no effect on the productive performance and carcass characteristics of pigs (Santos et al., 2011; Ying et al., 2015. Methionine deficient diets could enhance adipose tissue lipid metabolism, decrease oxidative damage, alter antioxidant pathways, and alleviate the inflammatory responses in growing pigs (Ying et al., 2015; Zhou et al., 2016). Therefore, low levels of Met+Cys could improve growth performance (Santos et al., 2011; Ying et al., 2015; Zhou et al., 2016). Nevertheless, Conde-Aguilera et al. (2010, 2014) observed that reduced Met+Cys levels in pig diets increased fat deposition, reduced protein synthesis and affected carcass characteristics, while Met supplementation seems to improve productive performance (Opapeju et al., 2012; Chen et al., 2014; de Oliveira et al., 2015) when the sulfur-containing AA requirements are met, in addition to producing extra benefits from Met acting as a functional AA (Wu et al., 2013). Nevertheless, an excess of Met+Cys can also affect growth performance and carcass characteristics (Santos et al., 2007; Pena et al., 2008).
The appropriate dietary concentration of Met+Cys has not been established because the optimal level of AA depends on the characteristic to be optimized (Zhang et al., 2013). Therefore, the objective of this study was to determine a multi-objective optimal level of Met+Cys in growing pig diets regarding growth performance and carcass characteristics.
Materials and Methods
Ethical considerations
The experimental procedures followed the recommendations of the Council for International Organizations of Medical Sciences (CIOMS, 2012) and followed the standards for ethics, biosafety and animal well-being of the Official Mexican Standard (Norma Oficial Mexicana; NOM-062-ZOO, 1999) for the use of experimental animals.
Location
The experiment was conducted in the Swine Unit at the Experimental Farm of Colegio de Postgraduados, located in Montecillo, State of Mexico (98º 48' 27" W and 19º 48' 23" N). The climate is temperate, semi-arid, with 15.9 °C average annual temperature, infrequent frosts, 686 mm average annual rainfall, and 2,250 m of altitude (García, 1988).
Animals and experimental design
Ninety-nine (Yorkshire×Landrace×Duroc) hybrid growing barrows were used in a completely randomized design, with 25.90±3.99 kg average body weight (BWi). Nine Met+Cys levels (0.500, 0.525, 0.550, 0.575, 0.600, 0.625, 0.650, 0.675, and 0.700%) were evaluated during 35 days. These levels were obtained by adding synthetic methionine to the control diet.
Diets and animal handling
Diets were formulated based on sorghum-soy- bean meal, supplemented with crystalline amino acids, and formulated with digestible amino acids (NRC, 2012) to meet or exceed the nutritional requirements for this stage of growth (Table 1). Pigs were individually housed in 1.2×1.5 m pens with concrete floors, equipped with a single feeder and a nipple drinker. Feed and water were provided ad libitum.
Table 1 Composition of experimental diets for growing pigs (25-50 kg) fed different Methionine+Cysteine levels.
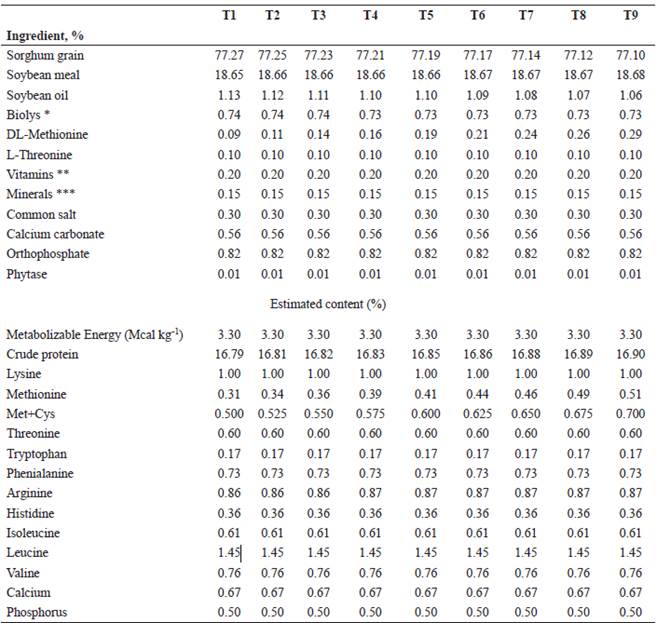
T, Treatment. *Biolys, 50.7%; lysine. **Supplied by kg of feed: 5.0×106 IU vitamin A; 1.0×106 IU vitamin D3; 2.0×104 IU vitamin E; 2 g vitamin K3; 1 g thiamine; 5 g riboflavin; 2 g pyridoxine; 25 g niacin; 15 g D-calcium pantothenate; 3 g folic acid; 225 g choline chloride; 0.3 g antioxidant; 15 mg vitamin B12; 180 mg vitamin H-biotin (REKA® Lapisa Animal Nutrition). *** Supplied by kg of feed: 0.2 g Se; 0.1 g Co; 0.3 g I; 10 g Cu; 100 g Zn; 100 g Fe; 100 g Mn (REKA® Lapisa Animal Nutrition).
Variables measured
The following variables were analyzed: Average daily gain (ADG), average daily feed intake (ADFI), and feed: gain ratio (FGR). On the first and last days of experimentation, backfat thickness (BT) and longissimus muscle area (LMA) were measured using a real- time ultrasound Sonovet 600 with a 3.5 MHz transducer (Medison, Inc., Cypress, California, USA). These data, along with the initial and final body weights, were used to determine the fat-free lean gain (FFLG) and the lean meat percentage (LMP) using the NPPC (Burson and Berg, 2001) equation.
Statistical analysis
The animals were assigned to one of the nine treatments, using a completely randomized design with nine Met+Cys levels. Each pig was considered an experimental unit. The Shapiro-Wilk and Levene's tests were used to check for normal distribution and variance homogeneity assumptions for the analysis of variance. Analysis of variance was performed with the GLM procedure (MINITAB, 2007) to estimate regression coefficients, which included linear and/or quadratic effects, using the p-value (p≤0.05), and the coefficient of determination (R2) as criteria to determine the best model. Initial body weight (BWi) was used as a covariate.
To obtain the optimal concentrations of Met, a response surface model was built using the RSREG command (MINITAB, 2007), with an optimization technique based on factorial planning to obtain the Met+Cys levels for the different productive and carcass characteristics. Then, maximization of ADG, ADFI, and minimization of FGR were considered to achieve the optimal multi-objective level of the variables affected by the Met+Cys level in the diet. The regression model was adjusted for each variable using the adjusted treatments means by BWi. Subsequently, the Response Optimizer was used to identify the combined configuration values of the input variables to optimize the overall response and determine the individual and composite desirability of the multiple response system (MINITAB, 2007).
Results
Growth performance variables are presented in Table 2. The ADFI and FGR showed a linear response to the Met+Cys levels in the diet (p<0.05). The optimal concentration of Met+Cys was estimated at 0.500% to maximize ADFI, and 0.700% to minimize ADFI (ADFI=1.68 - 1.01*Met+Cys + 0.032*BWi; R2=0.45). The optimal concentration of Met+Cys to minimize FGR was 0.700% (FGR=1.94 - 0.27*Met+Cys + 0.05*BWi; R2=0.41). The ADG did not show any effect (p>0.05) of the dietary level of Met+Cys. However, to determine the optimum biological level of Met+Cys with multi-objective optimization we thought necessary to consider that ADG showed a quadratic tendency (p=0.14) because ADG has an important interrelationship with ADFI and FGR, besides, it improves the adjustment of the model. The optimum biological level of Met+Cys that optimized the multi-objective solution was 0.677%. The FFLG, LMP, BT, and LMA were not affected (p>0.05) by the dietary concentration of Met+Cys.
Discussion
Methionine requirements can be altered by the protein level of the diet, feeding system, growth potential of the animals, interrelationships with other limiting amino acids, health status of the animal (Rakhshandeh et al., 2014) and interaction with other sulfur-containing amino acids such as cysteine since part of the supplemented Met is converted to Cys (NRC, 2012). Thus, the addition of Met into diets is an alternative to meet the requirements of the Met+Cys complex. Digestible Met+Cys requirements were established based on a minimum of 50% of sulfur-containing amino acids being supplied by Met (Rostagno et al., 2011).
There is no agreement on the digestible Met+Cys requirements of pigs. In the present experiment, we found that the optimal level of Met+Cys for ADFI and FGR was 0.70%; however, when considering the multi- objective optimal level, it was 0.677%. At this concentration, the best FGR is obtained with a lower ADFI, without affecting ADG. According to the NRC (1998, 2012), the requirements for digestible Met+Cys for growing pigs (20- 50 kg) are estimated to be 0.47 and 0.55%, respectively, whereas the Brazilian tables (Rostagno et al., 2017) have established their requirements based on productive performance (standard and high production) at 0.566 and 0.631%, respectively.
Table 2 Treatment means for the productive performance of growing pigs fed with different levels of methionine+cysteine (Met+Cys).
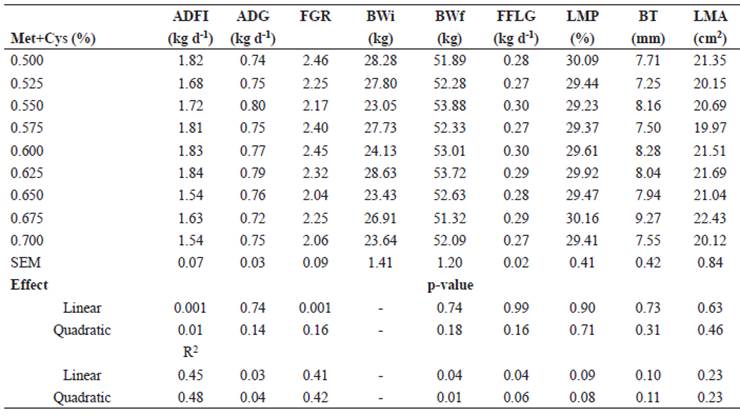
ADFI, average daily feed intake; ADG, average daily gain; FGR, feed:gain ratio; BWi, initial body weight; BWf, final body weight; FFLG, fat-free lean gain; LMP, lean meat percentage; BT, backfat thickness; LMA, longissimus muscle area; SEM, standard error of the mean.
The optimal Met+Cys requirement in our study was higher than that recommended by the NRC (1998, 2012), and very close to the one determined by Rostagno et al. (2017). A higher level could lead to extra benefits in the productive performance of the animal. Oral supplementation of Met at 0.02, 0.04, and 0.06% in growing pigs (20 kg), improves daily gain, feed intake, and feed: gain ratio, regardless of whether or not there was a deficiency (Opapeju et al., 2012). Other studies evaluating various dietary Met levels have shown better feed conversion and daily protein deposition in pigs as Met concentrations increase (de Oliveira et al., 2015). It is also reported that an extra 0.12% of Met supplementation increased ADG and improved feed efficiency during post-weaning (Chen et al., 2014). All these benefits are probably due to the functional role of dietary Met for intestinal growth and function, in addition to its role as a precursor for protein synthesis (Wu et al., 2013).
Our study confirms that reduction of Met+Cys in growing pig diets affects productive variables, as protein synthesis and accretion are affected by insufficient Met levels. Conde- Aguilera et al. (2010) found that pigs fed a Met deficient diet had lower body weight gain than pigs fed a control diet. Similarly, Kiefer et al. (2005) observed that productive performance and carcass characteristics had a quadratic effect in response to the Met+Cys levels (0.44-0.61%) in the diets for growing pigs, with the best level between 0.527 and 0.551%. Met and Cys deficiency have been observed to significantly suppress intestinal mucosal growth, reduced intestinal epithelial cell proliferation, and increased intestinal oxidative stress in piglets (Bauchart-Thevret et al., 2009; Chen et al., 2014). Methionine deficiency alters lipid metabolism, decreases oxidative damage, alters antioxidant pathways, and alleviates inflammatory responses in growing pigs (Ying et al., 2015; Zhou et al., 2016). Lower Met+Cys levels in pig diets, compared with NRC (1998) requirements, increase lipid content in subcutaneous adipose tissue and Longissimus dorsi muscle (Conde- Aguilera et al., 2014; Castellano et al., 2015). Pigs fed a Met deficient diet have a higher lipid composition than pigs fed a basal diet (Batterham et al., 1993; Martinez-Ramirez et al., 2008; Conde-Aguilera et al., 2014). In addition, pigs fed a Met-restricted diet had increased triglyceride content in subcutaneous adipose tissue with unchanged plasma triglyceride contents (Castellano et al., 2015).
Theoretically, a Met deficiency could affect the amounts of Cys and glutathione, consequently increasing oxidative stress, causing damage to the synthesis of important cellular components (fat, protein, DNA; Metayer et al., 2008). Oxidative stress may result in poor performance, disturbed health, and even death (Zheng et al., 2013). When pigs show a stressed-activated immune system, Met requirements are higher since Cys synthesis from Met tends to increase (Li et al., 2014; de Oliveira et al., 2015). A recent study (Conde-Aguilera et al., 2016) suggests that insufficient intake of sulfur AA during early growth may reduce glutathione content in the muscle of growing pigs. Moreover, Met supplementation (0.12%) is required for optimal protein synthesis, so, it could increase cysteine and glutathione concentrations in the plasma and tissues, leading to reductions in plasma and tissue redox potential and the maintenance of mucosal integrity in the small intestine of weaned piglets (Chen et al., 2014).
Some studies have shown that excess of Met+Cys can affect growth performance, carcass weight, amount of meat, backfat thickness and daily meat deposition in finishing pigs (Santos et al., 2007; Pena et al., 2008). Recent studies reported that dietary Met restriction in pig diets improved or did not influence growth performance and carcass characteristics (Santos et al., 2011; Ying et al., 2015). In contrast, Caldara et al. (2003) did not find differences in productive variables when using 0.63 to 0.74% Met+Cys. In addition, Castellano et al. (2015) observed that a dietary Met deficiency resulted in increased lipid content in subcutaneous adipose tissue and Longissimus dorsi muscle, which was associated with an increase in enzyme activity and expression levels of critical genes involved in de novo lipogenesis in these tissues. The activity of antioxidant enzymes increased in adipose and muscle tissues and altered the cellular redox status of young pigs fed a Met- deficient diet. Total antioxidant capacity in the plasma and glutathione levels in the liver were lower in pigs fed Met-deficient diets. These authors (Castellano et al., 2015) concluded that tissue metabolism adapts differently when facing an insufficient supply of dietary Met.
In conclusion, the optimal level of Met+Cys is 0.677% in growing pig diets to obtain the best feed:gain ratio, average daily gain, and daily feed intake. Our results indicate that below 0.667% Met+Cys daily feed intake increases to compensate for the lower intake of Met+Cys.