Introduction
Biofuels are regarded as one of the most viable options for reduction of CO2 emissions in the transport sector 1. Human beings have been using biofuels since pre-historical times. Wood and vegetable oil were the most common fuels before the 19th century, when they started been replaced by fossil fuel. Fossil fuels that attend over 80% of the world’s energetic needs today. However, their reserves are limited and are being depleted at alarming rates. Global warming, high oil prices, and new investments have been leading to the search of renewable and sustainable fuels. In spite of that, the so called 1st generation fuels can get in the way of feeding people. But the lignocellulosic residues generated in industrial processes can be applied to produce 2nd generation fuels that reduce solid residues along with solving the energy problem. Besides being promising we still have a long way to go before this new processes are economically viable 2), (3), (4), (5.
One of the most researched biofuels has been ethanol; it can be produced from multiple raw materials, such as, wood, agro-industrial residues, municipal sewage, paper industry effluents, and different grasses. The potential use of these materials is based on their large availability and low cost 3), (6. The biomass productions costs in Brazil are considered the lowest in the world, with great possibilities of promising results 7.
Orange is vastly consumed all around the world, for its flavor as well as its medicinal and nutritional properties.
Oranges are being processed as orange juice, therefore, generating large amounts of waste that can be easily collected from a single site, making it a promising biomass source. About 50% of processed orange is estimated to be waste. Brazil is the world leader of orange production, with 35% of the world’s production. The orange bagasse contains approximately 16% of hemicellulose, 28% of cellulose and 9% lignin, showing to be a viable alternative to produce second generation ethanol. In order to obtain ethanol from this residues it is necessary to hydrolyze the polysaccharides into fermentable sugars, for posterior fermentation 8), (9), (10. The presence of lignin in lignocellulosic biomass makes it resistant to enzymatic attack to release sugars. Pretreatment is an important step in bioethanol production in order to achieve maximum saccharification efficiency with high fermentable sugar yields 11. Pretreatment is a fundamental step for refining biofuels and biobased products from the lignocellulosic biomass as the bioconversion of cellulose into fermentable monosaccharides 12. Alkaline pretreatment, a kind of pretreatment method, possesses some desirable features. It mainly removes lignin and can be carried out at milder conditions than several other pretreatments, like hydrothermal pretreatments, etc. 13. Calcium hydroxide (Ca(OH)2) is a possible reagent to be used for alkaline pretreatment 14. The most impacting unitary operation is hydrolysis, and it can be either enzymatic or acid. The acid hydrolysis can be either dilute or concentrated. For the dilute process high temperatures are needed, and that can generate undesired compounds. Also, with concentrated acids there can be difficulties in the process, such as corrosion, and hydrolysate neutralizing costs. Depending on the conditions the acid hydrolyses can remove the lignin and part of the hemicellulose opening pores that give access to the cellulose and spare the pre-treatment phase. Though, but in order to break the cellulose high temperatures or acid concentrations are needed. While, the extreme conditions can generate inhibitory co-factors, and degrade the sugars to other compounds reducing the hydrolysis efficiency. Before fermenting it is necessary to neutralize the hydrolysate generating salts that can’t be separated and can either act as supplement or inhibitor to the yeast 3), (15), (16. Lignocellulosic biomass from vegetable waste has a great potential for use in the production of bioethanol. Although, due to its complex structure, it requires pretreatment to improve the yield of reducing sugars in the hydrolysate during enzymatic hydrolysis from cellulose and hemicellulose 17. For the enzymatic hydrolysis it is mandatory pre-treating the biomass to make the cellulose accessible to the enzymes. Most studies about the lignocellulosic ethanol production involve enzymes, besides that the hydrolysate volumes are insufficient to the following fermentation processes. Even with the reduction in the enzymes cost in the last 20 years, they still represent around 50% of the second generation ethanol cost, that has a very superior cost than first generation ethanol 2),(15. The aim with the present work was to study the feasibility of obtaining total reducing sugars from orange peels. For this, two types of hydrolysis, dilute acid and enzymatic, were evaluated. For acid hydrolysis, it was studied the application of milder temperatures, to reduce energy costs as well as the generation of inhibitory compounds. For enzymatic hydrolysis, a combination of cellulase and xylanase was studied, in order to increase the production of reducing sugars.
Materials and methods
Biomass
The orange peels were kindly donated by a juice processing plant (Gyn Fruit in Goiânia GO Brazil). They were then cut into smaller pieces and kilndried at 50°C until constant weight. The dried peels were crushed in Wiley mill, and the fines, diameter under 0.3 m, were discarded for interfering in the pre-treatment.
Biomass Characterization
The hemicellulose and cellulose content were determined by the method described by Browning 11. The ash and moisture contents were determined by the Instituto Adolfo Lutz 19 methods. The lignin was quantified using procedure preconized by IUPAC 20.
Pre-treatment
The pre-treatment was done using calcium hydroxide, biomass and distilled water (1:4:20 w/w/v) at 60°C for 72000s and 150 rpm 9. After the pre-treatment the biomass was washed with 10 times the reaction volume with distilled water and dried at 40°C until constant weight. After drying, the biomass was stored in plastic vials at room temperature away from the light.
Enzymatic activities
To maximize the total reducing sugars (TRS) production two types of enzymes were used, cellulase (Sigma-Aldrich) and xylanase (Granotec) both from Trichoderma reesei. The choice to combine this enzymes was due to the evidence of their synergic effect on the generation of sugars 14), (15), (16) (17.
The cellulase activity was determined using the paper filter method, considering the effect of pH and temperature in the activity, the results were expressed in FPU (Filter Paper Units)/10-6. m3 (14), (25. The activity of the xylanase was determined using the procedure of the University of New South Wales (Kensington, Australia), considering the effect of temperature and pH in the activity, and the results expressed in U/Kg 19.
Hydrolyses central composite design
Factorial planning was used in order to evaluate the dilute acid and enzymatic hydrolyses. The tests were planned using a factorial planning of central composite type at two levels, with three variables (2³ assays), added of three replicas in the central point, to investigate a linear model and also 6 experiments in the axial (α) points, bringing the total to 17 experiments. 27.
The dilute acid hydrolysis conditions for the codified and uncodified factors (acid concentration, time and temperature) are presented in Table 1. The factors are according Dussán et al. 15. The hydrolysis was done using 5.10-5 m3 of chloridric acid (HCl) solution in every concentration, and what was left after the pre-treatment of 2∙10-3 Kg of biomass in 125∙10-6 m3 Erlenmeyer flasks.
The enzymatic hydrolysis was carried out to evaluate the impact of the enzymes concentration and time of hydrolysis. The solids (cellulose and hemicellulose) were 1%. The buffer was sodium acetate, and the rotation was 150 rpm. The temperature and pH were defined by the enzymatic activities tests and constants have been kept for all experiments. Coded and non-codified values are illustrated in the development matrix provided in Table 2. The codified alfa levels were 1.68179. In order to measure the amount of total reducing sugars (TRS), after the hydrolyses, 1∙10-6m3 of the filtered was measured and put in an assay tube, then 2∙10-6m3 of dinitrosalicylic acid reactant (DNS) were added. A blank tube was done using 1∙10-6m3 of deionized water and 2∙10-6m3 of DNS. The tubes were taken to a thermostatic bath at 95°C for 300 seconds followed by an ice bath to stop the reaction. The volume was completed to 25∙10-6m3 and the absorbance was read in spectrophotometer at the wave length of 540 nm 28.
Results and discussion
Biomass Characterization
After being dried and milled the orange bagasse was sieved and the particles under 0.295.103 m were discarded. The dry bagasse moisture content was 7.38%±0.03%, and the ashes content was 3.79%±0.04%. The cellulose contents were 22.9%±0.41%. The literature shows values that are similar to this 10), (29), (30.
The amount of hemicellulose was 3.39%±0.38%, value much smaller than the researched references for orange bagasse 29), (30. The lignin content was larger than the found for orange bagasse by other researchers 29), (30. The composition of vegetal material may vary from different regions, soil composition, and time of the year it was harvested 7.
Enzymatic Activities
The cellulase activity was 24.08∙10-6FPU/m3 the optimum pH was 4.8 and the optimum temperature was 60°C. For the xylanase the activity was 199.58∙10-3 U/Kg, the optimum pH was 5.2, but by a 3.4% difference to 4.8, and the optimum temperature was 50°C. The pH of work was set at 4.8, and the temperature at 45°C.
Hydrolyses Central Composite Design
Statistical experimental design is one of the more efficient and easier methods to arrange and interpret 31. The results obtained in the CCD showed that there was a good reproducibility between the central points, statistically equal results, evidencing the quality of the repeatability of the process. The highest response was obtained in experiment 12, performed at the highest temperature used in the experiment (+ α). When comparing this experiment with one of the central points there is a nearly 50% increase in the value of the response. It is believed in the first instance that the temperature variable has a strong influence on the release of ART after the acid hydrolysis. It is observed a similarity of response between experiments 3 and 4, then it can be verified that when working with the lower level of acid concentration, higher temperatures and increase of time between the lower and upper levels the response does not change. With this, it can be inferred that the best responses combine high temperatures and high acid concentrations, and the time being less relevant.The average mass loss from the pre-treatment was 30.03%, less than the reported by Silva et al. 9, 58.43%. For the dilute acid hydrolysis the TRS levels varied from (9.32±0.68)∙10-3 Kg TRS per Kg of biomass to (30.15±0.31)∙10-3 Kg TRS per Kg of biomass. All the results are presented in Table 3.
The multiple regressions of the variables were calculated with the software Statistica ™ 7.0 and the significant terms (p≤0.1) are presented in Table 4.
The reduced equation with a determination coefficient R² of 96% was:
Where: TRS = Total Reducing Sugars; C = HCl Concentration; T = Temperature and t = Time
Response Surface Methodology (RSM) is successfully used for developing efficient bioprocesses. RSM is a statistical technique used for experiment design, model building, estimating the effect of input variables and predicting optimum conditions 32. From the reduced equation the response surfaces were constructed for each pair of independent variables in function of the response variable TRS (Figure 1).
The Figure 1a showed that higher temperature levels led to higher concentrations of ART combined with HCl concentrations at their lower levels. When the time and the concentration of HCl are compared, (Fig. 1b) it is observed that from intermediate concentrations of acid, a greater release of ART occurs, while there is no substantial interference of the time, which can be verified in the value of the coefficient of the time variable in Equation 1. Similar analysis can be verified by evaluating the relation between time and temperature, where the higher temperature levels, caused elevations in the ART levels, while the time was not significant for the increase of sugar concentration (Fig. 1c).
From the data obtained it is inferred that low temperatures of dilute acid hydrolysis lead to a partial hydrolysis of the lignocellulosic compounds, that is, a greater liberation of reducing sugars occurs at temperatures higher than those studied in this work. However, when comparing with the literature, it is seen that very high temperatures favor the degradation of the formed sugars, reducing the yield of the process 15), (16), (33).
The Figure shows that the temperature was the most significant factor for the generation of TRS, with time being the least significant. The researched literature shows that with different biomasses and higher temperatures (135°C - 175°C) the most impacting factor tends to be the acid concentration 15. The difference may be due to the fact that in this work the optimum conditions were not found, and too high temperatures may degrade the sugars, reducing the hydrolysis’ output 33.
The study of enzymatic hydrolysis after pretreatment with calcium hydroxide was performed with the enzymes cellulase and xylanase at pH 4.8 and 150 rpm at 1% solids. Through statistical planning the concentration of TRS achieved in the enzymatic hydrolysis varied from around 75.10-6 Kg TRS per 10-3 Kg of biomass to almost 100.10-6 Kg/ 10-3Kg (Table 5). It is possible to observe that the results of total reducing sugars released by the enzymatic hydrolysis were about 3 times higher than those of the diluted acid hydrolysis. This result may indicate that, although the optimal acid hydrolysis conditions have not been found, enzymatic hydrolysis is more efficient for the generation of sugars from the orange bagasse.
It can be observed from Table 5 that the highest concentrations of ART occurred in experiments 5, 6, 8 and 10, corresponding to the higher levels of cellulase concentration (+1 and +α) and variable levels of xylanase concentration and temperature. The lowest values of ART were in experiments 2 and 9 that have in common the lower levels of cellulase, -1 and -α respectively. It can be observed that the concentration of xylanase practically did not interfere in the release of ART, which can be observed when comparing experiments 1 and 3 that with equal levels of cellulase and time and different levels of xylanase show practically the same concentration of ART.
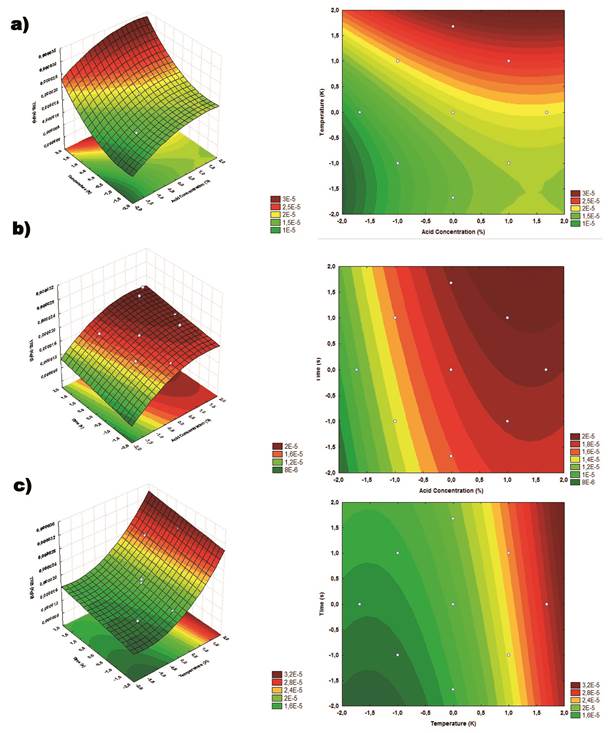
Figure 1.Response Surfaces and Contour Plots of the TRS (Kg.g-1): (a) HCl Concentration (C) vs. Temperature (T); (b) HCl Concentration (C) vs. Time (t); (c) Temperature (T) vs. Time (t)
The multiple regressions of the variables was all the terms were significant (p≤0.1) and are calculated with the software Statistica ™ 7.0, presented in Table 6.
The whole equation, with a determination coefficient R² of 98.3% was:
Where: TRS = Total Reducing Sugars, C = Cellulase Concentration, X = Xylanase Concentration t = Time.
With the whole equation the response surfaces were constructed for each pair of independent variables in function of the response variable TRS (Figure 2).
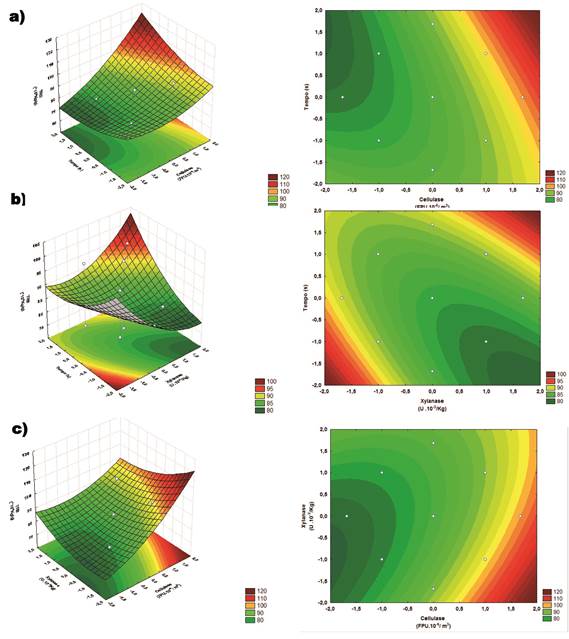
Figure 2 Response Surfaces and Contour Plots of the TRS (mg g-1): (a) Cellulase Concentration (C) vs. Time (t); (b) Xylanase Concentration (X) vs. Time (t); (c) Cellulase Concentration (C) vs. Xylanase Concentration (X)
The results demonstrate the relationship between enzyme concentrations in the release of total reducing sugars, the highest values were observed in the highest concentrations of cellulase (7.02∙10- 6 FPU/m3) and lower concentrations of xylanase (0.00∙10-3 U/Kg), with the cellulase concentration being more significant. When comparing the effect of time and cellulase concentration on the concentration of total reducing sugars, it was observed that the higher levels of the two variables (40 h and 7.02 ∙10-6 FPU/m3) led to higher concentrations of reducing sugars. In the time relationship with the xylanase concentration, two maximum regions were found for the liberation of total reducing sugars, one in the combination of the lowest levels (0.0∙10-3 U/Kg and 18.54 h) and the other in the combination of the highest levels (5.00∙10-3 U/Kg and 45.45 h)
The most significant factor for the generation of TRS was the concentration of cellulose, the other factors, besides being significant, were very much less impacting. Many authors have reported synergistic effects on the hydrolysis of sugars by the combination of cellulase and xylanase, for different substrates 21), (22), (23), (24. However, the results obtained in this work indicate the opposite, the best results were at the highest levels of cellulase and lowest of xylanase. When comparing the time and cellulase effect on the generation of sugars the best results were at the combination of the highest levels of both, which is in accordance with the literature 21), (22).
Conclusions
The temperatures and acid concentrations were insufficient to totally hydrolyze the sugars in the biomass, requiring further investigations with higher levels of both. The enzymatic hydrolysis yielded higher amounts of total reducing sugars (TRS) than the acid hydrolysis, indicating it could be more advantageous for further studies. The xylanase concentration did not impact the enzymatic hydrolysis of the orange bagasse. The studied conditions of time and cellulase concentration were not enough to totally hydrolyze the substrate, also requiring further studies at different conditions.