Introduction
It is estimated that the soils of the world store 1500 Gt of carbon (C) at 100 cm depth and that, approximately, half of this amount is found in 0- 30 cm topsoil (Batjes, 1996). In comparison, the carbon reserves in biomass are of the order of 500 Gt (IPCC, 2001; Denman, Brasseur, & Chidthaisong, 2007).
Considering the size and location of these reserves, a greater understanding of their composition and dynamic is required, not only at the level of the major bioclimatic regions of the world, but also for the local ecosystems on which the effects of global warming will be more evident.
From this, two general considerations must be taken into account: if the reduction of these stocks as a consequence of anthropogenic activities, continues, significant quantities of CO2 and other Greenhouse Gases (GG) will be transferred into the atmosphere. This would increase the concentration to critical levels which significantly alter the physical and biotic processes of the planet (IPCC, 2000; Canadell et al., 2007; Ritchter & Houghton, 2011).
On the other hand, the implementation of local and regional mitigating strategies, such as reforestation, conservation agriculture and the rehabilitation of soils present a high potential contribution to the preservation of these stocks and the capture of a proportion of atmospheric C (Guo & Gifford, 2002; Lal, 2004; Smith, Martino, & Cai, 2007). The incorporation of C in forest soils depends, in the first instance, on the contribution of organic residues to the surface layer and the transformation and stabilization of a fraction of these residues through different biogeochemical mechanisms (Smith, 2008).
However, when the natural recycling of the forest is altered through changes in their use, these contributions decrease and, in the extreme case of their conversion to agricultural soil, practically disappear. Similarly, the physical alteration of the soil through the removal of vegetal cover or farmland predisposes it to the breaking of its internal structure, exposing to mineralization the fractions of soil organic matter, encapsulated and protected by the mineral fraction, facilitating their loss until a new state of temporary equilibrium is reached (Guo & Gifford, 2002; Lal, 2005; Van der Werf, Morton, & DeFries, (2009).
Lal (2009), estimated the tropical and subtropical agricultural soils have lost among 60 and 80 % of their C reserves, which translates into the atmosphere to the order of 136+/- 55 Gt.
In the case of soils converted to agricultural use, a large part of carbon loss is considered to have taken place during the first years of cultivation (Watson et al., 2000; Smith, 2002). Initially, the C content decreases with the mineralization of the soluble fractions (carbohydrates and simple proteins). Subsequently, as tillage intensify, C content decreases with the gradual loss of more stable fractions contained in humic substances ( Alvarez-Arteaga, Krasilnikov, & García-Calderón, 2012). With these, C tends to be reduced by 25 to 30 %, until reaching the next level of temporary stability, which occurs independent to the soil type, management practices, and decomposition processes (Murty, Kirschbaum, Mcmurtrie, & Mcgilvray, 2002; Houghton 2004).
After agriculture, the conversion of forest into induced pasture (IP) is the condition of change in soil use which releases the second largest amount of carbon into the atmosphere in Mexico, with a little more than 6 % of total emissions. However, some evidence suggests that this change does not always act in this way, with some cases having shown increases post conversion, principally when the forest soil presents a low C content (Guo & Gifford, 2002).
The main difference among pasture and agricultural soil is that, in the latter, the constant alteration of the surface layer facilitates the mineralization of the labile soil organic carbon (LSOC), with the loss expected to be more intense. In contrast, when a crop field is abandoned, population of creeping and herbaceous plants will gradually increase the carbon reserves in the soil depending on the intensity of previous management, or even due to the different biophysical factors present in the soil (Murty et al., 2002).
Considering the importance of the study of SOC and distribution of soil carbon stocks as a starting point for the implementation of local actions to mitigate GG emissions in both natural and productive ecosystems, the aim of this research was to estimate the organic carbon stocks in volcanic soils (humic andosols), under different management practices and times of soil use. The research also seeks to determine the influence of soil use change on the bulk density of the soil, its acidity and, finally, its potential for preserving SOC.
Materials and methods
Study plots
The study was undertaken in soil plots in the municipality of San José del Rincon, State of Mexico, located among 19º 40´38.8" and 19º 42´11.8" North and 100º 13´5.6" and 100º 12´54.8" East, in the central region of the Trans Mexican Volcanic Belt (Figure 1).
The region performs an average annual temperature oscillating among 8 and 12°C and an average annual rainfall among 1000 and 1200 mm, with a marked seasonal variation between June and October.
The underlying geological material dates from the middle and late Miocene, and is characterized by extrusive igneous rock, specifically andesite and rhyolite. The dominant topographical system comprises low ridges of round hills and basalt plateaus. Slope varies among 10 and 30 %. The region is covered by plant associations of Abies religiosa (oyamel), Pinus pseudostrobus (pino), Cupressus lindheyi (cedro), and Quercus rugosa (encino).
Similar to other forest regions of the highlands on Central México, the region featured in this research, historically is subject to an accelerated process of disturbance and soil use change, characterized by fires, clandestine tree felling, controlled timber extraction and the exploitation of soils for agriculture and livestock.
Specifically, the plots under forest cover are subject to forest harvesting throughout a management plan, while soils under agricultural and IP use have a history of use with different ages.
Selection of sampling points and soil sampling
With the aim of including natural and productive systems and subsystems more representative of the evaluated area, a delimitation of the current soil uses was undertaken. Three conditions of soil use (forestry, induced pasture and agriculture) were obtained, as were eleven variants, all of which were found in soils originating from volcanic ash (humic andosols) among 2912 and 3060 m. a. s. l., four of these variants corresponded to forestry use (AF, CF, QF, PF) according with dominant species, four to seasonal agriculture (SA1, SA2, SA3, SA4), and three to induced pasture (IP1, IP+SA, IP2) (Table 1).
Soil samples were taken in each subsystem at 20 cm top soil in the central part of a representative polygon, with an average surface of 1500 m2, using a zigzag sampling scheme, thus generating a composite soil sample for each plot.
The samples were bagged and ticketed to be then transferred to the laboratory, where they were dried at room temperature and passed throughout a 2 mm mesh sieve.
An approximate portion of 5g of soil was ground and passed through a 0.25mm sieve for the total organic carbon analysis (TOC), using the Walkley and Black method. Dry and wet color analyses were undertaken using Munsell tables, while the pH (ratio 1:2.5 in H2O and KCl), and bulk density (BD) were taken using the cylinder method.
The estimation of the soil carbon stocks (Mg C ha-1), was carried out taking into account depth, bulk density, and SOC content. To determine possible significantly statistical differences among the different conditions for the soil use and management and soil carbon stocks, some variables were undertaken using a one factor ANOVA test which, in the event of significantly results, was complemented with post hoc Tukey (p= 0.05) tests.
Results and discussion
The results for the soil properties analyzed and significantly differences found, are shown in Table 2.
Table 2 Estimated parameters for the calculation of the soil organic carbon stocks at the different study plots at 20 cm top soil
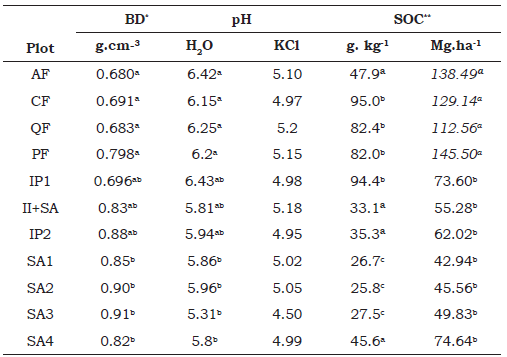
*BD= Bulk Density; **SOC = Soil Organic Carbon
† Value taken at a 20 cm top soil
Average values with different letters in the same column, differing significantly amonst themselves (P < 0.05)
Different studies have reported the implications of the soil use changes on several soil properties, such as the organic material content, bulk density, porosity and acidity.
Habitually, under similar formation conditions, forest soil performs BD lower than those for other soil uses, while these tend to increase with practices such as mechanized cultivation or grazing owing to the loss of the original structure and the compacting of the soil particles (Pulido-Moncada, Helwig-Penning, Timm, Gabriels, & Cornelis, 2014).
The BD was observed as greater in 22 and 12 % of the soil use for agriculture and induced pasture in this research, respectively, compared to the value obtained for the forest soils, with the only significantly difference found among soil use for agriculture and forestry. Such variations are consistent with those presented by Murty et al., (2002), who compared data from 78 case studies, observing average increases of 17 % for agricultural soils and 9.5% for livestock, with the extreme cases showing an increase of above 60%.
This variation translates in the reduction or loss of the original structure of the soil, caused by machine tilling or trampling by livestock. According to other cases, such as those presented by Gou and Gifford (2002), and Houghton (2004), it is probable the condition of the slope and the zero vegetation coverage in these soils present during one part of the year, can reduce the infiltration capacity and increase the levels of soil runoff, generating soil degradation conditions.
Table 2, shows an acidification gradient for the soil caused by the intensification of the soil use going from forestry to IP or agriculture. Statistically, this difference becomes significantly among soils used for forestry and agriculture, more than soils used for IP and the two remaining uses.
The previous finding could be considered to be related to leaching and the loss of interchangeable bases, including interchangeable aluminum (Al3+), which is abundant in soils originating in volcanic ash and contributes to the acidification of the soil, above all when its buffer capacity decreases by the reduction in SOC (Bernier & Alfaro, 2006)
The correlation among the SOC and BD (Figure 2), is significantly and negative, denoting with higher BD, decrease the soil carbon content.
Given these concerns, consistent with the effect of soil tillage on the destruction of aggregates and the release of organic components, which become more susceptible to attack and the mineralization of microorganisms in the soil or their loss throughout leaching (Unger, 2001).
The highest concentration of SOC is found in forest soils with an average value of 76.8 +/- 20.2 g C kg soil-1. It should be noted, the management conditions for these forest systems were considered as a referent, and there are no primary forests in the region. However, the average value differs significantly to those for agricultural soils (SA2, SA3, and SA4), with 3.3 +/- 1.9 g C kg soil-1 and those managed under induced pasture (IP1, IPI+SA and IP2) with 54.3 +/- 34.8 g C kg soil-1.
In the case of forest soils, management practices have allowed to favor a controlled felling and preservation of the organic layer which lies over the mineral soil, facilitating the nutrient recycling and preservation of the soil properties, such as high porosity, low bulk density, and slight levels of acidification.
Carbon stocks
In soils under agriculture and induced pasture, a lowest level of C accumulation was measured at 30 cm top soil (expressed in Mg C ha-1), compared to the original condition of forestry use (Figure 3).
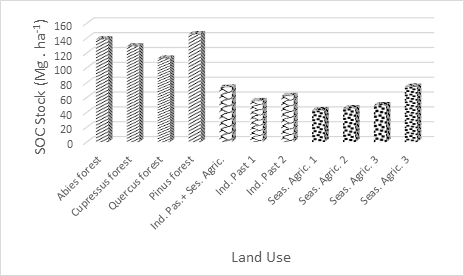
Figure 3 Soil Organic Carbon stocks in different soil uses in the municipality of San José del Rincon, Mexico
The C stored in the forestry, SA and IP soils was 131.42 +/- 14.25, 53.24 +/- 14.54 and 63.63 +/- 9.27 Mg C. ha-1, respectively. While the differences among SA and IP use, were not revealed any significantly difference.
The highest level of variability was recorded in agricultural soils, particularly for plot AT4. While this plot is currently subject to intensive management practices with the rotation of corn and potato crops, this management history only dates back 30 years, in contrast to the other agricultural soil plots with more than 100 years.
The foregoing tends to corroborate the assumption the tillage timing is another determining factor in the loss of carbon stocks, and which corresponds to the references consulted in this research. In IP soils, the C stock performs intermediate values compared to those previous soil uses, a finding mainly attributed to the contribution of grass root biomass to the soil, based on the assumption of a greater incorporation of organic material and an increase in the C stocks, once the agricultural soils is converted to IP.
One management alternative proposed for these regions, is the broadening of mixed production systems, not only to increase the carbon reserves in the soil throughout crop rotation and incorporation of perennial vegetation, but also to improve and even increase the long-term capacity of these soils to perform their distinct functions.
While the C content is significantly higher for forest soils, the current management conditions for the purpose of timber extraction do not enable the establishment of a point of reference for the natural condition of the forest, which would be able to indicate whether such management practices influence the accumulation of this component in the soil.
Conclusion
The soil use change from forestry to induced pasture and seasonal agriculture causes alterations in the physical and chemical properties evaluated which is in large part, the quantitative and qualitative changes in the organic material incomes, as well as the initial condition of the soil and the management intensity.
The foregoing suggests the implementation of local soil and vegetation management practices, such as the incorporation of living fences, crop rotation, or soil conservation practices, which can lead to the capture of atmospheric carbon, but also the recovery of some soil properties which facilitates the soil ecological functions, such as carbon capture and water infiltration