INTRODUCCIÓN
Nitrogen (N) is absorbed in nitrate (NO3 -) or ammonia (NH4+) form, is the second most-required element by papaya plants [Carica papaya (Linn)], and it is important to meet its demand throughout the plant life cycle (Neto et al., 2011). Once absorbed, NH4+ can be quickly used in the synthesis of amino acids and other organic nitrogen compounds (Bybordi, 2010), and there is no need to be reduced to nitrate, avoiding energy waste. However, excess NH4 + can affect the development of the plants (Bittsánszky et al., 2015; Esteban et al., 2016). Most species of plants develop toxicity symptoms when subjected to high concentrations of NH4 +. This may lead to decreased photosynthesis, lower growth and yield, lower cation content, and variation in the content of metabolites such as amino acids or organic acids and rhizosphere acidification (Borgognone et al., 2013; Esteban et al., 2016), although this ion is an intermediate in several metabolic reactions (Li et al., 2014).
Different physiological and biochemical hypotheses may explain the action of NH4 + in plants. These include acidification of the growth medium and NH4+-toxicity per se leading to antagonism of cation uptake, and alterations in the osmotic balance (Britto and Kronzucker, 2002; Horchani et al., 2010; Nasraoui-Hajaji and Gouia, 2014). The pH of the rooting medium is of paramount importance for plant growth, as a large number of processes (e.g., nutrient availability, uptake rate and availability of toxic ion species) are closely related to this parameter (Marscherner, 2012). A consequence of acidification is the lower availability of cations, such as K, Ca and Mg (Ahmad et al., 2006).
The damaging effects of NH4 + nitrogen contrast themselves with the beneficial effects of Si (Campos et al., 2015), because this beneficial element increases the growth ofmany plants under abiotic stresses from different mechanisms (Bybordi, 2010). In addition, in unstressed plants it affects the increase of nitrogen metabolism, enabling increases in fresh mass, seed production, photosynthesis rates, chlorophyll contents, and the activity of nitrate reductase (Bybordi, 2012).
Studies involving the use of Si as a possible attenuator of the deleterious effects caused by excess NH4 + have been carried out for some crops. These have included benefits to rice (Ávila etal., 2010), corn (Campos etal., 2015), cucumber (Campos et al., 2016) and tomato (Barreto et al., 2016), cauliflower and broccoli (Barreto et al., 2017), radish (Olivera et al., 2017), and beets (Viciedo et al., 2019); however, there was no effect for other crops, such as plum trees (Ferreira et al., 2013). These effects of NH4 + toxicity vary from species to species with probable distinctions in the damage of the growth of the aerial parts in relation to the roots, due to the differentiated cation accumulations of these organs, and it may depend on the Si accumulation in the species studied. It is hoped that Si is more important in attenuating NH4 + toxicity for species that have greater uptake of Si.
Given the need for a better understanding of Si and NH4+ interactions, our hypothesis is that NH4+ toxicity could be attenuated with the use of Si, depending on its intensity. Additionally, the NH4+ toxicity in papaya plants induces more damage in shoots than roots, given the possible greatest restriction of cation uptake and reduction of plant growth. Therefore, the purpose of this research was to investigate the response of cations, Si accumulations, and plant growth impacts of different NH4 + concentration in papaya plants when Si is present.
MATERIAL AND METHODS
The experiment was carried out in a greenhouse at Sao Paulo State University (UNESP) - Campus of Jaboticabal, Brazil, of January to March 2015. The experimental design was in randomized blocks in a 5 x 2 factorial arrangement, with five NH4+ concentrations (10, 20, 40, 80, and 100 mmol L-1), in the absence and presence of Si (2 mmol L-1), with five replications. Each experimental unit consisted of a seedling cultivated in a polypropylene pot (1.7 dm3).
The experiment was conducted on papaya seedlings (Caricapapaya L, 'Grupo Formosa' (Calimosa híbrida 01). The nutrient solution proposed by Hoagland and Arnon (1950) was used, by modifying the source of iron for Fe-EDDHMA. The nitrogen source used to compose the treatments was ammonia chloride (NH4Cl) solution, and the Si source was monosilicic acid. The pH of the Hoagland nutrient solution was monitored daily and maintained at 5.5 ± 0.5 using 1 N hydrochloric acid (HCl) solutions.
Sowing of papaya was carried out in polyethylene trays, with a capacity of 1.5 dm3, which was filled with a commercial substrate based on pine and coconut fiber, and a seed was sown in each container. Irrigation was daily, and substrate moisture was kept near the maximum water retention. After emergence (22 days), the papaya seedlings were transplanted to polypropylene pots, keeping the same substrate mentioned above. Thereafter, over seven days, applications of the Si solution (2 mmol L-1) were performed, using 20 mL per container only for the treatments with Si and a complete nutritive solution with N as nitrate in all treatments. After seven days, the treatment with nutritive solutions containing NH4 + began. For each pot, a volume of 50 mL of the solutions was applied in the first 15 days; with the growth of the plants, that volume increased to 100 mL, which was applied daily according to the respective treatments.
The vessels used contained at the bottom a collecting container to return the nutritive solution, thus avoiding its loss. The collection of the nutritive solution and new application to the pots were performed in the early morning between 8:00 to 9:00 am. After applying the treatments, the plants were observed daily, and at the time of the onset of the visual symptoms of NH4+ toxicity, the descriptions of the symptoms, the images of the plants, and the dates of each observation were recorded.
After 31 days of treatment applications, the height of the seedlings (cm) were determined, from the base of the seedlings to the insertion of the first fully developed leaf, with support of graduated rule; and the stem diameter (mm), 5 cm above the base of the seedlings, was measured with a digital caliper. After plants were collected, they were washed with distilled water to remove residues. Then the plants were washed with a detergent solution (0.2 %), hydrochloric acid solution (0.1 %), and finally deionized water (Prado, 2020). The seedlings were separated into roots and shoots, and then they were placed in paper sacks and dried in a forced-air oven (Tecnal® TE 394-3-Brazil) at a constant temperature of 65° C until they reached a constant weight, and then the root dry matter (RDM) and shoot dry matter (SDM) (mg per plant) were obtained.
After obtaining the dry matter weights, the samples of the shoots were then pulverized with a Wiley mill fitted with a stainless-steel chamber and blades (Marconi® MA 360, SCompany, China). The concentrations of N, K, Ca and Mg were determined according to the methodology proposed by Bataglia et al. (1983), and Si was determined according to Kraska and Breitenbeck (2010). Total N, K, Ca, Mg and Si accumulation in shoots were calculated as the N, K, Ca, Mg, and Si concentrations per total plant dry weight (mg per plant) (Lawlor, 2002).
The data obtained were submitted to variance analysis (ANOVA), using the F test at 5 % of probability. When significant for the averages of the source of variation of NH4 + doses, the polynomial regression analysis was carried out. The appropriate regression equation was selected on the basis of higher R2 values. The study of the relationships between the variables was performed by means of Pearson correlation (r). For the statistical analysis, the software Assistat 7.7 beta was used.
RESULTS
We found significant (p < 0.05) main differences in NH4+ concentration and no interaction effects of NH4 + and Si application on Ca, Mg, K, N, and Si accumulation in papaya plants (Figs. 1a-e). The increase of NH4+concentration in the nutritive solution origin decreased linearly in the accumulations of Ca, Mg, K, and Si in the shoots of papaya seedlings (Figs. 1a, b, c, and e), regardless of the presence of Si, but it did not affect the shoot N accumulation.
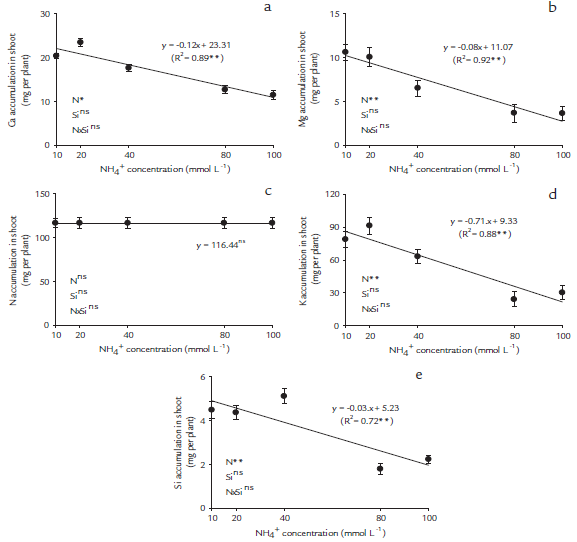
Figure 1 Cation accumulations in the shoots ofpapaya plants. Calcium accumulation (a), magnesium accumulation (b), nitrogen accumulation (c), potassium accumulation (d) and silicon accumulation (e), as a function of five NH4+ concentrations, 10, 20, 40, 80 and 100 mmol L-1, in nutritive solutions in the absence and presence of Si (2 mmol L-1). *Significant at p < 0.05 of probability of error **Significant at p < 0.01 of probability of error; ns-insignificant by the F test at p < 0.05.
There was a significant (p < 0.05) effect of NH4+ concentration and no interaction effect of NH4+ and Si on plant growth (Figs. 2a-d). In papaya plants, the plant heights (Fig. 2a) and stem diameters (Fig. 2b) decreased linearly with the increase of NH4+ concentration supplied via nutritive solution, regardless of the presence of Si.
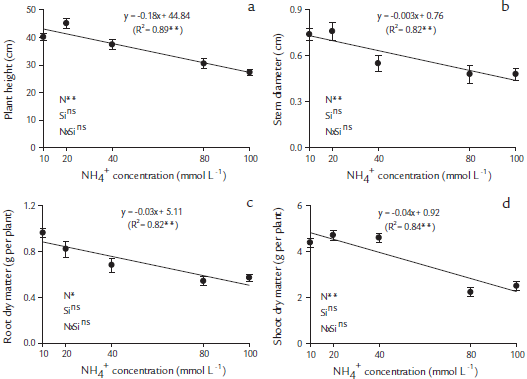
Figure 2 Plant growth characteristics in papaya seedlings, plant height (a), stem diameter (b), root dry matter (c) and shoot dry matter (d), as a function of five NH4+ concentrations, 10, 20, 40, 80 and 100 mmol L-1, in nutritive solutions in the absence and presence of Si (2 mmol L-1). ** Significant at p < 0.01 of probability of error; ns-insignificant by the F test at p < 0.05.Table 1. Correlations between the evaluated variables in papaya plants cultivated in nutritive solutions.
There were significant differences (p < 0.05) for NH4+ concentrations and no interaction between Si and NH4+ levels on root and shoot dry matter production in papaya plants (Figs. 2c and d). In papaya plants, the increase of NH4+ concentration showed a linear decreased in RMD (Fig. 2c) and in SDM (Fig. 1d), even when Si was present.
A significantly high correlation (p < 0.01) was observed among all variables evaluated (Table 1). The NH4+ toxicity induced decreases in dry matter production in shoots, especially with the strong correlation with the reduction of chemical element accumulations, such as K (r = 0.95**), total N (r = 0.94**) and Mg and Si (r = 0.93**) and by lower accumulations of RDM (r = 0.90**) (Table 1).
Table 1 Correlations between the evaluated variables in papaya plants cultivated in nutritive solutions.
VA/VA | Ca1 | Mg2 | N3 | K4 | Si5 | PH6 | SD7 | SDM8 | RDM9 |
---|---|---|---|---|---|---|---|---|---|
Ca | 1 | 0.90 | 0.85 | 0.87 | 0.74 | 0.80 | 0.85 | 0.89 | 0.84 |
Mg | ** | 1 | 0.81 | 0.95 | 0.83 | 0.80 | 0.85 | 0.93 | 0.89 |
N | ** | ** | 1 | 0.85 | 0.86 | 0.82 | 0.80 | 0.94 | 0.84 |
K | ** | ** | ** | 1 | 0.86 | 0.85 | 0.86 | 0.95 | 0.86 |
Si | ** | ** | ** | ** | 1 | 0.85 | 0.79 | 0.94 | 0.82 |
PH | ** | ** | ** | ** | ** | 1 | 0.95 | 0.89 | 0.84 |
SD | ** | ** | ** | ** | ** | ** | 1 | 0.88 | 0.91 |
SDM | ** | ** | ** | ** | ** | ** | ** | 1 | 0.90 |
RDM | ** | ** | ** | ** | ** | ** | ** | ** | 1 |
1 accumulation of Ca; 2 accumulation of Mg; 3 accumulation of N; 4 accumulation of K; 5 accumulation of Si; 6 plant heights; 7 stem diameters; 8 shoot dry matter; 9 root dry matter. Pearson correlation coefficients and their significance are given as **p < 0.01.
DISCUSSION
EFFECT OF NH4+ ON CATIONS AND SILICON ACCUMULATION
The overall results of this experiment suggest that 20 mmol-L-1 of NH4+ supplementation is enough to cause toxicity in papaya plants. Many plant species have been characterized as NH4+ tolerant or sensitive (Cruz et al., 2011). This study demonstrated that papaya plants are very sensitive to NH4+ application and showed a decrease of cations and Si accumulations (Figs. 1a-e), which led to a consequent reduction in the plant growth, even when Si was present. Therefore, the use of Si in nutrient solution did not affect papaya plant growth with or without ammonia toxicity (Figs. 2a-d).
In papaya seedlings, increasing of NH4+ concentrations significantly decreased the Ca, Mg, K and Si accumulations (Figs. 1a, b, d, and e), which caused significant reductions in plant growth (Figs. 2a-d), and high correlations among these variables (Table 1). In contrast, the exclusive supply of N as NH4+ is harmful to many plants and may cause ion imbalances (Szczerba et al., 2008; Domínguez-Valdivia et al., 2008). Recent studies have indicated that high concentrations of NH4+ decrease the accumulation of Ca and Mg in tomato plants (Borgognone et al., 2013). This is corroborated by a acidification of the rhizosphere leading a lower cation content, changes of several metabolite levels, leaf chlorosis, net photosynthesis decreases (such as amino acids or organic acids), and lower plant yields (Britto and Kronzucker, 2002).
An excess in NH4+ is reflected in the integrated phenotypic symptoms, such as reduced plant growth, changes in root architecture, decreases in the root/shoot ratios, and leaf chlorosis, among others (Bittsánszky et al., 2015; Esteban et al., 2016). This causes the inhibition of cations K, Mg or Ca uptake and consequent changes in plant ion balance, intra-cellular alkalization and extracellular acidification, the inhibition of root respiration and stimulation of photorespiration, interference with photosynthetic activity, and altered expression/activity of NH4+ (Cruz et al., 2011; Ariz et al., 2011; Esteban et al., 2016).
On the other hand, the decreased cation and Si uptake and accumulation in shoots of papaya plants can be attributed to the drastic reduction in root dry weight, which has also been observed in other sensitive species, such as Arabidopsis sp. (Li et al., 2014). The severity of NH4+ toxicity is increased when K is in a low concentration in the production system. It is important for NH4+ assimilation and in the formation of the amino acids (Ten Hoopen et al., 2010; Li et al., 2014). This situation did not occur in the present study, since an adequate concentration of K was used in the nutritive solution. Recent studies have indicated that NH4+ excess caused toxicity and ion imbalance in different species, such as wheat (Britto et al., 2001; Szczerba et al., 2008), plum trees (Ferreira et al., 2013), tomato (Von Wiren et al., 2000; Barreto et al., 2016), cauliflower and broccoli (Barreto et al., 2017), radish (Olivera et al., 2017), and more recently in sugar beet (Viciedo et al., 2019).
The presence ofSi in the nutritive solution did not affect the accumulation of K in the shoots of papaya plants (Fig. 1d). Different results were obtained in canola by Bybordi (2010), who observed an increase in K accumulation in the presence of Si without the occurrence of stress. The difference among results was due to the NH4 + toxicity in the present work inhibiting the interaction Si and K, and this situation did not occur in the cited work.
It has been reported that NH4+ toxicity can decrease Ca and Mg uptake. Such findings have also been demonstrated in other recent studies of different plant species, such as Hydrilla verticillata (Wang et al., 2010), Egeria densa (Su et al., 2012), and tomato (Borgognone et al., 2013).
In the present study, the Si accumulation was decreased according to increasing NH4+ concentrations, even when Si was present in the nutritive solution (Fig. 1e). This presupposes that the Si supply did not promote the cation uptake, and had been reported previously in rice plants (Mauad et al., 2003). One possible explanation for the reported findings is that papaya plants are dicots and are considered a non-Si-accumulating plant (Hodson et al., 2005). However, some dicots, such as cucumber, melon, strawberry and soybean take up Si passively (Mitani and Ma, 2005), and tomato and beans exclude Si from uptake (Liang et al., 2005; 2007). Recently, in dicotyledonous plants, the uptake of monosilicic acid (MSA) has been determined to be mediated by a process of diffusion, resulting in significantly lower MSA concentrations in the xylem compared to monocots (Mitani and Ma, 2005).
EFFECT OF NH4 + ON PLANT GROWN
To avoid toxicity, plants need to maintain a fine balance between the uptake, production, and consumption of NH4 + (Bittsánszky et al., 2015). This leads to the question of the nature of the first response of plants to high levels of NH4+ (Esteban et al., 2016). Therefore, the use of Si in the nutritive solution did not affect the growth of the papaya plants with or without NH4+ toxicity. A similar result was observed by Ferreira et al. (2013) for plum trees, in which Si did not promote the growth of the plants. The low importance of Si for papaya plants was possibly because the plant is not accumulative of the element, a classification that covers most dicotyledons (Hodson et al., 2005).
In this study, the excess NH4+ affected the root dry weight, even when Si was present (Fig. 2c), this could be affected by the cation and Si uptake (Figs. 1a, b, d, and e). Our results corroborate the work done by Borgognone et al. (2013), in which the root dry matter of tomato decreased with the increase ofNH4+ dose, from the concentration of0.021 mmol L-1. This is possible because, in NH4+ sensitive plants, such as papaya, the plant growth was affected. A similar effect had been previously reported in Arabidopsis sp., in which shoots tended to be the parts of the plant most sensitive to NH4+ (Li et al., 2014). In contrast, the roots constitute the first NH4 + sensor, and the initial signals of NH4 + toxicity appear at the root level, with a severe modification of the root system architecture. Commonly observed modifications include shorter primary root systems, the inhibition of root elongation, and embracing primary and lateral roots (Rogato et al., 2010; Esteban et al., 2016).
Depending on the dosage, the exclusive supply of N as NH4 + may cause poor biomass accumulation (Szczerba et al., 2008; Domínguez-Valdivia et al., 2008). It has been observed that excess NH4 + in nutritive solutions resulted in the growth suppression of corn plants (Campos et al., 2015) and tomato plants (Borgognone et al., 2013), which occurred with NH4 + concentrations in the nutritive solution from 30 mmol L-1 and 1 mmol L-1, respectively. These results differ from those presented by (Mendoza-Villarreal et al., 2015), in which the application of 15 mmol L-1 of N with different NH4 + proportions promoted an increase in the stem diameters and heights of lisianthus plants (Eustoma grandiflorum (Raf.) Shinn), according to the increase of NH4+ proportion in the nutritive solution.
On the other hand, in sunflower plants, applying the concentration of 210 mg L-1 of N in the NH4+ form, resulted in a decrease in the dry matter production of the plants (Silva et al., 2010). NH4+ caused a reduction in the development of Salvinia cucullata under concentrations of 10 and 15 mmol L-1, making the roots smaller and atrophied (Jampeetong et al., 2012). Probably the growth inhibition has been related previously by Britto et al. (2001). Britto and Kronzucker (2002) and Von Wiren et al. (2000) verified that the futile transmembrane cycle of NH4+ uptake and efflux through cell roots may carry a high energetic cost and be responsible for detrimental effects.
It was shown by the angular coefficients of the regression line that each 1 mmol L-1 of NH4+ added to the solution reduced the SDM by 0.0285 g per plant and the RDM by 0.0042 g per plant, totaling 0.0327 g per plant. The highest proportion of this decrease in the plant dry matter induced by NH4+ toxicity occurred for SDM (87 %) as compared with the RDM (13 %) (Figs. 2c and d). This greater loss of dry matter accumulation of the aerial part in relation to the plant roots under NH4 + stress is due to a slight restriction of root of cation uptake that easily reaches the aerial parts without having accumulated in the plant roots. Excess NH4+ in the aerial parts increases the contents of oxygen (O2) and hydrogen peroxide (H2O2), inducing oxidative stress, lower contents of carotenoids (Wang et al., 2010), lower photosynthetic rates, stomatal conductance (Borgognone et al., 2013), and increases in ethylene synthesis (Britto and Kronzucker, 2002), reflected in the decrease in the dry matter of aerial parts.
Symptoms of NH4 + toxicity in papaya plants were characterized by the necrosis of older leaves, yellowing, and death of leaves, similar to that described by Esteban et al. (2016). These symptoms were evident from the 40 mmol L-1 NH4+ concentration. Similar results for toxicity were observed by Holzschuh et al. (2011) in rice plants at a 5 mmol L-1 NH4 + concentration in the nutritive solution. Differences in tolerances of the species to the toxicity depend not only on genetic factors, but also on the concentrations of other nutrients in the nutritive solution.
It is also worth mentioning that increasing ammonium concentrations in nutrient solutions resulted in increased Cl ions, reaching 100 mmol L-1, and this could have aggravated the ammonia toxicity. However, plants have high chlorine tolerance, may have in their tissues 20 to 200 times more than adequate levels without causing toxicity (Malavolta et al., 1997), but there may be differences between species. According to Greenway and Munns (1980), dicotyledonous species continue their growth at concentrations between 250-500 mmol L-1 of Cl, presenting an optimal growth near 250 mmol L-1. While for papaya plants, information is restricted for the Cl concentrations in nutrient solutions that impair its growth.
Thus, our results confirm the hypothesis that increased concentration of NH4 + in the nutrient solution resulted in a deleterious effect in ion balance and papaya seedlings even when silicon was present. This study provides the evidence that effect of Si supplied in nutrient solution alone did not attenuate the NH4+ toxicity in this sensitive species. There is, however, the possibility of studying the combined root and foliar applications of Si, to increase the Si accumulation in non-accumulating plants and possible benefits.
CONCLUSIONS
The results confirmed that ammonia applied in high concentration markedly adversely affected the uptake of cations and plant growth of papaya plants. Consequences revealed from increased ammonia concentrations showed toxicity in papaya plants and stronger reduction in Ca, Mg, K and Si accumulations, plant heights, stem diameters, and root and shoot dry matter productions even when silicon was present. Although all levels of added ammonia effectively increased the deleterious effects on papaya plants, there was a greater effect on the shoot dry matter (87 %) than on the root dry matter (13 %).