INTRODUCTION
Aedes albopictus, also known as the Asian tiger, is a mosquito from Southeast Asia, the Pacific and Indian Ocean Islands. It has spread and colonized every continent except Antarctica in the past 30-40 years, primarily by trading of tires, and is expected to continue to disperse1-2). A. albopictus is commonly found in sub-urban, rural, semi-rural and savage environments from tropical, subtropical and temperate regions2,3,4). The Asian tiger mosquito has been linked to the transmission of arboviral and filarial infectious diseases of humans and animals5-6). Its high potential to carry a wide range of human pathogens is consequently of wide concern.
A. albopictus presents vector competence for 26 arboviruses from the families Flaviviridae (e.g., Dengue virus, Nile virus, yellow fever, Japanese encephalitis), Bunyaviridae (e.g., Potosí, LaCrosse virus), Togaviridae (e.g., Chikungunya and Ross River virus) and Reoviridae (e.g., Orungo and Nodamura virus)(7,8,9). Naturally, A. albopictus is able to transmit important diseases such as dengue and chikungunya fever. The Asian tiger mosquito has played a significant role in Chikungunya virus (CHIKV) outbreaks in Central Africa, Asia and Europe10,11,12,13. In addition to CHIKV, A. albopictus, a species that is sympatrically distributed with Aedes aegypti, is epidemiologically important in transmitting the dengue viruses (DENV) throughout areas of Southeast Asia, Africa, North America and Europe14,15).
Worldwide, Aedes aegypti is the primary vector for the DENV, a disease that remains a serious public health problem in many tropical and subtropical countries16. In the Americas, A. aegypti is the only confirmed natural dengue virus vector17. Although its geographical distribution is more limited, A. albopictus is considered a potential vector in the Americas due to the high level of vector competence of local populations for DENV18-19). A meta-analysis of 14 studies on the relative susceptibility of A. albopictus and A. aegypti for DENV suggests that A. albopictus is more susceptible to midgut infections than A. aegypti; however, the ability of the virus to disseminate in the latter mosquito is considerable, suggesting a greater potential for transmission in nature20. Nevertheless, currently A. aegypti is the primary vector for the DENV in the Americas21,22).
Given the sanitary and epidemiological importance of A. albopictus, the understanding of the patterns of genetic structure and gene flow among A. albopictus populations is pivotal for the development of rational vector control programs23. Population genetics studies of A. albopictus have been carried out globally as the species continues to spread and displace A. aegypti in some áreas4. Different genetic markers have been used to study the population genetic structure of A. albopictus, such as Isozymes/Allozymes24-25), Restriction Fragment Length polymorphism (RFLP26), Random Amplified Polymorphic DNA (RAPD27), Mitochondrial DNA (mtDNA(28,29,30) sequence haplotype, ribosomal DNA (rDNA(31) and Microsatellites32.
Genetic studies with early populations of A. albopictus, using Isozymes/Allozymes, indicated that populations cluster by continent or country of collection24,25,33,34. Subsequent researches examined variation at smaller and/or wider geographic scales using molecular markers such as RAPD, mtDNA, rDNA and microsatellite; these genetic studies report varying levels of population differentiation at both local and continental scales29,35,36).
Population genetic studies provide insights into the basic biology of arthropod disease vectors by estimating dispersal patterns and their potential to spread pathogens37. Significant progress has been made in understanding insect diversity and ecology by using protein markers such as isozymes/allozymes38. The isozymes, developed in the late 70s, were originally defined as multiple molecular forms of enzymes with identical or similar functions and that are present in the same individual39-40. The isozymes may have different allelic forms known as allozymes(41). The isozymes application is guided for quantifying heterozygosity, genetic diversity, genetic differentiation and other measures of genetic variation within and among populations. However, one of the problems of the protein markers is the lack of ability to detect polymorphisms between related species, since the proteins are the result of gene expression, which may differ from one tissue to another, from one stage of development to another, or from one environment to another42.
Protein markers made a significant contribution in the early periods when DNA technologies were not as advanced as it is now. However, with the development of DNA-based marker systems, such as RFLP, RAPD, mtDNA and microsatellites, it was found that a greater level of polymorphism could be obtained by using DNA rather than protein markers in many cases43. The RFLP was the first DNA marker used in population studies44 and is used to detect DNA fragments from different molecular weights (by digestion with the same restriction enzyme) in different organisms, usiny electrophoresis on agarose or polyacrylamide gel38. The RFLP has been used for constructing genetic maps, cloning of genes based on maps and for helping to resolve taxonomic and phylogenetic problems45. However, the main disadvantage of the RFLP is the requirement of large amounts of high quality DNA to recognize loci single copies, which only detect a fraction of the variability of existing sequences in the genome, which means the information is limited46.
The RAPD markers method has been reported to be an efficient tool to differentiate geographically and genetically isolated population. The RAPD technique uses the PCR principle for random amplification of DNA sequences. The RAPD-PCR is a dominant type of molecular marker, that is unable tt differentiate heterozygotes from homozygotes43. These markers allow the study of a large number of loci and provide a random sampling of DNA, therefore, present high levels of polymorphism compared to RFLP and protein markers46. However, they have significant limitations when compared to codominant markers (e.g., microsatellites) and/or haploid (e.g., mtDNA), since, the amplified fragments often do not correspond to DNA bound to a character, but to one repeated, and it does not provide information about the number of copies of genomic DNA containing the amplified sequence43).
The mtDNA is used for marker analyses largely because of their maternal inheritance, haploid status, and high rate of evolution47. The mtDNA is a type of marker used for the recognition of cryptic species, phylogenetic studies and/or genetic structure of populations48,49,50. One of the disadvantages of using mtDNA in population and phylogenetic studies is the presence of nuclear mitochondrial pseudogenes (NUMTs)51-52. NUMTs are non-functional copies of mitochondrial sequences that have become incorporated into the nuclear genome53. Samples containing mixtures of mtDNA and NUMT sequences are expected to significantly affect the outcome of genealogy- and frequency-based analyses. This is because mtDNA and NUMTs have separate genealogies and thus, evolutionary history52.
The ribosomal DNA (rDNA) can be found in the mitochondria, chloroplast and nucleus. The rDNA has been analyzed at the structural level in a large number of multicellular eukaryotes, including insects54. The rRNA occurs in tandem repetitions and it consists of three highly conserved subunits (18rDNA, 5.8rDNA and 28rDNA),dseparated by two External Transcribed Spacers (ITS1 e ITS2) with high replacement rates55. Due to the low rate of substitution present, these sequences are useful in phylogenetic studies on taxa with old divergence time56. Nevertheless, it has been found NUMTs in A. aegypti derived from the tRNA and rRNA genes throughout the mtDNA genome53.
Microsatellites are also used as popular markers in insect studies because of the high abundance and highly variable nature of their loci in genome57. However, in contrast to most other arthropods (e.g., Anopheles gambiaes.58), microsatellites appear to be underrepresented within some members of the mosquito subfamily Culicinae (e.g., Culex pipiens, C. pipiens quinquefasciatus, and A. aegypti59-60). Nevertheless, in A. aegypti for instance, microsatellites are commonly used in population genetics studie(61).
Regarding these marker systems (Isozymes, RFLP, etc.), some details about A. albopictus movement, gene flow patterns and genetic structure has been inferred. However, no published article has focused on analyzing the current scenario of the genetic diversity from natural populations of the Asian tiger mosquito. Hence, the objective of this systematic review was to defind the current scenario of the genetic diversity of natural populations of A. albopictus. For this purpose, data from the first record and distribution of the vector was compiled and included; besider, discussion as focused on the current knowledge of genetic diversity through different molecular techniques. Finally, some important gaps of knowledge, that needed to be addressed, were identified for further research.
MATERIALS AND METHODS
Throughout May 2014, a systematic review was carried out on articles about: The first records of the vector, Genetic diversity, and distribution of natural populations of A. albopictus. Distribution data of the vector was considered from the reviews authored by: Rai62, Benedict et al.63), Caminade et al.2, Medlock et al.64 and Bonizzoni et al.1. The database used for the research of the early records of the vector and the genetic diversity, sere: Web of Knowledge (“all databases”, including Biological Abstracts, Biosis, Current Contents Connect, Web of Science, and Zoological Records) by Thomson Reuters and the Google search engine (limited to the first five pages of results). The Google search engine was used to identify reports, conference abstracts, guidelines, etc. Data research was performed including all dates andelimite to sources i: English, Spanish and Portuguese. keywords used for the research on the early records of the vector was, ‘Aedes albopictus’ followed by the phrase ‘first record’. Only the first record for country was considered. Regarding the research on genetic diversity, the keyword used was: ‘Aedes albopictus’ followed by the terms ‘genetic diversity’, ‘gene flow’, ‘population structure’ ‘population genetics’, ‘mtDNA’ and ‘nuclear DNA’. From the results of the research, all the titles and abstracts found were read, and from these, only articles related to the search criteriasweretconsidered. After reading the title and abstract, replicas and items that did not meet the inclusion criteria, were removed from the search. The publications included in the analysis were summarized using a data extraction tool developed from Microsoft Excel 2010. Two data matrices were constructed: one related to the first record-distribution and the other on genetic diversity. The first matrix on the first record-distribution contained data such as: Location (state, city, region, county, district, and street), year, geographic coordinates, distribution, and references. The second one, on the genetic diversity, included data like: Location (state, city, region, county, district, and street), geographic coordinates, genetic diversity (polymorphic diversity / haplotype / gene / nucleotide), molecular technique, genetic structure (p-value that indicate genetic structure such as: X2 test (Isozymes/ Allozyme)/GST (RAPD)/F ST (mtDNA, Microsatellites)) and references. Maps were designed based om the geographical coordinates of the two matrices and the molecular techniques. The georeferencing data were calculated using Google Earth 7.1.
RESULTS
A total of 65 published articles between 1987 and 2014 were analyzed. From these articles, 63% referreg to the first record of the vector and the other 37% on genetic diversity (Table1-2). The first record of A. albopictus outside Asia (place of origin of the vector) was registered in 1979 in Europe (Albania). Since then, the Asian tiger has been dispersed in the continents of Oceania, Africa, Europe and America during the last 36 years (Figure 1a,Table 1). In Oceania, the vector is present in 10 of the Torres Strait Islands, since its appearance in Brisbane (Queensland, Australia) in 1988. In Africa, there are records of A. albopictus from 1991 in Nigeria (Delta State) and South Africa (Cameroon). However, nowadayt, there are no records of the vector along the African continent. In Europe, A. albopictus has been confirmed in 16 countries from the continent after its appearance in Albania (1979), and later in Genova (Italy) in 1990. In America, A. albopictus was initially introduced in the middle of the decade of the 1980 in United States (Texas). Consequently, the Asian tiger has been registered in South America and Central America since 1980 until 1990, primarily in Brazil (1986, Rio de Janeiro) and Mexico (1988, Coahuila), and subsequently in the remaining countries (Table 1).
Literaturs on genetic diversity showed that the Asian tiger populations have been studied in all ite distribution arear (Figure 1b). A total of 267 vector populations have been studied throughout the world. The largest number of populations studied was founs in the American continent (37%) followed by Europe (21%), Africa (20%), Asia (16%) and Oceania (6%). The 37% in the American continent is distributed into: 56% in North America (United States), 41% in South America (mainly Brazil) and 3% Central America (Dominican Republic, Guatemala and Cayman Islands; Figure 1b, Table 2).
In general, most of the populations of A. albopictus have hat genetic structure studies at regional and global levels. The genetic diversity (Hd) of the Asian tiger populations ranged from 0.0 (Central Africa) to 0.83 (China, Singapore, Japan, Italy, United State), rnonetheless, most of the Hd studies results were lower than 0.7. Furthermore, the haplotype diversity (π) of the Asian tiger populations ranged from 0.00 to 0.30 (Table 2).
The data were obtained from the published literature (Table1-2). The colors indicate vector distribution: Gray (Unknown or no data), Red (Indigenous) and Blue (Current distribution range).
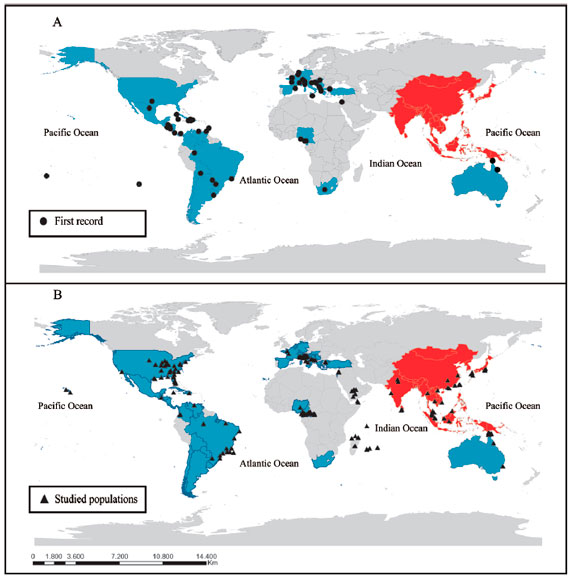
Figure 1 Political maps indicating: A. The first record and distribution of A. albopictus, and B. The A. albopictus populations used in genetic diversity studies.
Table 1 First records (in chronological order) of natural populations of A. albopictus in the world.
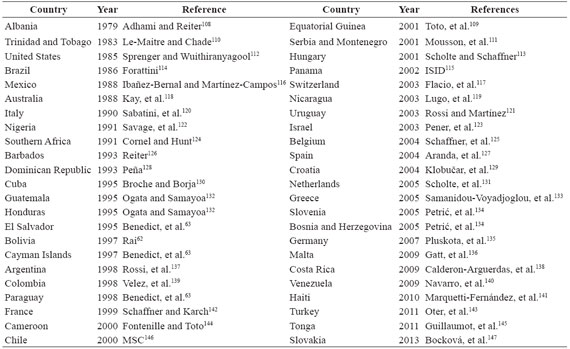
findings indicate that molecular techniques used in studies on genetic diversity of A. albopictus are: RFLP, Allozymes, Isozymes, RAPD, mtDNA (Cytb, COI, ND5), microsatellites and ITS2. However, there were also found studies in which more than one molecular technique was used such as: mtDNA and microsatellite and mtDNA and ITS2 and (Table 2). From the total of the population studied, 50.9% have been analyzed using mtDNA, 24.7% allozymes, 7.5% isozymes, 6.4% RFLP, 4.5% mtDNA and microsatellites, 3.0% mtDNA and ITS2, 2.6% RAPD and 0.4% microsatellites (Figure 2). On the other hand, the 89.5% of the known genetic diversity is based on the use of RFLP, allozymes, isozymes, and mitochondrial molecular markers, which exhibie problems reported on the literature. Molecular techniques (in inverse chronological order) used to estimate genetic diversity in A. albopictus populations are: ITS2 (2013-present), microsatellites (2011-present), mtDNA and RAPD (2002-present), and RFLP, allozymes and isozymes (1988- 2003) (Table 2).
Table 2 Genetic diversity worldwide observed (in chronological order) in natural populations of A. albopictus using various molecular markers.
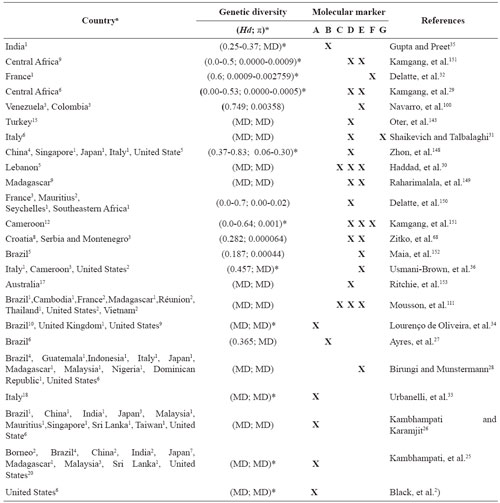
n = Number of cities/towns/sampled regions; Hd = haplotype diversity; π = nucleotide diversity; * = Genetic structuring (p < 0.05); MD = missing data; A = RFLP/Isozymes/Allozymes; B = RAPD; C = Cytb; D = COI; E = ND5; F = Microsatellites; G = ITS2.
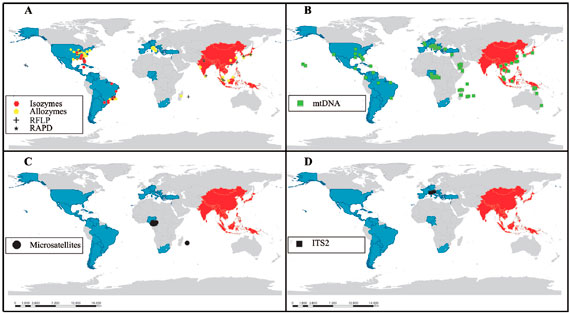
Figure 2 Political maps showing the populations of A. albopictus analyzed in genetic diversity studies using the molecular techniques: A. Isozymes, Allozyme, RFLP and RAPD; B. mtDNA (Cytb, COI and ND5); C. Microsatellite; and D. ITS2. The colors indicate vector distribution: Gray (Unknown or no data), Red (Indigenous) and Blue (Current distribution range).
DISCUSSION
This study revealed that A. albopictus is distributed globally wity structured populations exhibiting low genetic diversit,; most of the genetic diversity known is based on genetic markers that present witw significant problemse. For the last 36 years, the Asian tiger has spread form Asia (place of origin) to Oceania, Africa, Europe and the Americ as. However, mathematical models of distribution indicate that A. albopictus will continue spreading all over the world due to factors such as transportation means, the environment and climate change20,21,22,23,24,25,26,27,28,29,30,31,32,33,34,35,36,37,38,39,40,41,42,43,44,45,46,47,48,49,50,51,52,53,54,55,56,57,58,59,60,61,62,63,64,65. Successful dispersion of A. albopictus is associated mainly to its ecological plasticity (i.e., the vast array of breeding habitats ranging from treeholes and cut bamboo to a wide variety of man-made containers), and also, to its passive transport of eggs through the international trade of semi-new tires, plants shipping (Dracaena spp.) from Asia, accidental transportation of adults in aircrafts and other means of transportation1,2,3,4,5,6,7,8,9,10,11,12,13,14,15,16,17,18,19,20,21,22,23,24,25,26,27,28,29,30,31,32,33,34,35,36,37,38,39,40,41,42,43,44,45,46,47,48,49,50,51,52,53,54,55,56,57,58,59,60,61,62,63,64.These situations make A. albopictus a highly invasive species, and also link the gene flow among A. albopictus populations to the human transportation, as it was globally observed in A. aegypti populations66.
The pattern observed of genetic variation in populations of A. albopictus may be attributed to the chemical measures used in vector control programs67. Worldwide, extensive and repeated insect control activities have involved source reduction and insecticide application, leading to the reduction and/or eradication of A. albopictus populations68-69. As a result, reduced levels of genetic variation were observed in the current study. Increased use of insecticides for agricultural pest control, for direct control of A. albopictus or for control of sympatric vectors (e.g., other Anophelinae and Culicinae species), has imposed selection pressures on A. albopictus populations for increased resistance, as it was observed in A. albopictus populations from Asia, Africa, Central America and South America70-71. In these resistant populations, genetic polymorphisms could have decreased quickly on any part of the mosquito genome due to the use of insecticides, thereby showing a low genetic diversity.
Low genetic diversity is most likely a result of a decline in population size caused by insecticide use, as it was observed in American A. aegypti populations72,73,74. However, some studies have revealed the presence of greater genetic diversity in areas that are frequently treated with insecticides, as shown in A. aegypti populations from French Polynesia and Brazil75-76. In our findings, most of the genetic diversity of the Asian tiger populations were lower than 0.7. Those results were lower than in other studies on the mtDNA ND4 gene of A. aegypti, a genetic marker widely used in genetic diversity studies in A. aegypti.47,77,78. For instance, in 36 locations in the Americas, Asia and Africa (Hd = 0.80) 79) and five states in Brazil (Hd = 0.80)80 showed higher genetic diversity than the observed in A. albopictus populations.
Most Asian tiger populations were genetically structured, a trend also found on A. aegypti populations from Asia, Africa, and America23,61,72. The genetic structure of A. albopictus populations have implications for vector control program, since, studies on selection pressure in A. aegypti populations using insecticides such as organophosphates and/ or pyrethroids under laboratory conditions, show the fixation of the population resistance phenotype in only a few generations81,82,83,84,85.
For the development of control programs, it is important to know the dispersal patterns and genetic diversity of the vector79,86. Genetic markers are widely used to understand the biology and population dynamics of disease vectors87. However, in our study, the 89.5% of the known genetic diversity is based on the use of RFLP, allozymes, isozymes, and mitochondrial molecular markers, which have problems reported in the literature. For instance, the RFLP, allozymes and isozymes markers (developed in the late 70s) are no longer employed in genetic diversity studies, since they present significant limitations when compared to microsatellites and/or mtDNA, due to these show little variation, need sufficient training time and also are poorly reproducible in the laboratory88,89.
Nevertheless, the main concern is that most of the genetic diversity found in the Asian tiger mosquito populations (51%) is through the use of mtDNA markers. In the last decade, the use of mtDNA has been widely used in population genetics studies for reconstructing historical patterns of population demography, admixture, biogeography and speciation in arthropods, included A. albopictus47,69. However, integration of mitochondrial sequences in nuclear DNA (referred to as NUMTs) has been discovered in many eukaryotes, including A. aegypti53,90,91,92,93. Thus, PCR amplification using mtDNA marker loci using total genomic DNA can potentially amplify these nuclear copies. These sequences complicate the employment of mtDNA as a molecular marker in genetic studies. In insects, because of the relative small genome size, high copy number of NUMTs sequences may interfere in effective separation of mtDNA from its nuclear paralogs52,94. This has been evident among 85 sequenced eukaryotic genomes where the NUMTs sequences were found to have different mitochondrial origin95. Thus, population studies using mitochondrial markers derived from these loci can potentially mislead the results.
Another problematic issue of using mtDNA markers has been identified in cases where the host insect harbours maternally inherited microorganisms such as Wolbachia. It is a gram-negative endosymbiotic bacterium that causes many developmental defects such as cytoplasmic incompatibility, feminization and sex ratio distortion96. As the Wolbachia infection sweeps through an insect species, the frequency of mitochondria from infected individuals also increases in the population due to the similar mode of transmission used by Wolbachia and the mitochondria. As a result, the spread of the mtDNA from infected individuals reaches high prevalence in these populations, phenomenon commonly referred to as ‘genetic hitchhiking’. Thus, inferring evolutionary history of populations solely based on use of mtDNA markers in insect species harboring such maternally inherited microorganisms may be misleading97. Wolbachia is commonly found in mosquitoes including A. albopictus. This species naturally carries two strains of the bacterium Wolbachia, wAlbA and wAlbB98. Wolbachia inherited bacteria are able to invade insect populations using cytoplasmic incompatibility and provide new strategies for controlling mosquito-borne tropical diseases, such as dengue and Chikungunya fever, as shown by Blagrove, et al.99 and Mousson, et al.98 in their works.
Currently, there is no presence of NUMTs in A. albopictus, therefore, further studies should be done in order to reduce the error caused by NUMTs in the published mtDNA (COI, Cytb, ND5) sequences. Here, we suggest the search for heterozygous sites in the chromatogram and additional termination codons. Common analysis applied on population genetics studies in A. aegypti when mtDNA markers are used (see: Gonçalves, et al.47; Aguirre-Obando, et al.72).
Despite the mtDNA markers have been widely used in vector genetic diversity studies, including A. albopictus29,100), these are not as sensitive to detect genetic variation as microsatellites and/or SNPs (Single Nucleotide Polymorphism) are61,87. Microsatellites have been used as genetic markers for a number of arthropod vectors of human diseases, including A. albopictus32. However, there are a few studies using microsatellites in A. albopictus as our findings show. Nevertheless, the use of microsatellites in A. albopictus populations has shown they are highly polymorphic. Delatte et al.32 using 10 microsatellites (two of them previously used in A. aegypti) in A. albopictus populations from Reunion Island, in the southwest Indian Ocean, found population genetic structuring. An alternative to increase the number of polymorphic microsatellites in population genetics studies in A. albopictus would evaluate the microsatellites described for A. aegypti (33 microsatellite loci60), as some of them has proved to be highly polymorphic32.
On the other hand, the SNPs, are the most common way of molecular variation in vertebrates and invertebrates101,102,103,104. Currently, SNPs have become one of the selectable markers for studies on population genetics, characterization of genes or disease to elucidate the evolutionary processes at the molecular level, since they are easy to detect when compared, for example, with microsatellites87,105. In vectors diseases such as Anopheles gambiae, A. funestus (vectors of malaria in Africa) and A. aegypti, SNPs have been highly polymorphic87,104,106-107. For A. aegypti, Paduan & Ribolla106 sequenced seven genes of 16 Brazilian populations of this species. These genes revealed the existence of 53 individual SNPs; eight of them are independent and highly polymorphic to be used in genetic diversity studies. Since, our search did not find any work related to the use of SNPs in A. albopictus, we suggest to test the polymorphic SNPs described for A. aegypti in A. albopictus, since other molecular markers developed in A. aegypti like microsatellites, have shown highly polymorphic in A. albopictus32. It can be concluded then, that the current scenario of genetic diversity in A. albopictus populations, is based on genetic markers that present significant problems reported in the literature, thus vector control programs, understanding of the vectors transmission, and the spread of genetic traits, such as vector competence and insecticide resistance, may be compromised.