Introduction
Cattle (Bos indicus and B. taurus) production in Venezuela is a pastoral activity, subject to forage dry matter (DM) availability variations according to the year dry or rainy season (Mora et al., 2010). In the Venezuelan beef cattle production systems to provide roughage preserved (hay, silage) during the dry season is not a common practice, even in the Brahman cattle genetic centers (73.3 % of these) (Depablos et al., 2010). Therefore, the feeding practices seek to maximize the efficiency of forage use and/or correct nutrient deficiencies (Mora et al., 2014). The low quality of tropical forages is known in terms of crude protein (CP; 40-108 g/kg DM) (Lima et al., 2018; Mlay et al., 2006), metabolizable energy content (1.36-1.85 Mcal/kg DM) (Mlay et al., 2006), and the greater content of neutral detergent fiber (NDF), with values from 675 to 759 g/kg DM (Lima et al., 2018; Mlay et al., 2006). Higher NDF and lower protein values have been reported in some forage species at different seasons of the year in central (Depablos et al., 2009; Herrera et al., 2009) and western Llanos of Venezuela (Mora et al., 2014; Parada & Mora, 2019), therefore, the animals are subjected to periods of malnutrition during their productive life.
In addition to the environmental conditions, herds have groups of animals more susceptible to these effects of low supply of forage and nutrients such as replacement heifers (Chicco & Godoy, 2005). In beef cattle production, these animals must be mating at 2-year-old, however, under nutritional deficit conditions, growth is negatively affected and also the reproductive performance during the breeding season (Moriel et al., 2020). Therefore, low average daily gain delays puberty, increasing the age at first mating. In most of the country's farms, replacement heifers have the first mating at 3-year-old (Camaripano, 2013), however, with an improved fed management, only 18-20 % of these (Depablos et al., 2009).
Strategic supplementation in its different forms: energetic, protein, and mineral, has been considered an option to correct the nutrient deficiencies of the pastures and minimize the periods of undernutrition that the animals go through, as well as to increase the economic productivity of the livestock system. Chicco and Godoy (2005) suggested the use of low rumen load supplements, defined by these authors, as supplements with nutrient supply with a catalytic effect that maximize ruminal fermentation (greater degradation of organic matter and NDF, and higher production of volatile fatty acids, ammonia nitrogen, and microbial protein), taking up less space in this compartment to allow for greater forage intake. Additionally, low rumen load supplements including rumen-undegradable protein sources (hydrolyzed feather meal, fish meal, blood meal) may increase the flow of essential amino acids to the small intestine (Scholljegerdes et al., 2005).
Another supplementation option are calcium salts of long-chain fatty acids (CaLCFA), which like other fats increase diet energy density, besides minimize the negative effects of unsaturated fats on rumen fermentation and digestion (Palmquist & Jenkins, 2017), and because they are protected from biohydrogenation in this compartment, the absorption in the small intestine of essential polyunsaturated fatty acids is increased (Purushothaman et al., 2008). Additionally, supplementation with CaLCFA improves the reproduction in cattle, associated with an increase in the concentration of cholesterol and progesterone (Sklan et al., 1991), as well as a greater number of larger follicles (Lucy et al., 1991), since cholesterol-LCFA esters are a critical component of follicular steroidogenesis associated with the maturation of dominant follicles (Gwynne & Strauss III, 1982).
For these reasons, the objective of this research was to evaluate the effect of supplementation with CaLCFA and CaLCFA + protein, using hydrolyzed feather meal as the main source of rumenundegradable protein, on body weight (BW) changes, reproductive variables, and some components of blood chemistry of Brahman replacement heifers grazing in the western Llanos of Venezuela, as well as evaluating quantity and quality of forage along the experimental period.
Materials and methods
Experiment management
The experiment was carried out in the western Llanos of Venezuela at the “Agropecuaria El Caibón S/C”, located at 07°22′29″N and 71°10′13″W, Santa Cruz de Guacas, Barinas State. The weather of this region is classified as tropical savanna (Peel et al., 2007), with an annual average pluviometry of 1,800 to 2,400 mm during the rainy season from April to December, with dry periods between January and March (Marvéz, 2003). The experiment was initiated in November (end of the rainy season) and ended in February (dry season) for a duration of 102 d. The historical average monthly rainfall in the region for November, December, January, and February was 59, 28, 12, and 9 mm, respectively. The historical record included rainfall between the years 2011-2017 according to (Instituto Nacional de Meteorología e Hidrología [INAMEH], 2020), and between 2019-2021 recorded on-farm. The farm has soils with silty clay loam texture; pH of 4.6-5.8 and organic matter content of 1.80-5.70 %.
Table 1. Dry matter (DM) content and fatty acids profile of CaLCFA supplement
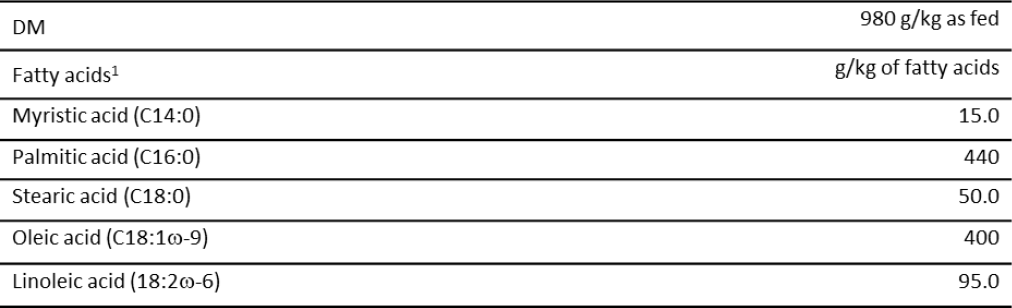
Note:1Made with plant-based oils from Elaeis guineensis Jacq (Oil palm). Assurance levels provided by the manufacturer.
Source: Elaborated by the authors
Fifty-seven Brahman replacement heifers with 309.1 ± 3.04 kg BW (mean+ se) and 1,010 ± 8.51 d of age (or 33.2 ± 0.28 months), were divided in three BW uniform groups, and assigned to three treatments: 1) grazing forage only (F); 2) F + CaLCFA (table 1); and 3) F + CaLCFA + protein-energy mix, with 453 g CP/kg DM (CaLCFA+CP, table 2). The supplements were offered in quantities of 200 g/animal/d and 1 kg/animal/d, for CaLCFA and CaLCFA+CP, respectively. Daily, the two supplemented groups were taken up and each heifer was supplemented individually between 6:30 and 7:00 hours. In the paddocks, all animals had free access to 50 g/animal/d of a mineral mix (250 and 85 g/kg of Ca and P, respectively), and water from a natural source. The supplements (CaLCFA and CaLCFA+CP) offered and orts were weighed daily with a portable weight (Pocket Balance, Germany). The supplements intake was calculated by subtracting their amount in the orts from the daily amounts offered.
The animals were kept in a rotational system (63 ha) with 12 paddocks (5.25 ha), 9 d in and 27 d out of them, in pastures with Urochloa arrecta (Hack. ex T. Durand & Schinz) Morrone & Zuloaga mainly, and U. humidicola (Rendle) Morrone & Zuloaga (Poaceae) and Leersia hexandra Swartz, with an average stocking rate of 0.76 AU/ha. Animals were kept in three paddocks for 9 d and then rotated in the same paddocks every 3 d to minimize the pasture effect. The supplementation started 57 d before the beginning of the breeding period, the latter lasting 45 d. Heifers were bred by artificial insemination using Holstein’s sexed semen at the first insemination and Holstein’s non-sexed semen at the second one.
Table 2. Supplement composition
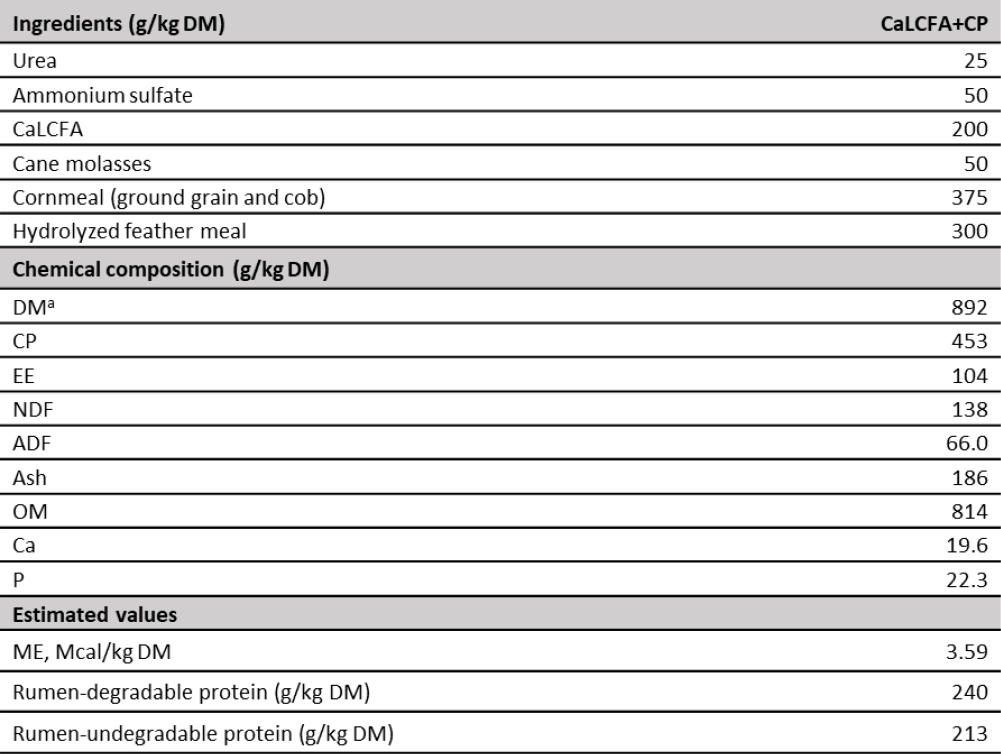
Note:ag/kg as fed. CaLCFA: calcium salts of long-chain fatty acids. DM: dry matter. CP: crude protein. EE: ether extract. NDF: neutral detergent fiber. ADF: acid detergent fiber. OM: organic matter. Ca: calcium. P: phosphorus. ME: metabolizable energy.
Source: Elaborated by the authors
The breeding period started in January (the beginning of the dry season). During the latter, before supplementation (6:00 h), heifers were grouped into three pens (one pen/treatment) and observed for 30 min for heat detection, using one teaser bull per group. The same heat observation procedure was repeated at 16:30 h.
Forage sampling
Systematic forage samplings were taken considering the spatial and botanical variability of the pasture. Six forage samples per paddock were taken every 9 d (when starting the grazing of a group of three paddocks), for a total of n = 198 samples. The samples were collected using a metal frame (Paladines, 1992) of 0.375 m2 for a harvest area of 6.75 m2/d. Forage was harvested clipping at 10 cm above ground level. Canopy height within the sampling area was measured twice per frame using a graduated ruler (precision of 1 cm), and canopy cover was expressed as the percentage of soil covered by forage. To determine biomass, forage samples were pre-dried in a forced-air oven (Thelco®, Model 6M, U.S.A.) at 60 °C until constant weight according to the Association of Official Analytical Chemists (AOAC, 2016). Sixty-six composite samples were made, two per paddock. Each sample was divided into two fractions for structural and chemical analysis. For the former, according to Chacón et al. (1977), green, dead, leaf and stem percentages, green:dead ratio and leaf:stem ratio were calculated.
For chemical analysis, the remaining fraction was ground (Thomas Wiley®, Model 4, U.S.A) in 1 mm sieve, and was analyzed according to AOAC (2016) for DM by gravimetric estimation at 105 °C, CP by Kjeldahl method (N × 6.25) and ash by ignition at 550 °C. The NDF was performed according to Van Soest and Wine (1967) without sodium sulfite, and Ca and P were determined by atomic absorption spectrophotometry (AOAC, 2016) and colorimetric procedure (Fiske & Subbarow, 1925), respectively.
Chemical analysis of CaLCFA+CP supplement was performed. Dry matter, CP, ash Ca and P were determined by the same methods described for forage samples, ether extract by reflux with hot petroleum ether (AOAC, 2016), and NDF and acid detergent fiber according to McQueen and Nicholson (1979) and Van Soest (1973), respectively (table 2). Supplement metabolizable energy was calculated based on theoretical values of cane molasses according to National Research Council (NRC, 2000) and CaLCFA from oil palm according to De Blas et al., (2010); cornmeal (Zea mays, ground grain and cob), and hydrolyzed feather meal according to in vitro DM true digestibility reported by Mora et al., (2015), transformed into digestible energy according to Heaney and Pigden (1963) and subsequently to metabolizable energy according to NRC (2000). Rumen-degradable and undegradable proteins of the supplements were estimated according to the values reported by NRC (2000) for each ingredient.
Animal sampling
Animal BW was measured (Iderna®, Capacity 3,000 kg, Venezuela) at 0, 28, 56, 84 and 102 days of the experimental period (one weighing per animal per day), with no access to grazing and water 16 h before weighing. The average daily gain (ADG) in each period was calculated.
Blood samples were taken by jugular puncture from six animals per treatment randomly selected at the beginning of the experiment. The same animals were sampled at the same time when BW was determined. The samples were collected with 21G×1” needles (BD Vacutainer®, USA) and deposited in tubes Vacuum Diagnostics® (6 mL, China) without anticoagulant. Blood samples were centrifuged (IEC Centrifuges®, Model Clinical, U.S.A.) at 915 × g for 15 min, and serum was kept at -20 °C (Bosch® Model GSD32, Germany) to determine glucose (Trinder, 1969) and cholesterol (Allain et al., 1974) by enzymatic-colorimetric procedure, and urea by enzymatic kinetic (Tiffany et al., 1972). Wiener lab kits (Wiener lab®, Argentina) were used to determine glucose and cholesterol concentrations, while Invelab kit (INVELAB S.A.®, Venezuela) was used for urea determination. Absorbance readings were taken on a Microlab 200 spectrophotometer (Lab Wiener 2000, Germany) at a wavelength of 505 nm for glucose and cholesterol, and 340 nm for urea, according to the kit instructions.
Animals were palpated transrectally (Bekele et al., 2016) at 0, 28, 56 d of the experimental period, to detect the presence of ovarian structures (follicles and corpus luteum). Pregnancy was detected by transrectal palpation, at 45 d after the end of the breeding period (Bekele et al., 2016). The number of animals born from the animals of each treatment was recorded during the calving period.
Statistical analyses
Forage variables data were grouped in periods (0-28, 29-56, 57-84, and 85-102 d of experiment) and treated by ANOVA according to a complete randomized design, using the GLM procedure of SAS® (University Edition). The statistical model was Yij = µ + Pi +Eij, where Yij is a dependent variable, µ is the mean for all observations, Pi is the effect of period i, and Eij is a random error (Montgomery, 2017). The green:dead and leaf:stem ratios, as well as Ca were transformed to neperian logarithm (Ott & Longnecker, 2010). Differences among periods were evaluated using the Tukey-Kramer procedure for unbalanced data (Montgomery, 2017).
Data for BW, ADG and blood chemistry were treated by ANOVA as a complete randomized design, with measurement repeated using the MIXED procedure of SAS® according to the following statistical model: Yijk = µ + Di + Aij +Tk + (D×T)ik + Eijk, where Yijk is the response at time k on animal j in treatment i, µ is the overall mean, Di is the fixed effect of treatment i, Aij is the random effect of animal j in the treatment i, Tk is the fixed effect of time k, (D×T)ik is the fixed interaction effect of treatment i with the time k, and E ijk is a random error at time k on animal j in treatment i (Littell et al., 1998). Differences among treatments were evaluated using Tukey’s test (Montgomery, 2017). When the interaction Treatment × Time was significant the PLM procedure of SAS® and Tukey’s test were used. The presence of ovarian structures, pregnancy and calving were considered binary variables and were analyzed by logistic regression (Ott & Longnecker, 2010) using the GENMOD procedure of SAS®. The results were expressed as a percentage.
Results and discussion
Biomass and structural and chemical análisis
The amount of biomass in the paddocks (table 3) showed variations during the experimental time with lower values corresponding to periods 0-28 d (2,402 kg DM/ha) and 84-102 d (2,249 kg DM/ha). The lower value of biomass at the beginning of the experiment may be to the fact that the pastures were grazed by other animals prior to the experiment with a greater stocking rate. However, biomass gradually increased (10 %) and by the end of the experiment diminished (23.2 %) due to limited rainfall. In all cases biomass was always higher than 2,000 kg DM/ha, the value suggested by Minson (1990) as sufficient for an adequate intake under grazing conditions. Similar average values of U. arrecta biomass (2,264 kg DM/ha; harvested at 28 days of age) were observed by Homen et al., (2010) in Venezuela between November and February months, end of the rainy season and dry season, respectively. Nevertheless, at the end of the experiment, biomass was lower than 30 kg of DM/AU/d suggested by Lamela (1992) as the adequate amount. This decrease was probably due to limited rainfall as was observed by Herrera et al., (2017) in the dry season in western Llanos of Barinas State.
Table 3. Biomass and structural analysis of forage
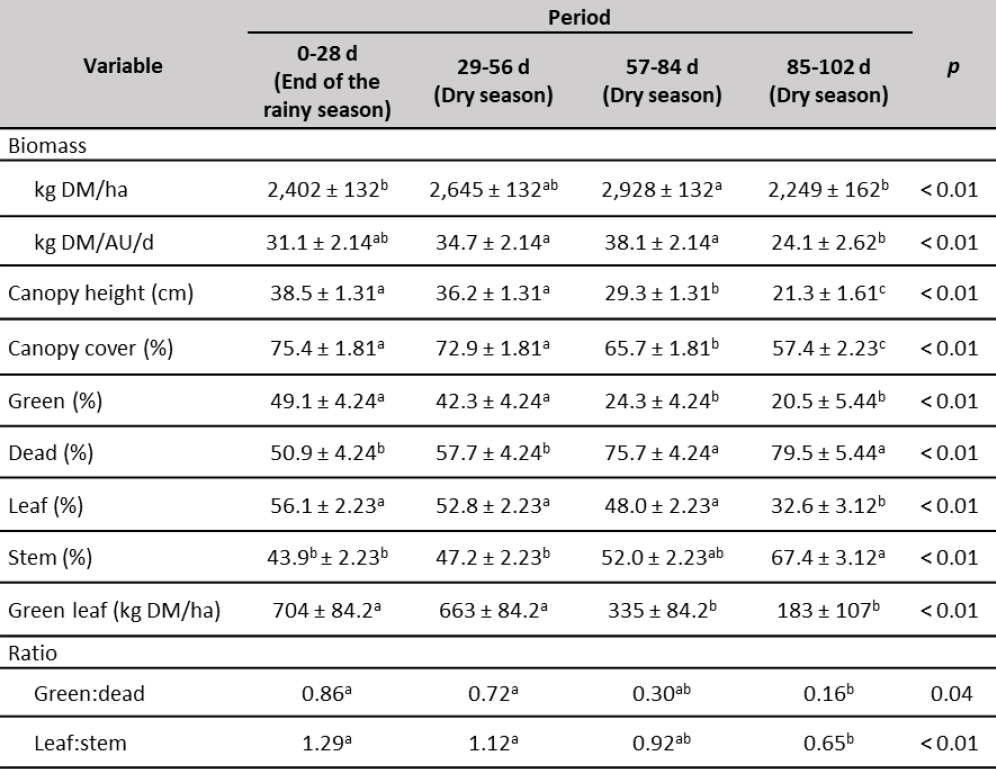
Note:a, b, c Means with different superscripts in the same row are different (p < 0.05).
Source: Elaborated by the authors
Canopy height and canopy cover (table 3) decreased along the experimental period, possibly due to the decrease in rainfall, during that time of year, characteristic of the region. Growth inhibition under drought is a typical defense reaction among plants. Water deficit in the soil leads primarily to the reduction of the water potential of plant shoots and the stimulation of the growth of the root system (Staniak, 2016). The greater canopy height observed until day 56 of the experiment (> 36.7 cm) could have favored a greater DM intake (Paula et al., 2012), due to an increase in the size of the bite (Allden & Whittaker, 1970). From day 56, the canopy height began to decrease, which could negatively affect the DM intake.
The green and leaf material percentages, as well as the green:dead and leaf:stem ratios (table 3) decreased along the experiment, which can negatively affect the DM intake since the animals prefer to eat the green and leaf fractions of the forage. Once the grazing animal is accustomed to eating the leaf fraction, it appears to continue to search out the leaf even when very little is present, and this can lead to very low forage intake (Minson, 1983). Additionally, the animal prefers to restrict the forage intake rather than increase the stem consumption (Minson, 1982), this explains the increase of the stem fraction during the experimental period. The amount of green leaf per hectare followed the same behavior as the percentage of green material and was always less than 1,000 kg DM/ha, which may have a negative effect on the leaf selection, and consequently, the organic matter intake by the animal decreases (Chacon & Stobbs, 1976).
Crude protein and NDF of forage (table 4) decreased throughout the experimental time. In the first three periods, CP was higher than 700 g/kg DM, which is the lower limit to sustain microbial growth and support efficient fibrous carbohydrate digestion in rumen (Lazzarini et al., 2009), however, at the end of the experiment (85-102 d) dropped to 616 g/kg DM, this might have a negative effect on forage intake (Milford & Minson, 1966). The decrease of CP concentration, probably was due to the leaf fraction decrease, since CP content in the leaves is greater than stems (Queiroz et al., 2012). In addition, CP concentration in plants is the result of nitrogen uptake which is greatly determined by water availability (Allahdadi & Bahreininejad, 2019). Crude protein forage content was adequate to heifer requirements to have a minimum ADG of 291 g/d (NRC, 2000) only during the first 56 d of the experiment.
On the other hand, NDF of forage was within the range reported in tropical grasses (675-759 g/kg DM; Lima et al., 2018; Mlay et al., 2006) and showed the highest values at the end of the rainy season (721 g/kg DM), and gradually decreased until reaching 666 g/kg DM at the end of the experiment (dry season). The calcium content of forage decreased along of experimental period, while P was not influenced (table 4). Calcium was greater than P content, which was opposite to observed from other authors in forages of the western Llanos of Venezuela (Herrera et al., 2017; Mora et al., 2014; Parada & Mora, 2019). Both mineral elements showed adequate concentrations to satisfy the requirements of replacement heifers (NRC, 2000).
Table 4. Forage chemical composition during the experiment
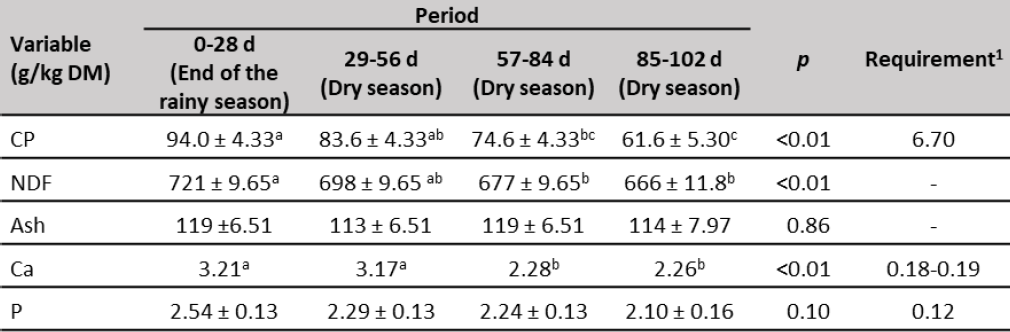
Note:CP: crude protein. NDF: neutral detergent fiber. Ca: calcium. P: phosphorus. a, b, c Means with different superscripts in the same row are different (p < 0.01). 1309-430 kg BW replacement heifers and ADG of 291 g/d (NRC, 2000).
Source: Elaborated by the authors
The intake of CaLCFA supplement was complete (200 g/animal/d), while the average intake of CaLCFA+CP supplement was 192 g/animal/d. Although the low consumption of the CaLCFA+CP supplement may be associated with the low palatability of hydrolyzed feather meal (Aderibigbe & Church, 1983). Recent research using 180-360 g/animal/d of this ingredient does not report rejection or decrease in its consumption (Mora et al., 2014, 2015; Ngu et al., 2019).
Body weight and average daily gain
Heifers BW showed an interaction treatment × time (figure 1), being similar at the beginning of the experiment (309.1 ± 3.04 kg), however, at day 28 superiority in CaLCFA of 4.5 % and 3.5 % was observed over F and CaLCFA+CP, respectively. At the beginning of the breeding season (day 56), the superiority of CaLCFA was maintained, with BW 2.28 % greater than the other two treatments, which presented similar weights among them. At the end of the experiment the three treatments showed different BW, being CaLCFA greater than CaLCFA+CP (2.96 %) and F (5.15 %), and CaLCFA+CP greater than F (2.11 %), this latter lost BW in the last period of the experiment. Body weights at the beginning of the breeding season were 328, 336 and 329 kg for F, CaLCFA and CaLCFA+CP, respectively. These values are lower than 340 kg, suggested as adequate by Camaripano (2013) for mating and to ensure a higher pregnancy rate in Brahman heifers, BW that was only reached by CaLCFA along the breeding season.
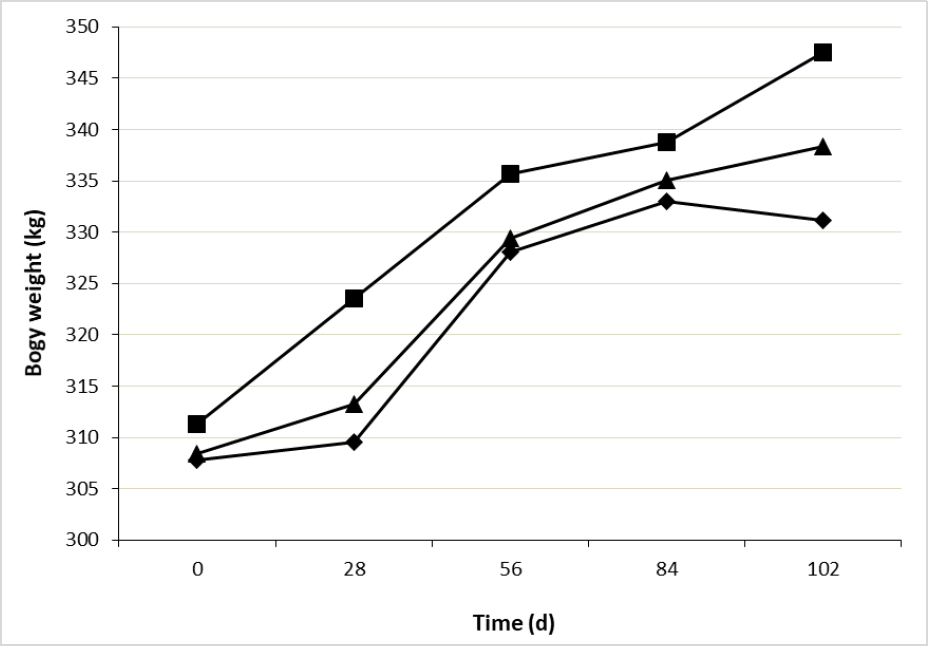
Source: Elaborated by the authors
Figure 1. Effect of the interaction treatment × time (p < 0.01) on body weight of Brahman replacement heifers. F (¨), CaLCFA (■), CaLCFA+CP (▲).
On the other hand, ADG was influenced by treatments, with greater gains (g/d) for CaLCFA (358) in relation to CaLCFA+CP (281), and lower for F (206) compared to the former two treatments (table 5). The better response when using CaLCFA, possibly is the conjunction with forage feeding. When ruminants are fed with a forage-based diet, the amount of ruminal acetate produced is greater (Kozloski, 2019), and CaLCFA supplementation also increases ruminal acetate (Behan et al., 2019). This latter is the most important source of metabolizable energy through its oxidation and is also the main substrate for lipogenesis (Kozloski, 2019). When formaldehyde-treated lipid supplements are added to the diet, there was a reduction in the partitioning of acetate towards lipogenesis (Yang et al., 1978), therefore increase the availability of acetate for oxidative purposes (Van Houtert et al., 1990), this may help explain why supplementation with CaLCFA improves ADG and BW.
Table 5. Average daily gain (ADG) and blood chemistry of Brahman replacement heifers supplemented with CaLCFA and CaLCFA+CP
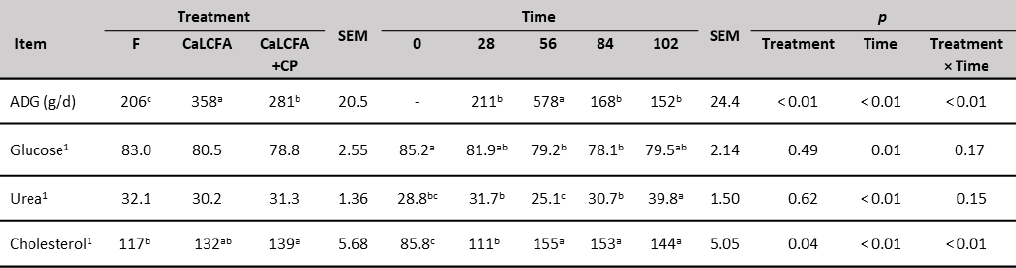
Note:1mg/dL. a, b, c Means with different superscripts in the same row are different (p < 0.01). SEM: Standard error of the mean.
Source: Elaborated by the authors
With the use of CaLCFA and a bypass protein meal of 438 g CP/kg DM (formaldehyde-treated cottonseed meal) in heifers, Van Houtert et al. (1990) observed that when adding CaLCFA (100; 200 o 300 g/animal/d) to 300 g of bypass protein meal, the ADG increased compared to the heifer's group that did not receive CaLCFA (372 vs. 419 g/d), and this response was maximized when 900 g of bypass protein meal were added (469 vs. 616 g/d). This greater ADG response was not observed in the present experiment when CaLCFA+CP was used, possibly due to the low consumption of this supplement (192 g/animal/d), which also implied an average CaLCFA intake of 34.3 g/animal/d and 51.4 g/animal/d of hydrolyzed feather meal.
The greater ADG of CaLCFA+CP compared to F, may be due to the CP and rumen-undegradable protein intake through a supplement, which was estimated at 77.6 and 36 g/animal/d, respectively. Sawyer et al. (2012) observed that a low intake of CP as 40 g/animal/d of CP with 30 g of rumenundegradable protein content (blood and feather meal mix), can replace 160 g CP/animal/d of cottonseed meal supplement (88 g rumen-degradable protein) and maintain ruminal function. Sawyer et al. (2012) indicated that protein sources greater in rumen-undegradable protein may improve CP use efficiency when fed at lesser amounts by minimizing ruminal and metabolic N losses and maintaining NDF fermentation. In addition, Mora et al. (2015) observed that supplementation with hydrolyzed feather meal led a ruminal environment of volatile fatty acids and organic matter degradation of forage similar to that produced when soybean meal supplement was used, and superior to the non-supplemented group.
Average daily gain showed treatment × time interaction (table 5 and figure 2) with more persistent values greater than 400 g/d when animals were supplemented with CaLCFA, during 3 of 4 evaluations of ADG.
In the period from 29 to 56 d, CaLCFA+CP and F showed an ADG greater than CaLCFA, however, it was not enough to reach the BW of CaLCFA, due to greater ADG of the latter, in the previous evaluation period.
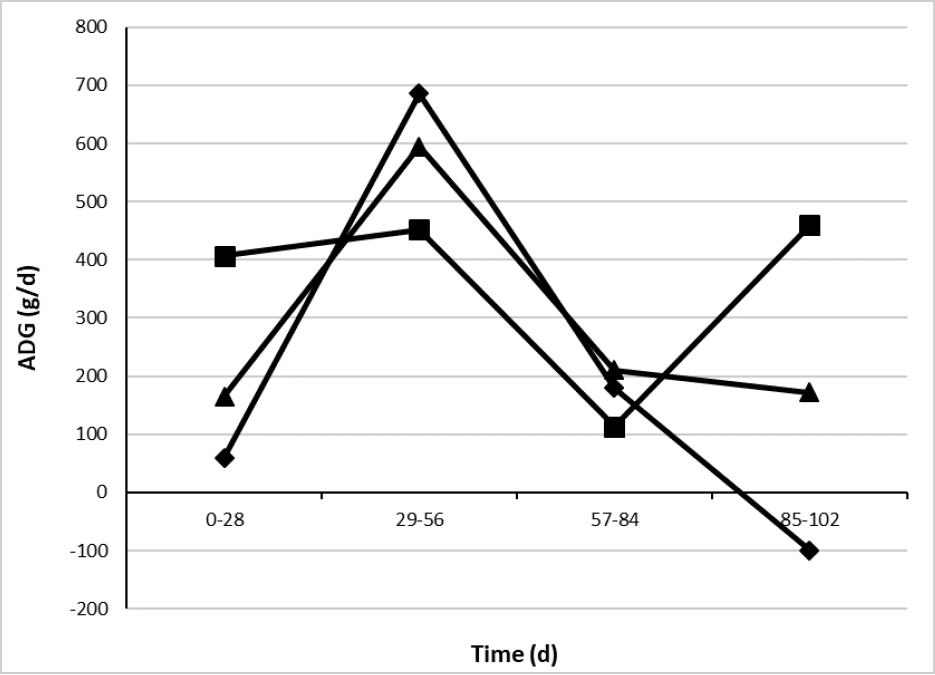
Source: Elaborated by the authors
Figure 2. Effect of the interaction treatment × time (p < 0.01) on average daily gain (ADG) of Brahman replacement heifers. F (¨), CaLCFA (■), CaLCFA+CP (▲).
Along 57-84 d period, all treatments showed ADG with no difference and decreased compared to the previous period, particularly CaLCFA showed its lower ADG along the experimental period. It was possibly due to the beginning of the breeding period at 56 days, the animals were kept in the pens twice a day for heat detection, which could have a negative influence on the dry matter intake of forage, and therefore on the ADG, since they were not adapted to this management.
In the final period of the experiment (85-102 d), despite the decrease in the forage quality (CP, NDF, green, dead, leaf, stem) as well as in the quantity (biomass, canopy height, canopy cover), the CaLCFA supplemented group showed greater ADG, followed by CaLCFA+CP, and BW losses in CONTROL. Probably the supplements were able to compensate for the decrease in the quality and quantity of the pasture.
Blood chemistry
Serum blood glucose concentration (table 5) was no affected by the treatments, however, was affected by the factor time, being highest at the beginning of the experiment, and greater than the critical value of 40 mg/dL (González, 2000) along the experiment. This result is opposite from Cappellozza et al. (2014) using Angus × Hereford heifers with 226 d of age and 200 kg BW, they observed greater glucose concentration on an animal with protein (65.1 mg/dL) and energetic supplementation (65.0 mg/dL) vs. the no supplemented group (59.3 mg/dL). Nevertheless, in tropical conditions, supplementation with protein sources did not affect glucose concentrations in Brahman first-calf heifers under grazing conditions (Mora et al., 2014), nor crossbred cows in confinement conditions (Mora et al., 2015).
Serum blood urea concentration (table 5) was not influenced by treatments, being these values within the reported reference (15-42 mg/dL), which indicates that there was no low protein intake, nor a high intake of rumen-degradable protein (Wittwer, 2000). Similar results were reported by (Mora et al., 2014) in grazing animals and supplemented with 200 and 420 g CP/d.
The factor time influenced urea concentration, with lower values during the first 84 d of the experiment, and higher at day 102 (39.7 mg/dL). This increase may be due to the decrease in forage biomass (quantity, height, green, leaf) and therefore possible lower consumption of structural carbohydrates during this stage of the experiment, since a decrease in energy intake inversely influences the concentration of ruminal ammonium due to a reduction in the microbial protein synthesis, increasing the concentration of blood urea (Wittwer, 2000). A similar increase in urea concentration (in milk) was observed by Herrera et al. (2017), when biomass was lower than 2,000 kg DM/ha.
On the other hand, serum cholesterol was influenced by supplementation type (table 5) with higher concentrations for CaLCFA+CP (139 mg/dL) and lower for F (117 mg/dL). This result was unexpected, due to the low consumption of CaLCFA (34.3 g/animal/d) through the CaLCFA+CP supplement. The results are contrary to those observed by different authors (Espinoza et al., 2010; Long et al., 2014), who observed higher concentrations of blood cholesterol when the animals were supplemented with CaLCFA (100 to 200 g/animal/d). Previously was described the reduction in the partitioning of acetate towards lipogenesis when bypass fat was used, and greater acetate would be available for oxidative purposes. Therefore, lesser acetyl-coenzyme A could be used for cholesterol synthesis, which begins with the condensation of two acetyl-coenzyme A molecules (Nelson & Cox, 2014). This can explain partially the lack of response in the cholesterol concentration for CaLCFA. The average cholesterol concentrations along the experimental time, in the three treatments were within the normal range 66-166 mg/dL (Wittwer, 2000).
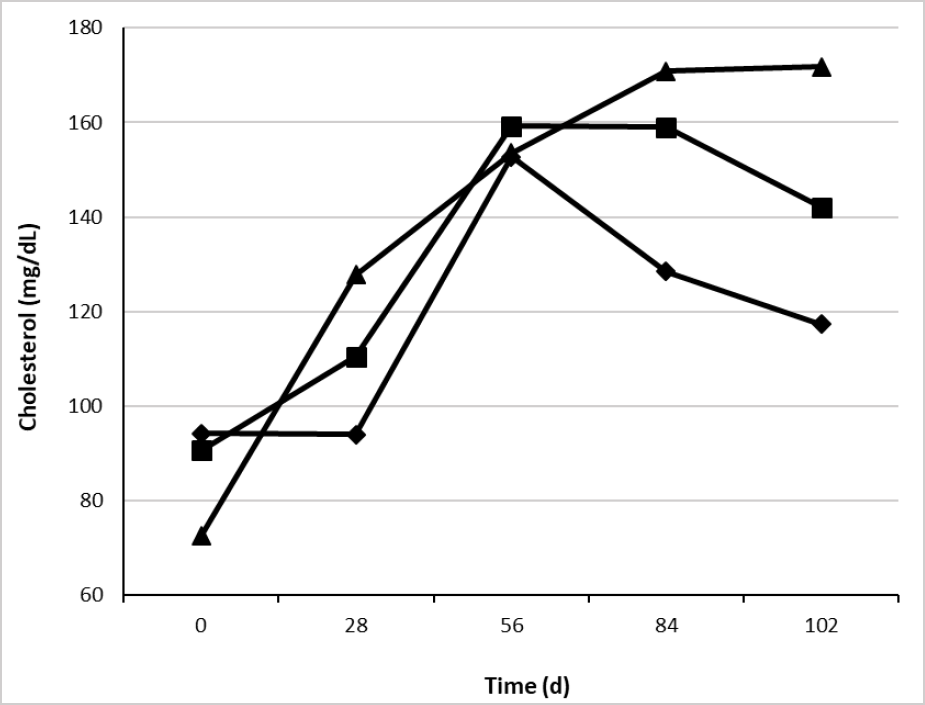
Source: Elaborated by the authors
Figure 3. Effect of the interaction treatment × time (p < 0.01) on serum blood cholesterol concentration of Brahman replacement heifers. F (¨), CaLCFA (■), CaLCFA+CP (▲).
Serum cholesterol showed an interaction treatment × time (table 5 and figure 3), being lower in CaLCFA+CP at day 0, and increasing progressively, with higher concentrations after the breeding period started (84 and 102 d), and slightly above the normal range, while in F group, cholesterol concentrations decreased in the same period. The decrease in the forage quality and the decrease in the F group of ADG and cholesterol concentration, allows us to infer that there was a decrease in the energy provide since the decrease in cholesterol concentration indicates a low energy intake in the diet (Wittwer, 2000).
Reproductive variables
The percentage of animals with follicle and corpus luteum (table 6) at day 0; 28 and 56 was similar among treatments, while at day 28, the percentage of animals with no ovarian structures was greater in CaLCFA (63.1 %) and lower in CaLCFA+CP (15.7 %).
Pregnancy rate at the first insemination (table 6) tended (p = 0.07) to be greater in CaLCFA+CP and lower in CaLCFA and F. Pregnancy rate corresponding to the second insemination was similar among treatments. The low number of animals at the second insemination could be limiting to detect differences among treatments (Ferguson & Chalupa, 1989).
The overall pregnancy rate (table 6) was greater in CaLCFA+CP and lower in F. The improvement on the reproductive performance in the CaLCFA+CP supplemented group, may be due to the contribution of the amino acids glutamate and aspartate, that are found in high concentrations in hydrolyzed feather meal (Divakala et al., 2009; Pacheco et al., 2016). Glutamate and aspartate are considered excitatory amino acids and are an essential component of the neuroendocrine transmission line that regulates anterior pituitary luteinizing hormone and follicle-stimulating hormone secretion (Brann & Mahesh, 1994).
Table 6. Reproductive performance of Brahman replacement heifers supplemented with CaLCFA and CaLCFA+CP
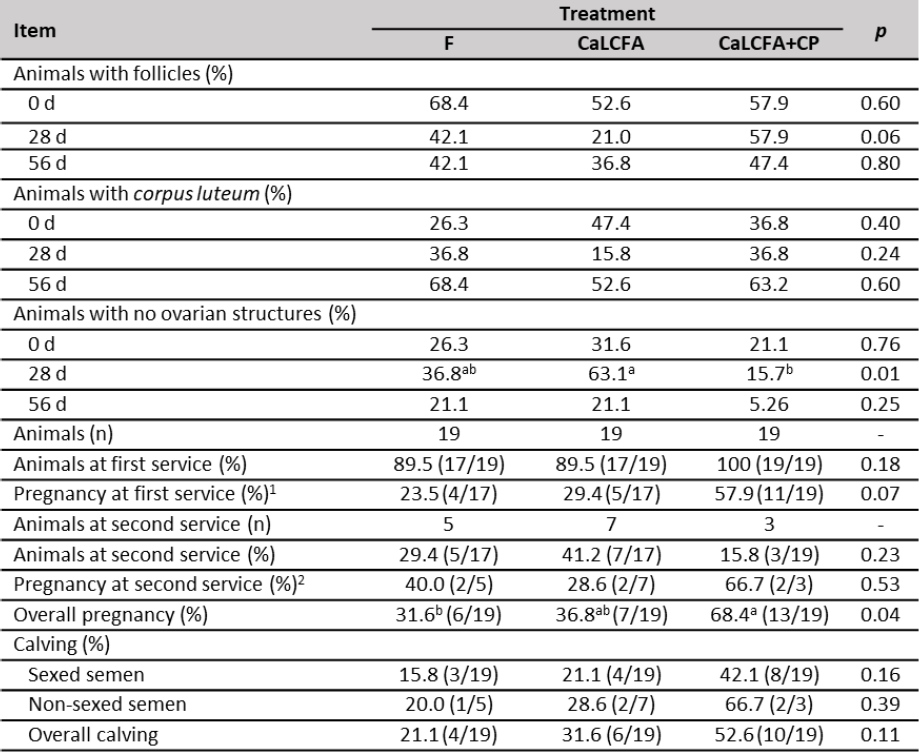
Note:a, b Means with different superscripts in the same row are different (p < 0.05). 1Sexed semen. 2Non-sexed semen.
Source: Elaborated by the authors
The increase in cholesterol concentrations in blood serum along the breeding period in CaLCFA+CP, also may be able to improve the pregnancy rate since cholesterol serves as a precursor for progesterone synthesis by ovarian luteal cells. Progesterone prepares the uterus for implantation of the embryo and also helps maintain pregnancy (Funston, 2004). The increase in progesterone concentrations has been associated with a better conception rate (Butler et al., 1996; Sklan et al., 1991).
The lack of response in the pregnancy rate for CaLCFA, despite having a greater BW at the beginning of the breeding period, may be due to a long supplementation period (102 d). Lammoglia et al. (2000) observed that supplementing replacement heifers with fat (Safflower seeds; Carthamus tinctorius L. cv. Centennial (Asteraceae) during 162 d, did not improve pregnancy rate, due to a dietary fat-reproduction antagonism. The authors suggested a feeding period with the fat of approximately 60 d before the beginning of the breeding period, due to the increase in backfat thickness changed dramatically after 56 d of supplementation, the value that remained until day 162. Increased body fat content has been associated with increased blood leptin concentration (Chilliard et al., 2005; Long et al., 2007), and the latter also increases in heifers due to the effect of CaLCFA supplementation (5 d/week, 276 and 200 g/animal/d) (Long et al., 2007, 2014, respectively) during 60 d before the beginning of the breeding period. In the ovaries, thecal cells have high-affinity receptors for leptin, leading to an inhibition of insulin-induced progesterone and androstenedione production, reducing, therefore, ovarian activity (Spicer & Francisco, 1998). In beef heifers, no difference was observed in the pregnancy rate among the control group and the saturated or unsaturated rumen bypass fat supplemented groups (Long et al., 2014).
The calving rate was not affected by treatments on both sexed and non-sexed semen inseminated animals, the same response was observed in the overall calving rate.
Conclusions
The decrease in biomass and forage structure (canopy height, canopy cover, green, leaf, green:dead and leaf:stem ratios) as well as in CP content, could affect negatively the forage intake by heifers. The Ca and P content in the forage were adequate to satisfy replacement heifer requirements.
Supplementation with CaLCFA and CaLCFA+CP improved BW and ADG of Brahman heifers at first mating, despite the low quantity and quality of forage at the end of the experiment. However, reproductive performance and blood serum cholesterol concentrations increased only with CaLCFA+CP supplementation, in spite of low consumption of the supplement.