INTRODUCTION
Microalgae are an important source of food for various aquatic organisms, which is due to a series of key features, including cell size, ease of reproduction, and the concentration and quality of their bio-compounds (Díaz et al., 2006; Martínez-Fernández et al., 2006). Within the microscopic marine ecosystem, there are diatoms (phylum Bacillariophyta), a group of microalgae that make up between 20 and 25% of species abundance in phytoplankton. Moreover, this group contributes with 30 to 60% of the proteins and between 5 and 20% of the lipids in the trophic diet, serving as an important food source for different growth stages in larvae, fish, crustaceans, and mollusks (Granum et al., 2002).
Likewise, most marine diatoms produce important natural bioactive compounds for different industries, such as the food, pharmaceutical, nutraceutical, and cosmetic sectors. They also produce medium- and long-chain polyunsaturated fatty acids such as eicosapentaenoic acid (EPA) and docosahexaenoic acid (DHA) (Baldisserotto et al., 2019).
On the other hand, due to their high growth rate, photosynthetic efficiency, and lipid content, they are primarily used as nutritional supplements in aquaculture and as additives in human nutrition (Bozart et al., 2009; Maeda, 2015). These derived metabolites possess antioxidant, anti-inflammatory, anticancer, antidiabetic, antiangiogenic, and antimicrobial properties (Bhattacharjya et al., 2020).
The central coast of Peru has a high phytoplankton production, which is important for fishing activities, as it constitutes the main source of nutrition in the first link of the food chain (Ochoa and Tarazona, 2003). Among the species widely distributed along the coast of Peru are the diatoms of the genus Skeletonema and Chaetoceros (Delgado et al., 2019). Skeletonema costatum is a dominant species on the coasts of Peru, which represents 96% of the total phytoplankton, especially during winter, followed by several species of the genus Chaetoceros (Arellana et al., 2006). These genera are reported throughout the year and are associated with harmful algal blooms in major bays and fishing areas (Orozco et al., 2017; Baylón et al., 2019).
Many research studies on aquaculture catalogue diatoms as suitable food for the cultivation of organisms of commercial interest, e.g., in bivalves, for the early larval stages of crustaceans, and in some fish species, given their high protein content and EPA. Among the most prominent genera are Skeletonema, Phaeodactylum, Chaetoceros, and Thalassiosira (Hernández and Labbé, 2014; Velasco et al., 2016; Araújo et al., 2020).
Diatoms bioprospecting is important because it allows obtaining native strains that are adapted to the environment and have no ecological impact on it (Elyakoubi et al., 2020). Likewise, these new strains potentially have the nutritional profile required for aquaculture and for standing out over commercial strains. Currently, there are few studies on potential native strains for aquaculture nutrition in Peru.
Given the above, this study aimed at evaluating the nutritional profile of two diatom genera collected from the central coast of Peru (Ica and Callao), which corresponded to native strains from the Germplasm Bank of Aquatic Organisms (BGOA) of the Peruvian Sea Institute (IMARPE), in order to assess their potential as an ingredient for aquaculture.
MATERIALS AND METHODS
Study area
The samples were collected from the bays of Callao (12°02’34’’ S, 77°10’13’’ W) and Ica (13°52’0.01’’ S, 76°16’0.01’’ W) on the central coast of Peru. The selected strains were obtained, identified, and coded by the Germplasm Bank of Aquatic Organisms (BGOA), showing the following characteristics (Table 1):
Microalgal biomass production
A batch-type culture was carried out from 50 mL to 125 mL and maintained in a Binder climatic chamber (KBW 400, Germany) under the following conditions: f/2 culture medium (Guillard, 1975); pH: 7.5 to 8.5; temperature: 17 °C; lighting: 35 µmol.s-1.m-2; 12:12 hour photoperiod. After 12 days, scaling to 1 L and 7 L was carried out in triplicate, under the previous conditions, and with an aeration system using an aquarium pump. After 15 days of cultivation, the biomass was concentrated using a Hettich refrigerated centrifuge (Rotina, Germany) at 4500 rpm for 20 minutes at 10 °C. The wet biomass obtained was preserved at -20 °C. It was then dried using a Labconco lyophilizer (18 L, USA), according to the parameters described in the IMARPE manual (2020).
Nutritional profile
Moisture and ash tests were carried out according to Kolar’s methodology (1992), and lipid assays according to Folch’s methodology, with some modifications (Flores-Ramos et al. 2020). Proteins were quantified via the modified method of Lowry et al. (1951), and carbohydrates according to the phenol-sulfuric acid method of Dubois et al. (1956). The results were expressed as a percentage by weight (% w/w).
The extracted lipids were derivatized according to the methodology of Ichihara and Fukubayashi (2010). Subsequently, the fatty acid methyl esters were analyzed by gas chromatography using Varian equipment (CP-3800, USA) and a Restek, Stabilwax WCOT fused silica column of 30 m × 0.25 mm × 0.25 µm, employing a splitless injection method (0.50 minute), a 1 μL injection volume, an injector temperature of 250 °C, helium as the carrier gas at a flow rate of 1 mL/min, and a temperature ramp of 120 °C for one minute, 30 °C/min up to 160 °C, 160 °C for one minute, 4 °C/min up to 240 °C, and 240 °C for 7 minutes. The total time of the temperature program was 30.33 minutes, and the detector temperature was set at 260 °C. The results were expressed as a relative percentage.
The amino acid assay was carried out according to the instructions of the AccQ-Fluor reagent kit (Waters Corporation, Milford, MA, USA). The derivatized amino acids were injected into an HPLC Elite LaChrom (Hitachi High Technologies, Japan). The equipment conditions were as follows: Hypersil GOLD C18 column of 5 µm × 4.6 mm × 150 mm, column temperature: 37 °C, injection volume: 5 µL, mobile phase flow: 1 mL/min, excitation wavelength: 250 nm, emission wavelength: 395 nm, mobile phase A: sodium acetate, pH 5.1, mobile phase B: acetonitrile. The mobile phase ramp was as follows: 0 min (100.0), 2 min (100.0), 24 min (83.5, 16.5), 30 min (75.25), 30.1 min (100.0), and 38 min (100.0). Amino acids were quantified using a calibration curve from 5 to 50 pmol/µL. The results were expressed as a percentage by weight (% w/w).
Amino acid index
The amino acid score (AAS) was calculated according to the description provided by Peñaflorida (1989) and Oser (1959). The standard protein used corresponded to the essential amino acid requirements recommended for the species Pleuronectes ferruginea, Hippoglosus hippoglosus, and Paralichthys olivaceus, flatfish like the ‘flounder’ (Paralichthys adspersus) cultivated in our country (Kim and Lall, 2000), as well as to those for shrimp (Penaeus vannamei) (Xie et al., 2012) (Table 2). If the amino acid score was higher than 1, this value was replaced with 1. The essential amino acid index proposed by Peñaflorida (1989) is shown in Equation 1.
An EAAI value higher than 0.95 indicates a high quality of protein. If it is between 0.86 and 0.95, it is deemed to be of ‘good’ protein quality, and values between 0.75 and 0.86 correspond to a ‘usable’ protein quality. Finally, a value of less than 0.75 refers to an ‘inadequate’ protein (Oser, 1959).
Table 2 Amino acid requirements in different aquaculture species (% w/w). Concentrations adapted from Kim and Lall (2000) and Xie et al. (2012).
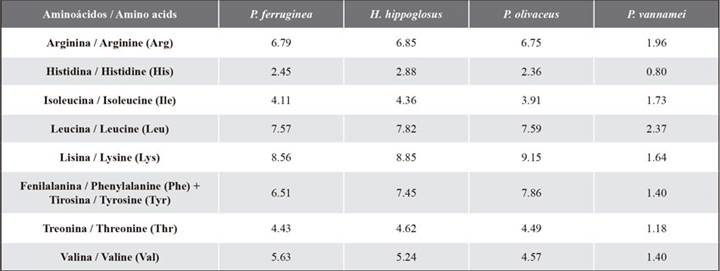
Statistical analysis
An analysis of variance (ANOVA) was conducted with a significance level of α = 0.05 and post hoc Tukey tests, in order to compare the averages of all assays regarding lipids, proteins, carbohydrates, ash, moisture, fatty acids, and amino acids. This was done via the Minitab 17 software (Pennsylvania, USA). Likewise, a heat map was used to determine the relationship between the microalgae strains and the fatty acids and amino acids using the R-Studio 1.2.5001 software (Boston, USA).
RESULTS
Nutritional profile
The highest percentage of proteins was obtained by strain IMP-BG-466 of the genus Skeletonema (30.4 ± 0.8 %), and the lowest percentage was from strain IMP-BG-056 of the genus Chaetoceros (12.7 ± 0.7 %) (Figure 1). The other strains of the genus Skeletonema did not show significant differences (p > 0.05).
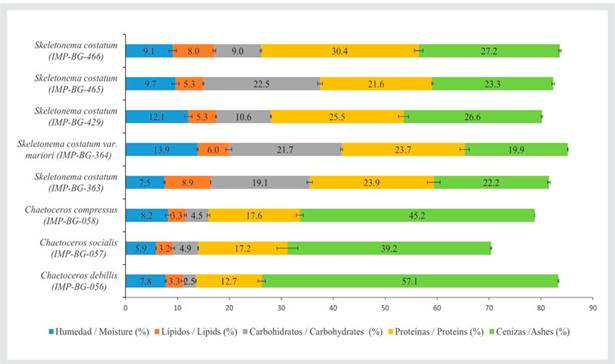
Figure 1 Proximate percentage corresponding to the biochemical profile of 8 different marine microalgae strains. Error bars indicate the standard deviation of the means.
Regarding the percentage of total lipids, strains IMP-BG-363 and IMP-BG-466 of the genus Skeletonema showed the highest percentages, i.e., 8.9 ± 0.0 % and 8.0 ± 0.2 %, respectively, with no significant differences between them (p > 0.05). The other strains of the genus Skeletonema did not show significant differences (p > 0.05). The strains of the genus Chaetoceros reported total lipids percentages of less than 3.5 % and did not show significant differences (p > 0.05).
The highest percentages of carbohydrates were found in the group of the genus Skeletonema (ranging from 9 to 23 %), while the strains of Chaetoceros did not exceed 5 %. Within the latter, strain IMP-BG-056 showed the lowest percentage (2.5 ± 0.1 %), while strains IMP-BG-057 and IMP-BG-058, corresponding to the species C. socialis and C. compressus, did not show significant differences (p > 0.05). Likewise, in the genus Skeletonema, only strains IMP-BG-364 and IMP-BG-465 reported a carbohydrate percentage higher than 20 %, and they did not show significant differences between each other (p > 0.05).
Regarding the percentage of ash, the strains of the genus Chaetoceros showed values higher than 39 %, with strain IMP-BG-056 (C. debilis) being the one with the highest percentage (57.1 ± 0.1 %). On the other hand, the strains of the genus Skeletonema showed ash percentages between 19 and 27 %.
The percentage of moisture in all samples was less than 14 %. The strains IMP-BG-364 and IMP-BG-429 from the genus Skeletonema showed the highest moisture percentage and exhibited no significant differences (p > 0.05). The strains IMP-BG-465, IMP-BG-466 (genus Skeletonema), IMP-BG-058, and IMP-BG-056, the latter two belonging to the genus Chaetoceros, showed no significant differences between each other (p > 0.05).
Fatty acid composition
In general, the fatty acids with the highest relative percentages were C14:0, C16:3n-4, and C20:5n-3 (EPA). The relative percentages of the fatty acid C14:0 ranged from 11 to 24 %, with significant differences between them (p < 0.05). Strains of the genus Skeletonema had the highest relative percentage of the acid C16:3n-4 (between 18 and 27 %), while strains of the genus Chaetoceros had the lowest percentages (between 0.6 and 4.8 %). Regarding EPA, the strain IMP-BG-056 of the species C. debilis showed the highest relative percentage (30.2 ± 0.9 %), and the strain IMP-BG-465, corresponding to S. costatum, had the lowest one (13.4 ± 0.0 %).
On the other hand, the highest DHA/EPA ratio was recorded for the Skeletonema strains, followed by the other species of Chaetoceros because the relative DHA percentages were less than 1 %. The percentages of ω3 ranged from 18 to 33 %, with EPA being the fatty acid that contributed the most in all strains, while the ω6 values were between 0.5 and 1.2 %. The strains of the genus Chaetoceros, IMP-BG-056 and IMP-BG-058, exceeded the ω3 / ω6 ratio > 50 (Table 3).
Table 3 Fatty acid composition of the evaluated strains expressed as the average of the relative percentage ± standard deviation.
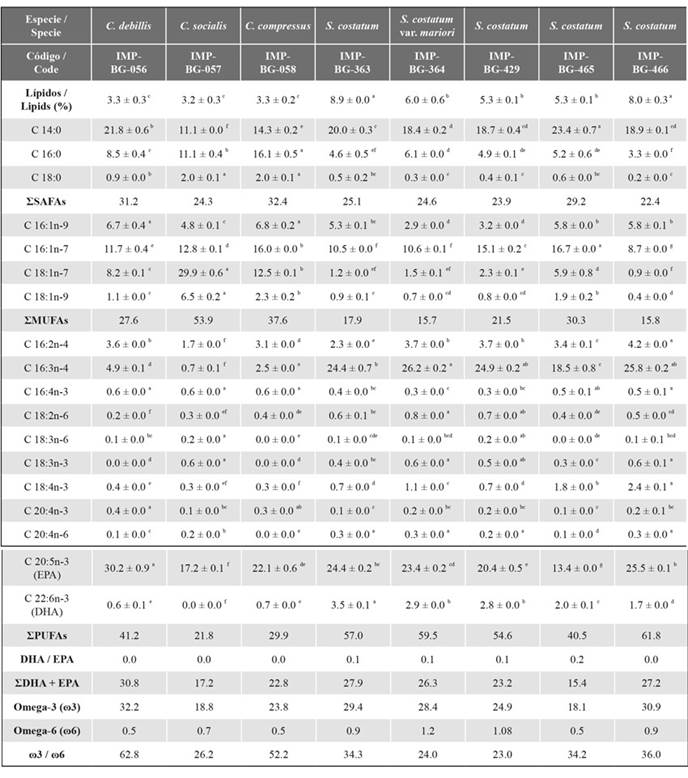
SAFAs: Saturated fatty acids, MUFAs: Monounsaturated fatty acids, PUFAs: Polyunsaturated fatty acids. Comparisons of the average relative fatty acid percentages by strain showed significant differences, as indicated by the letters a-g, according to the Tukey post hoc test.
In the heat map, the strains of the genus Skeletonema and Chaetoceros were grouped into different clusters due to differences in the relative percentage of fatty acids C 16:0, C 16:3n-4, and C 18:1n-7. The fatty acids were separated into two clusters: those with a higher relative percentage (C16:3n-4, C 14:0, and EPA) and the rest with a lower relative percentage (Figure 2).
Amino acid composition
Among the identified amino acids, glutamic acid, aspartic acid, leucine, and alanine had the highest concentration (each one ≥ 1 %). On the other hand, the amino acid with the lowest percentage in all strains was histidine (≤ 0.7 %). The strain IMP-BG-466 of the species S. costatum showed the highest percentage of amino acids (33.3 ± 0.2 %) (Table 4).
Table 4 Amino acid composition (% w/w) of the evaluated strains.
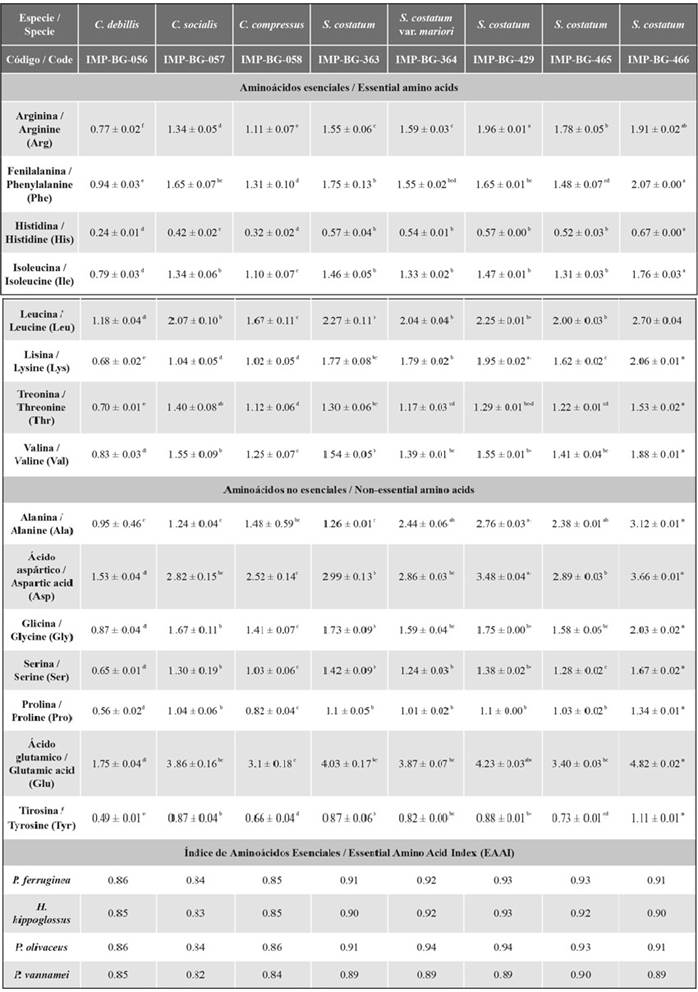
The groups that do not share the same letter have averages with statistically significant differences.
Regarding the EAAI, in general for flatfish and shrimp, the strains of the genus Skeletonema have a good protein quality, as the EAAIs were between 0.86 and 0.95. Table 4 shows that strains of the genus Chaetoceros possess a usable protein quality of protein (EAAIs ≤ 0.86). In addition, according to the amino acid score (data not shown) of both microalgae genera, for flatfish, the limiting amino acids were lysine (from 0.45 to 0.80) and histidine (from 0.61 to 1). In the case of the shrimp P. vannamei, the limiting amino acids were histidine (from 0.52 to 0.70) and arginine (from 0.73 to 0.94).
In the amino acid heat map (Figure 3), the strains were classified into two groups. The first cluster grouped most of the strains of the genus Skeletonema, and the second classified those of the genus Chaetoceros together with the strain IMP-BG-363 of the species S. costatum. These two clusters are separated by the difference in percentages of alanine and leucine. Likewise, the amino acids were separated into two clusters according to their percentage, one with a higher percentage by weight (aspartic acid and glutamic acid), and the other with the rest of the amino acids of a lower percentage.
DISCUSSION
Nutritional profile
In aquaculture, it is necessary to search for new protein sources that meet the requirements of each cultivable species. According to the FAO, the protein requirements in the diets of fish and shrimp range between 20 and 45 % (Tacón, 1987). This demand would be satisfied by the protein percentages analyzed in S. costatum (20 to 31 %), and these percentages are within the values reported in the literature (Brown and Jeffrey, 1995; Lestari et al., 2014; Van Houcke et al., 2017; Bastos et al., 2022). According to Vásquez-Suárez et al. (2010), these percentages could be increased to over 45 %. Within the genus Chaetoceros, the protein percentages of the strains analyzed (12 to 18 %), coincide with those reported by Medina-Reyna and Cordero-Esquivel (1998) and Pacheco-Vega and Sánchez-Saavedra (2009) for the C. mulleri strain (6 to 30 %).
In the culture of aquatic organisms, lipids are essential for the growth and survival of fish larvae (Kanazawa, 1983) and crustaceans (Pascual, 1984), with essential fatty acids of the omega-3 (ω3) and omega-6 (ω6) series being of the highest nutritional value. According to the FAO, the requirement for total lipids is 5-8 % of the diet (Leger et al., 1979; Tacón, 1987). This demand would be met by the analyzed strains of S. costatum (5 to 9 %), but not by the analyzed strains of the genus Chaetoceros (< 5 %). However, there are studies on S. costatum that report high levels of lipids, i.e., between 14 and 19 % (Brown and Jeffrey, 1995; Vásquez-Suárez, et al., 2010), while others report less than 2 % (Lestari et al., 2014). In the case of the genus Chaetoceros, the reports are varied: 11-23 % lipids in strains of C. calcitrans; 19-22 % in C. muelleri (Pacheco-Vega and Sánchez-Saavedra, 2009; Banerjee et al., 2011; Velasco et al., 2016); and only 7.6 % for the strain CCMP 3283 of C. socialis (d’Ippolito et al., 2015). The lower percentage of lipids in the genus Chaetoceros was possibly due to optimal culture conditions (Hu et al., 2008). Moreover, these strains have the potential to increase it up to 40 % under stress conditions due to nutrient deficiency and high light intensities (Rodolfi et al., 2009).
In aquaculture nutrition, contrary to proteins and lipids, carbohydrates do not have an absolute requirement percentage; they are a low-cost source of energy, substitute the conversion of proteins to energy, and are used in diet formulation as binders and flavor enhancers (Tacón, 1987). The carbohydrate percentages of the analyzed strains of S. costatum (9 and 23 %) did not exceed the percentages of Van Houcke et al. (2017), which were 23 to 25 % for strains of the same species, but they do contain the 18.3 % reported by Vásquez-Suárez et al. (2010). On the other hand, the carbohydrate percentages of the analyzed Chaetoceros strains (< 5 %) were similar to those reported by Brown and Jeffrey (1995) and Banerjee et al. (2011) in C. calcitrans, i.e., around 6 %. In contrast, Bastos et al. (2022) recorded values between 23 and 26 % for C. calcitrans, and values of 10 to 37 % were reported for C. mulleri according to Medina-Reyna and Cordero-Esquivel (1998), Pacheco-Vega and Sánchez-Saavedra (2009), and Velasco et al. (2016). This variation is possibly due to the fact that carbohydrates play a role in maintaining the cell wall, and it is likely that their availability changes according to laboratory conditions and growth phase (Schmid et al., 1981).
In aquaculture, the micronutrients present in ashes have very important functions, e.g., zinc, which prevent fish diseases (Shearer et al., 1992) or play a role in the growth of solid tissue such as exoskeletons of sponges and valves in mollusks and crustaceans (Simpson and Volcani, 1981; Welladsen et al., 2014). However, some studies have reported that a high ash concentration produce reductions in fish growth and effects on digestibility and water quality in the culture, so their percentage is minimized in the preparation of feed (Satoh et al., 1987). According to the nutritional requirements for fish diets, ash percentages should be between 3 and 12 %, as well as from 2 to 10 % for shrimps. However, there are supplies that increase this proportion up to 20 % of the diet, e.g., fishmeal (Faidutti, 1992; Moreno-Álvarez et al., 2000; García et al., 2004; Cruz et al., 2012). According to the results, the required percentage for fish and shrimp would be exceeded by more than double for the genus Skeletonema and more than triple for the genus Chaetoceros. According to Bastos et al. (2022), the ash percentage of S. costatum and C. calcitrans increases with respect to the concentration of silicates in the culture medium, up to 53 and 50 %, respectively. On the other hand, Medina-Reyna and Cordero-Esquivel (1998) and Pacheco-Vega and Sánchez-Saavedra (2009) achieved maximum values of 54 and 40 % in a strain of C. muelleri, respectively. Other studies mention that the increase is due to the nature of the siliceous frustules that form the cells (Paasche, 1973; Parsons et al., 1984; Cordero-Esquivel et al., 1993).
Fatty acid composition
Given the demand for new sustainable sources of fatty acids, the use of marine microalgae was proposed, as they are natural sources with a high relative percentage of polyunsaturated fatty acids. Research conducted by Seiliez et al. (2003) and Izquierdo (2005) concluded that, for the optimal growth of the fish Sparus aurata, the diet must contain a DHA/EPA ratio of at least 1.3 and a maximum of 2.5. The highest DHA/EPA ratio in this research was 0.15 for the strain IMP-BG-465 of the species S. costatum. Although it does not reach the required DHA/EPA ratio, it can be used in combination with other strains of microalgae with a higher relative percentage of DHA or with fish oil.
Skeletonema costatum is a species used in the aquaculture industry given its high relative percentage of EPA, which is important in the diet of many cultivable aquatic organisms (such as crustaceans and fish larvae) for their good development and survival (Koven et al., 2001; Pratiwy and Pratiwi, 2020). It is also an ideal species for mass cultivation due to its rapid growth and low nutrient requirements (Zafra-Trelles et al., 2017).
EPA was the fatty acid with the highest relative percentage in the diatoms analyzed. In the case of S. costatum, reports range from 6 to 24 % (Guihéneuf et al., 2008; Lestari, et al., 2014; Van Houcke et al., 2017; Gao et al., 2019 and Bastos et al., 2022). In the genus Chaetoceros, maximum values of 19 % were reported for C. calcitrans (Bastos et al., 2022), as well as 17 % for C. muelleri (Pacheco-Vega and Sánchez-Saavedra, 2009), which is less than the analyzed strain, i.e., C. debilis IMP-BG-056 (30.2 %).
A notable contribution of this research, as observed in the heat map, was the separation of the genera Skeletonema and Chaetoceros based on their fatty acid composition, recognizing some of them as possible chemotaxonomic markers, which is similar to that found by Hernández-Acevedo et al. (2019). One of these fatty acids, C 16:3n-4, is used by diatoms in the production of volatile aldehydes for the trophic regulation of zooplankton (d’Ippolito et al., 2004).
Amino acid composition
According to the amino acid scores, the limiting amino acids were lysine and histidine for flatfish and arginine and histidine for shrimp, which could affect various functions. In fish, lysine is related to growth, muscle tissue formation (Pineda-Quiroga, 2010) and reproductive cells (Hamid et al., 2016). On the other hand, histidine serves as an antioxidant and pH regulator in fish organs (Wade and Tucker, 1998; Munakata et al., 2000). In shrimp, arginine is a precursor to growth hormones (Wan et al., 2006) and regenerates ATP in muscles (Denis et al., 1998).
Regarding the EAAI, Okaichi (1974) reported indices for the diatoms Cyclotella sp., Skeletonema costatum, and Phaeodactylum tricornotum in the range of 0.66 to 0.76 for the fish Seriola quinqueradiata (yellowtail). The values reported in our research were higher for flatfish and shrimp, especially regarding the strains of the genus Skeletonema. However, comparisons are limited by the few references available, despite the fact that, in our results, both studied genera possess good and usable protein quality. On the other hand, according to Becker (2013), the amino acid composition is similar among microalgae species. However, in the heat map, a separation of two clusters was observed as a function of the species. Moreover, the strain IMP-BG-366 of the genus Skeletonema has an amino acid composition similar to that of the genus Chaetoceros.
CONCLUSIONS
The assessment of the nutritional profile of Peruvian diatom strains kept in the BGOA contributed to selecting the strain IMP-BG-466 of the genus Skeletonema as a potential ingredient for formulating feeds in aquaculture. This strain reported the highest percentage of protein (30.4 %) and was classified as having good and usable protein quality according to its EAAI for flatfish and shrimp, respectively. This, in addition to reporting the highest relative percentage of omega-3 fatty acids (31.0 %). However, this strain needs to decrease its high ash content (27.2 %), as well as to increase its low relative percentage of DHA (< 2 %) and limiting essential amino acids such as arginine, histidine, and lysine to be a feed that meets all the nutritional requirements of the tested aquatic organisms. We recommend evaluating this microalgae in ingestion and digestibility trials, safety tests, and the formulation of balanced diets with other food sources that complement its deficiencies