Services on Demand
Journal
Article
Indicators
-
Cited by SciELO
-
Access statistics
Related links
-
Cited by Google
-
Similars in SciELO
-
Similars in Google
Share
Ingeniería y competitividad
Print version ISSN 0123-3033
Ing. compet. vol.15 no.2 Cali July/Dec. 2013
Molecular adsorption model for organic compounds over TiO2 - P25 by protonic distribution affinity
Modelo de adsorción molecular para compuestos orgánicos sobre TiO2 - P25 mediante el método de afinidad protónica
Miguel A. Mueses
Photocatalysis and Solar Photoreactors Engineering, Chemical Engineering Program, Universidad de Cartagena, Colombia
E-mail: mmueses@unicartagena.edu.co
Fiderman Machuca-Martínez
School of Chemical Engineering, GAOX Research Group, Universidad del Valle, Campus Melendez, Cali, Colombia
E-mail: fiderman.machuca@correounivalle.edu.co
Eje temático: Chemical engineering / Ingeniería química
Recibido: 23 de Abril de 2013
Aceptado: 26 de Junio de 2013
Abstract
A predictive molecular adsorption model for organic compounds over TiO2-P25 particles was formulated. The model is an extension of a theoretical method of surface characterization called Protonic Distribution Affinity (PDA), which is obtained from potentiometric titration analysis, zero point charge (ZPC) of the material and adsorption isotherms of the Langmuir-Hinshelwood type. The chemical nature of the compound in the model was established by initial pH and molecular weigh. We found that, for the TiO2-P25, the ZPC was 6.8. The model was validated using experimental data of dichloroacetic acid (DCA), 4-chlorophenol (4-CP) and phenol (PH) to different conditions. The model has high predictive capacity, numerical stability and sturdiness for obtaining the solutions. For available experimental data, the correlation coefficient was 0.97.
Keywords: Photocatalysis, protonic distribution affinity, Langmuir-Hinshelwood isotherm, potentiometric titration.
Resumen
Un modelo predictivo para adsorción molecular de componentes orgánicos sobre partículas de TiO2-P25, fue formulado. El modelo es una extensión de un método teórico de caracterización de superficies denominado Método de distribución de Afinidad Protónica (PDA), el cual se obtiene a partir del análisis de datos de titulación potenciométrica, ZPC del material e isotermas adsorción del tipo Langmuir-Hinshelwood. La naturaleza química del componente en el modelo es establecida por el pH inicial y su peso molecular. Se encontró que para el TiO2-P25 el ZPC es 6.8. El modelo fue validado con datos experimentales de ácido dicloroacético (DCA), 4-clorofenol (4- CP) y fenol (PH) a diferentes condiciones de operación. Se encontró que el modelo tiene alta capacidad predictiva, estabilidad numérica y robustez en la obtención de las soluciones. Para los datos experimentales disponibles, el coeficiente de correlación fue de 0.97.
Palabras Clave: Fotocatálisis, Distribución de Afinidad Protónica, Isotermas de Langmuir-Hinshelwood, Dióxido de titanio, titulación potenciometrica.
1. Introduction
The heterogeneous photocatalysis mechanism involves a proper understanding of the associate phenomenology to molecular processes dynamic on interface semiconductor/solution. In generally the idea is to describe the initiation stage of reactions involving photogenerated electron-hole pairs, the possibility of capture, transport through load bearing species adsorbed on the catalytic surface (water molecules, molecular oxygen and hydroxyl ions) and their recombination; for generating hydroxyl radicals which attack the substrate molecules [Ri ] for redox reactions (Mueses et al, 2013; Monllor et al, 2007; Friedmann et al, 2010; Herrmann, 2010; Turchi & Ollis, 1990; Alfano et al, 1997).
The main mechanism of these processes involves an oxidative way by presence of indirect transfer of photogenerated charges with adsorbed water and air molecules over catalyst surface (Mueses et al, 2013; Fujishima et al, 2010; Turchi & Ollis, 1990; Alfano et al, 1997). In this case, the reactions can occur at the interfacial charge region of the semiconductor solution or at the Helmholtz layer. In the latter case, a direct attack is carried out from the photogenerated hydroxyl radicals to the molecules of the organics compounds adjacent to the surface in pseudo-heterogeneous phase. The molecular adsorption of the species although in much lower proportion involved activity loss by surface contamination of semiconductor.
Most of heterogeneous photocatalysis kinetic models do not consider the molecular adsorption as a rate-limiting stage; different semi-empirical kinetic models describe adequately photocatalytic degradation of organic pollutants have been proposed (Ollis & Turchi, 1990; Sawage et al, 2010; Satuf et al, 2008 and 2007; Zalazar et al, 2005; Ballari et al, 2009; Cabrera et al, 1997; Alfano et al, 1997; Monllor et al, 2007), however these do not consider adsorption mechanism in the mathematical structures. Some researchers have reported that photooxidation reactions follow a classical Langmuir-Hinshelwood mechanism, assuming implicit adsorption/desorption equilibrium, not only in the dark phase but also in the lighted (photoreaction) stage (Friedmann et al, 2010, Herrmann, 2010; Sawage et al, 2010; Alfano et al, 1997; Monllor et al, 2007). However, even if the numerical predictions of experimental data are suitable in most of cases, the effects of the adsorption and the photocatalysis are not clearly distinguished because these overall equilibriumbased models cannot describe the dynamics of the system at high concentrations and high pH. These operating conditions are not desirable since TiO2-based photocatalysis processes are usually favoured at low pH.
Experimental evidence shows that in all heterogeneous photodegradation processes with suspended particles, the initial adsorption stage is critical and can yield substantial organic matter removals (between 5 and 25%), which is finally attributed to the photocatalytic degradation (Mueses et al, 2013; Suaterna et al, 2012).
This phenomenon is critical in a proper quantification of photocatalytic performances, since it is involve in the loss of catalytic ability of the semiconductor surface due to the occupancy by organic molecules of active sites available to photoinduction, in addition, loss of substrate molecules and potential contamination of the catalyst with organic compounds. The molecular adsorption of components (organic molecules, water, oxygen and hydroxyl ions) is essential in limiting the yields of the process; in addition, molecular diffusion in homogeneous phase becomes a limiting of rate reaction (Mueses et al, 2013; Herrmann, 2010; Friedmann et al, 2010; Serpone 1997; Linsebigler et al, 1995).
This indicates that the molecular adsorption phenomenon needs to be quantified independently of the photocatalytic process as prior step reagent system suitability. In this work, we developed a new model based on a predictive determination of potential active sites for adsorption of organic molecules in the semiconductor surface. The model is also based on a conventional method of surface characterization and it was generated from adsorption Langmuir-Hinshelwood isotherms obtained by PDA.
2. Methodology
2.1 Photocatalytic postulates
It was considered that for a heterogeneous photocatalytic process the first step of the reaction mechanism is associated with molecular adsorption phase; therefore, we distinguished the following reaction step (Mueses et al, 2013): i) Molecular adsorption of water, hydroxyl ions and organic compounds over TiO2 surface, ii) Photonic excitation of the semiconductor with a given wavelength, λ, within the absorption catalyst range and the later generation of electrons/ holes (e -/h+) pairs, iii) Recombination of (e ‒/h+) pairs in the particle bulk, iv) Capture of holes by water molecules and hydroxyl ions adsorbed over the TiO2 surface. The probability of holes capture by organic compounds was considered negligible v) Capture of electrons by adsorbed molecular oxygen (main electrons acceptor), and vi) Hydroxyl radical attack to organic molecules in free state contained in the Helmholtz layer.
The n-type semiconductors as the TiO2-P25, which has been used in photocatalysis processes applied for organic compounds removal, are favoured at acid pH (pH <ZPCTiO2). This condition implies the presence of high specific adsorption of dissociated water molecules at the Stern plane in the semiconductor-solution interface (Linsebigler et al, 1995). The process generates a significant availability of the water molecules for subsequent reactions with carriers load, however modifies the surface properties (surface electronic charges) of the semiconductor. This generates a high deficit of protons and of surface active sites for reaction with organic molecules (Linsebigler et al, 1995; Villarreal et al, 2012) but it favors the molecular adsorption of water and hydroxyl ions. Under these circumstances, we can establish mathematically that the concentration of water molecules and hydroxyl ions adsorbed on the surface is kept constant (Mueses et al., 2013; Villarreal et al, 2012).
Despite of the deficit of available active sites, these can be occupied probably by species [Ri] that ultimately affect the initial concentration of the substrate in the heterogeneous photocatalysis processes. An Adsorption Probability Function (APF) is proposed as a theoretical method for predicting the possible amount of organic molecules adsorbed on the semiconductor surface. APF was calculated by using a modification of the PDA method, which permitted a theoretical characterization of the surface properties of solids and to identify the functionality of the acid sites present on the surface of the material from potentiometric experimental data and protonation constants, pK (Villarreal et al, 2012).
The PDA method has been implemented for the surface characterization of semiconductors and activated carbon. The obtained results of the prediction fit satisfactorily to experimental characterization data from the same surface using XDR and BET (Villarreal et al, 2012).
The main assumption was supported under the hypothesis that the PDA method allows to obtain the maximal fraction of active sites on the surface of a material; therefore this method will allow the prediction of the ion absorption [H+] or the fraction of the surface occupied by organic molecules. This fraction could be considered as an equivalent to the highest probability for absorption of species [Ri] on the solid surface.
This is consistent with the molecular mechanism of adsorption in which the adsorbed species for heterogeneous photocatalysis effects are mainly water molecules and hydroxyl ions; therefore, the remaining fraction (<< 1) will be organic species of the reagent system (Mueses et al, 2013).
2.2 Mathematical formulation
The PDA functions were generated by analysis of binding protons curves obtained from potentiometric titration (Villarreal et al, 2012): See Eq. (1).
It is considered that surface has a "multi-site" configuration, in which the presence of acidic sites on the surface is minimal and it can be described by a Langmuir-Hinshelwood equation for the binding protons: See Eq. (2).
Where θi*(pH) and K+ are fraction of site i and protonic affinity constant, respectively; and [H+] is the molar concentration for the equilibrium in the basic form inside the solution with pH = -log[H+] (Villarreal et al, 2012).
The binding protons equation (Ec. 2) can generate a continuous spectrum of acid sites from discrete experimental data coupled to an interpolation mathematical method.
For PDA determination, we considered an integral function in terms of the distribution function f(pK) and the binding protons equation θi *(pH) , which define a global average fraction θAv(pH) of acid sites on surface with an acid affinity constant within an interval of (pH, pH + θpH) : See Eq. (3).
The distribution function f(pK) was calculated by using a local integral solution of the L-H isotherm. This solution was: See Eq. (4).
The summation function was truncated in second term because of the high order terms were considered not representative and they can introduce unstable performance. In addition, these terms are very sensitive to experimental errors. These functions were calculated by using a Taylor series: See Eq. (5) to Eq (6).
Where dh is the pass for the numerical derivative. The integrals were calculated with the Simpson 1/3 algorithm.
2.3 Probability function p(κads)
Finally, since it was required to correct the value of the function of the average amount of active sites for each specific substrate; it was proposed a simple correction factor of Eq. (3), which involved the initial concentration, the molecular weight of the water and the reaction compounds. Furthermore, since the main premise was referred to keep constant molecular adsorption of water and hydroxyl ions, this was divided by the molecular weight of water to have a specific function of the absorption probability. The proposed probability function, p(κads), was given by the following equation: See Eq. (7).
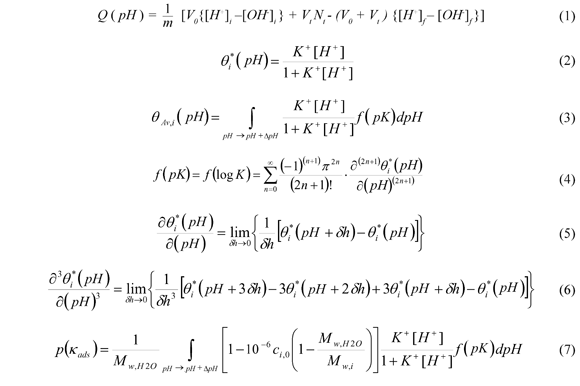
Where Mw,i and Mw,H2O are molecular weight for organic compounds and water respectively. In this equation the initial concentration ci,0 was given in ppm.
2.4 Experimental validation
2.4.1 Materials and equipment
For experimental test we used dichloroacetic acid (DCA: CAS 79-43-6), 4-chlorophenol (4CP: CAS 106-48-9) and phenol (CAS 108-95-2) Merck® analytic grade as organic compounds and titanium dioxide Degussa P-25 as adsorption particle. HCl and NaOH, both analytic grade from Merck®, were used for potentiometric titrations. A dark chamber with 100 mL-beakers, with magnetic stirring, was used for the tests. In addition a spectrophotometer SHIMADZU UV 1800 was used for concentration analysis. Filters of Nylon of 0.15 μm, a pH-meter AB15 from Fisher Scientific, micropipettes EPPNDORF of 100 to 1000 μL and a centrifuge JANETZKI-T23 were used for guaranty an efficient separation of particles from solution.
2.4.2 Experimental procedure for molecular absorption
A solution of 700 mL with distilled water was prepared with the substrate systems (DCA, 4-CP or phenol) using a concentration of 300 ppm for each compound. After dilutions were done to obtain different solutions of 50 mL between 120 and 30 ppm of initial concentration (conventional concentrations found at heterogeneous photocatalytic processes). Each solution was stirred and adjusted to pH 4.0 with NaOH or 2M HCl, depending on the case. An aliquot was taken to associate the blank and later it was weighed and added to the TiO2-P25 to the stirred solution with an equivalent concentration of 0.3 g/L of the catalyst. The solutions were stirred during 12 hours. After stirring, the pH was measured and the aliquot was filtered, centrifuged and analyzed by UV-Vis spectrophotometry. The experiments were performed in the dark chamber in order to avoid the catalyst activation by the natural radiation.
2.4.3 ZPC determination
For the estimation of the ZPC of TiO2-P25, we used the intersection method (Villarreal et al, 2012). An alkaline aqueous solution was prepared with sodium hydroxide and the pH was set at 10.1. The solution was stirred and then the catalyst was added for setting its concentration at 0.01 g/L. Then, it was acidified with HCl for adjusting the pH at 3.1, recording the values of pH at equilibrium. Correspondently, it was prepared an acidic aqueous solution of HCl at a pH of 3.1 and later stirred, meanwhile the TiO2 was added for setting its concentration at 0.01 g/L. This solution was later alkalinized with NaOH at a pH of 10.1.
3. Results and discussion
3.1 Potentiometric titration and ZPC
In Figure 1, we showed the potentiometric titration curve obtained for TiO2-P25 Deggussa. The measured pH in both processes was plotted in function of the sequence of collected data. By transferring the charge effects, the isotherms (acid and basic) presented an intersection that corresponds to the ZPC of the solid.
The obtained value using potentiometric titration for ZPC of TiO2-P25 was 6.8. This value is very similar to the reported one in literature 6.6 (Zalazar et al, 2005). The protonic affinity constant estimated at this pH value was 1.25893x10‒7.
3.2 Adsorption probability function
The probability function of the generalized adsorption for TiO2-P25 semiconductor, in a range between 3<pH<10 and without correction effects for molecular weight and initial concentration, is showed in Figure 2.
From Figure 2, it can be inferred that, at acidic pHs, the probability of adsorption of organic species [Ri] is smaller than at alkaline pHs. This indicates that the adsorption of water molecules and hydroxyl ions is higher and therefore the probability of an attack of photogenerated holes to these species is greater as well. This last phenomenon is beneficial for heterogeneous photocatalytic degradation processes with TiO2-P25 (Mueses et al, 2013; Linsebigler et al, 1995).
The peaks observed in Fig. 2 correspond to maximal values of the adsorption probability for a given pH, which relates the surface potential points interacting with organic molecules supported. The effect of pH on the interface plane semiconductor/solution (Helmholtz phase layer) modifies the band-gap energies (valence and conduction) and it generates positive or negative curvatures to the plane of semiconductor surface energy (Linsebigler et al, 1995). These changes of the surface energy density can support chemisorption or physisorption processes (depending on the chemical nature of the organic molecule), which are reflected in the peaks with most probability in the curve generated.
According to these results, the catalytic activity could benefit those materials with carboxylic acid functional groups or similar ones and reactions with charge transfer (such as photocatalytic reactions). This relationship is valid for values of f(pK) obtained for the distribution curves regarding to the conventional classification reported in literature for the OH groups for different materials (Villarreal et al, 2012).
Figure 2 showed the performance of the probability function for generalized absorption, which does not include molecular weights. In the APF, the chemical nature of the compound is established as a direct dependency on the molecular weight and the intrinsic pH of the molecular surface. We considered effects to the equilibrium only; however, the model does not include the limiting stages due to the mass transport and the molecular diffusion.
In addition, despite of the non-linear performance associated to APF, the estimation of probability curve was stable and robust, which indicated that the approximation used for higher order derivatives was valid to calculate the slopes of the binding protonic isotherms given by the LH model.
3.3 Predictions of the model
From the obtained APF, it is possible to predict the molecular adsorption of characterized organic substances in terms of their molecular properties of superficial energy (reflected in pH) and its molecular weight.
For example, the dichloroacetic acid (Mw =128.942) with a initial concentration of 0.8531 mmol/L (110 ppm approximately), with pH=4.4 and a TiO2-P25 concentration of 0.35 g/L, the value of the uncorrected function (Figure 2) was 0.066756. After applying the correction to the function, its value was 0.47699643. By multiplying this value by the initial concentration and divided by the catalyst load, the final result for the concentration was 1.1626 mmol/gCat. By comparing this result to the experimental value of 1.218 mmol/gCat, an error of 3.2% was estimated. Thereby, it can be stated that the model has high capability of prediction, as shown in Figure 3. It is observed that the model has more accuracy at low initial concentrations but its fitting is not satisfactory at high concentrations. This behavior is explainable because the model was built from the Langmuir-Hinshelwood adsorption isotherms; therefore, it has the same thermodynamic constraints for prediction of experimental data at high concentrations. The overall data for components evaluated (DCA, 4-chlorophenol and phenol) at different operating conditions, have an adjustment coefficient of 0.97, which ensures that the model has high predictive ability.
4. Conclusions
A predictive model for molecular adsorption for organic compounds on titanium dioxide particles Degussa-P25 was proposed. The model was obtained from a method for the theoretical characterization of materials surface called proton distribution affinity method, which is based on an analysis of adsorption/desorption isotherm of Langmuir-Hinshelwood type obtained by potentiometric titration. It was experimentally found that for the P25 TiO2, the ZPC was 6.8, a very similar value to that reported one in literature. The mathematical models showed high predictive ability for molecular adsorption experimental data of DCA, 4-chlorophenol and phenol, with a correlation coefficient of 0.97. The model showed high stability and robust numerical prediction of the experimental data.
5. Acknowledgments
The authors thank the Universities of Valle and Cartagena for funding this publication. Also they thanks to Colciencias for financing their doctoral studies. The authors also thank to the Chemical Engineers, Julian Betancourth and Sergio Castillo, for collaborating wth the experimental runs.
6. References
Alfano, O., Cabrera, M., & Cassano, A. (1997). Photocatalytic reaction involving hydroxyl radical attack I: Reaction kinetic formulation with explicit photon absorption effects. Journal of Catalysis , 172, 370-379. [ Links ]
Ballari, D., Alfano, O., & Cassano, A. (2009). Photocatalytic degradation of dichloroacetic acid: Akinetic study with a mechanistically based reaction model. Industrial & Engineering Chemistry Research , 48, 1847-1858. [ Links ]
Cabrera, M., Negro, A., Alfano, O., Cassano, A. (1997). Photocatalytic reactions involving hydroxyl radical attack II. Kinetics of the decomposition of trichloroethylene using titanium dioxide. Journal of Catalysis , 172, 380-390. [ Links ]
Friedmann, D., Mendive, C. & Bahnemann, D. (2010). TiO2 for water treatment: Parameters affecting the kinetics and mechanism of photocatalysis. Applied Catalysis B: Environmental , 99, 398-406. [ Links ]
Fujishima, A., Zhang, X., & Tryk, D. (2008). TiO2 photocatalysis and related surface phenomena. Surface Science Reports , 63, 515-582. [ Links ]
Herrmann, J. (2010). Fundamentals and misconceptions in photocatalysis. Journal of Photochemistry and Photobiology A: Chemistry , 216, 85-93. [ Links ]
Linsebigler, A., Lu G., & Yates, G. (1995). Photocatalysis on TiO2 surfaces: Principles, mechanisms and select results. Chemical Review , 95, 735-758. [ Links ]
Monllor, D., Gómez, R., Gonzales-Hidalgo, M., & Salvador, P. (2007). The "Direct-Indirect" model: An alternative kinetic approach in heterogeneous photocatalysis based on the degree of interaction of dissolved pollutant species with the semiconductor surface. Catalysis Today , 129 (1-2), 247-255. [ Links ]
Mueses, M., Machuca-Martínez, F., & Li Puma, G. (2013). Effective quantum yield and reaction rate model for evaluation of photocatalytic degradation of water contaminants in heterogeneous pilot-scale solar photoreactors. Chemical Engineering Journal , 215-216, 937-947. [ Links ]
Ollis D., & Turchi, C. (1990). Photocatalytic degradation of organic water contaminants: Mechanisms involving hydroxyl radical attack. Journal of Catalysis , 122, 178-192. [ Links ]
Sagawe, G., Satuf, M., Brandi, R., Muschner, J. Federer, C., Alfano, O., Bahnemann, D., & Cassano, A. (2010). Analysis of photocatalytic reactors employing the photonic efficiency and removal efficiency parameters: degradation of radiation absorbing and nonabsorbing pollutants. Industrial & Engineering Chemistry Research , 49, 6898-6908. [ Links ]
Satuf, M., Brandi, R., Cassano, A., & Alfano, O. (2007). Quantum efficiencies of 4-chlorophenol photocatalytic degradation and mineralization in a well-mixed slurry reactor. Industrial & Engineering Chemistry Research , 46, 43-51. [ Links ]
Satuf, M., Brandi, R., Cassano, A., & Alfano, O. (2008) Photocatalytic degradation of 4-chlorophenol: A kinetic study. Applied Catalysis , B 82, 37-49. [ Links ]
Serpone, N. (1997). Relative photonic efficiencies and quantum yields in heterogeneous photocatalysis. Journal of Photochemistry and Photobiology A: Chemistry , 104, 1-12. [ Links ]
Suaterna, N., Insignares, C., Camargo, A., & Mueses, M. (2012) Solar Photocatalytic Degradation of 4-Chloropenol a Pilot-Scale. Información Tecnológica , 23 (6), 13-24. [ Links ]
Villarreal, T., Mueses, M., & Machuca-Martínez, F. (2012). Protonic Distribution Affinity (PDA) of Aluminium Oxide Synthesized From Wastewater Industry and Used In Methylene-Blue Photodegradation. Journal of Advanced Oxidation Technologies , 15 (2), 399-404. [ Links ]
Zalazar, C., Romero, R., Martin, C., & Cassano, A. (2005). Photocatalytic intrinsic reaction kinetics I: Mineralization of dichloroacetic acid. Chemical Engineering Science , 60, 5240-5254. [ Links ]
Revista Ingeniería y Competitividad por Universidad del Valle se encuentra bajo una licencia Creative Commons Reconocimiento - Debe reconocer adecuadamente la autoría, proporcionar un enlace a la licencia e indicar si se han realizado cambios. Puede hacerlo de cualquier manera razonable, pero no de una manera que sugiera que tiene el apoyo del licenciador o lo recibe por el uso que hace.