Anthracnose, a disease caused by the phytopathogenic fungus Colletotrichum acutatum Simmonds affects different plants, such as tamarillo (Solanum betaceum Cav.), tomato (Solanum lycopersicum L.), strawberry (Fragaria x ananassa Duch.), apple (Malus domestica Borkh.), blackberry (Rubus glaucus Benth.), mango (Mangifera indica L.), among other fruits of economic importance (Damm et al., 2012; Wharton and Diéguez-Uribeondo, 2004). Its presence occurs in temperate and tropical climates around the world. Conventionally, its control has been based on the application of synthetic fungicides, which can cause adverse effects to the environment and to man because of their low selectivity (Gao et al., 2017; Wharton and Diéguez-Uribeondo, 2004; Gaviria-Hernández et al., 2013). In addition, as a result of the gradual development of resistance by the microorganism to this class of substances used for its control, it has become necessary to apply higher and more constant doses in the field, which has led to an increase in the production costs and furthers the problem of food security (Jílková et al., 2015).
In response to the above, new alternatives of control disease in important crops are needed. One of the approaches that have become more prevelant in recent years is the one that uses essential oils and extracts of plants or their major components for the control of phytopathogenic microorganisms (Grande-Tovar et al., 2018; Nazzaro et al., 2017). Alternative methods to control C. acutatum have been developed; these include, the use of natural antimicrobials, biocontrol agents, ultraviolet radiation and resistant cultivars (Falconí and Yánez-Mendizábal, 2018). For example, He et al. (2018) determined the efficacy and possible mechanism of cinnamon essential oil on inhibition of C. acutatum isolated from kiwi fruit. Meanwhile, Rashid et al. (2018) evaluated the antifungal effects of Rhus coriaria L. fruit extracts against tomato anthracnose caused by Colletotrichum acutatum. The results indicate that R. coriaria extract can be utilised as a deterrent and curative natural product for the anthracnose disease in tomatoes. Some substances of this type have demonstrated a high antimicrobial activity, good selectivity, low toxicity, and little persistence in the environment (Tripathi and Dubey, 2004).
Edible and herbal plants/spices such as garlic, oregano, cinnamon, clove, parsley, among other are an important source of essential oils and have been employed alone or combined as natural food preservatives with antimicrobial properties (Pisoschi et al., 2018). Particularly, parsley is an easy plant to grow with low production costs, and is an aromatic herb commonly used in Colombia. The essential oil and different extracts obtained from parsley have shown antimicrobial activity against Gram positive and negative bacteria (Khalil et al., 2018; Semeniuc et al., 2017; Farzaei et al., 2013). To the extent of our knowledge, there are few works to establish the antifungal potential of compounds obtained from parsley. In the present work, the antifungal activity against C. acutatum of n-hexane, dichloromethane, ethyl acetate, and methanol extracts, the essential oil, and their main constituents from parsley was evaluated.
MATERIALS AND METHODS
Chemicals
NaOH, H2SO4, HCl, Na2SO4, glucose, K2HPO4, NaNO3, MgSO4 FeSO4 were purchased from Merck KGaA (Darmstadt, Germany) and Sigma-Aldrich (St. Louis, MO, USA). Solvents methanol (MeOH), dichloromethane (CH2Cl2), ethyl acetate (EtOAc), and n-hexane were acquired from Merck. All chemicals and reagents used were of analytical grade.
General methods
Thin layer chromatography was performed with silica gel F254 (0.2 mm Merck) plates. Compounds were detected by UV fluorescence (254 and 365 nm) and/or spraying with AcOH-H2SO4-H2O (143:28:30, v/v) “Universal stain”, followed by heating at 80 °C for 1-2 min. 1H and 13C NMR spectra were recorded in CDCl3 on a Bruker AMX 300 spectrometer (1H at 300 MHz; 13C 75.5 MHz). Chemical shifts (δ) are reported in ppm relative to the residual solvent signals (CDCl3: δH 7.24 and δC 77 ppm) and coupling constants (J) in Hz. Mass spectrometry analysis was performed using a Hewlett-Packard 6890 (Agilent Technologies) gas chromatograph coupled with a HP 5973 MSD (Mass selective detector-Quadrupole type). A HP-5 MS capillary column (30 m x 0.25 mm x 0.25 μm, Agilent Technologies) was used. Hydrogen was the carrier gas, having a constant flow rate of 1.0 mL min-1 and split ratio 1:10. The temperature was programmed as follows: column temperature, 50 °C; injector temperature, 150 °C; detector temperature, 280 °C. The oven temperature was programmed from 50 to 250 °C at 10 °C min-1 and, then, held isothermally for 6 min. The MS system operated with an electron impact of 70 eV, an acceleration voltage of 1.1 kV, and scan range of m/z 40-500. The products were identified by comparison of their mass spectra with those of the US National Institute of Standards and Technology (NIST) mass spectral library (Version 2008). Quantitative analysis of each essential oil component (expressed in percentages) was carried out by peak area normalization measurement.
Plant material and extraction procedure
Extracts: Fresh parsley plant materials (curly leaf) were purchased from a local supermarket in Medellín, Colombia. Samples were washed under tap water and roots were discarded. Leaves and stems were homogenized in a laboratory blender. Then, vegetal material (900 g) was extracted sequentially with 700 mL of each solvent (n-hexane (HEX), dichloromethane (DCM), ethyl acetate (EA), and methanol (ME)) by percolation (5 cycles per 24 h) a room temperature until exhaustion. Extracts were dried over anhydrous sodium sulfate, filtered, evaporated using a rotary evaporator (Buchi Heating Bath B-490, Buchi Rotavapor R-200) under reduced pressure at 40 °C, and stored in a refrigerator at 4 °C in amber vials. Essential oil: The essential oil (EO) was obtained by hydrodistillation. A quantity of 900 g of fresh leaves and stems of parsley was coarsely chopped and immersed in 1.5 L of distilled water contained in a 3 L flask. Distillation was carried out using a modified Clevenger apparatus. The extraction process was performed for 2 h after the first drop of distillate until complete exhaustion of the plant. Then, sodium chloride (1 g) and 40 mL of dichloromethane (2x20 mL) was added with the aqueous distillate in a separating funnel. The organic layer was separated, dried over sodium sulfate, filtered, evaporated using a rotary evaporator (Buchi Heating Bath B-490, Buchi Rotavapor R-200) under reduced pressure at 40 °C, and stored in a refrigerator at 4 °C in amber vials.
Isolation and identification of major constituents in bioactive materials
The bioactive n-hexane extract and essential oil from P. crispum were measured chromatographically with silica gel (Merck, Darmstadt, Germany, silica gel 60, 230-400 mesh) column and eluted with gradients of n-hexane:EtOAc (100:0, 95:5, 90:10, 80:20, 70:30, 0:100 v/v). Next, the n-hexane and n-hexane:EtOAc (95:5) fractions were measured in a silica gel column chromatograph eluted with n-hexane and gradients n-hexane:CH2Cl2 (98:2, 95:5, and 90:10 v/v). Fractions 2 to 4 were mixed and measured through a Sephadex LH-20 column chromatograph eluted with n-hexane:CH2Cl2:MeOH, 50:25:25, v/v affording two compounds, which were identified through spectroscopic methods (one-dimensional (1H and 13C) and two-dimensional (COSY, HSQC and HMBC) NMR, as well as EI/MS analysis.
Antifungal activity
The strain of phytopathogenic fungus C. acutatum was isolated from commercial fruits of tamarillo (S. betaceum) affected by the disease and purchased at the local markets in the city of Medellín (Colombia). Identification was performed by the Phytopathology Laboratory of the Universidad Nacional de Colombia-Sede Medellín, evaluating morphological and molecular characteristics (Afanador-Kafuri et al., 2014). The evaluation of antifungal activity was carried out using the poisoned agar method (Balouiri et al., 2016). Essential oil, extracts, and pure compounds were mixed with ethanol (<0.2% EtOH) and tested at 100, 200, and 400 μg mL-1. Thymol (2-isopropyl-5-methylphenol) and carbendazim (methyl benzimidazol-2-ylcarbamate) at 100 μg mL-1 were used as positive controls. Mycelial growth (mm) were determined as mean values ± standard deviation of three replicates of mycelium diameter. In addition, for bioactive materials and their major constituents, the concentration that inhibits 50% fungal mycelia growth (IC50) was determined according to Rivillas and Soriano (2007).
RESULTS AND DISCUSSION
Table 1 shows the extraction yield for the four solvents of different polarities -n-hexane (HEX), dichloromethane (DCM), ethyl acetate (EA), and methanol (ME)-, and the essential oil (EO) collected by hydrodistillation from P. crispum. The order of increasing yield was HEX < DCM < EA < ME, coinciding with a growing polarity of the extracting solvent. Thus, the highest yield was achieved by the most polar solvent, methanol. The n-hexane extract presented approximately 10 times less of the weight reached by the methanol extract. This suggests that the proportion of low polarity products extractable with hexane in P. crispum is low, compared to those of high polarity present in extracts in ethyl acetate and methanol. The yield from essential oil hydrodestillation extraction was closer to the extraction yield from n-hexane. Overall, the total amount of extractable material with organic solvent in P. crispum was 13.73 g (1.42%).
Then, all extracts and essential oil were tested for antifungal activity using the poisoned-food technique at 100, 200, and 400 μg mL-1, according to Balouiri et al. (2016).
The inhibitory effects of organic extracts and essential oil of P. crispum at 400 μg mL-1 on the growth of C. acutatum are presented in Figure 1. All extracts displayed a significant inhibitory effect in the growth of C. acutatum after 264 h. In general, the growth of fungal mycelium was dependent of the type and amount of extract in the culture medium. The n-hexane extract caused the least growth of C. acutatum; after 264 hours, the radial growth was almost 30 mm less than the control. Higher polarity extracts, methanol followed by ethyl acetate, had the lowest inhibitory effect on C. acutatum. After 72 h, the n-hexane extract showed an inhibition percentage of 57%. Then, the fungistatic effect presented a slight decrease, reaching 48% inhibition after 264 h.
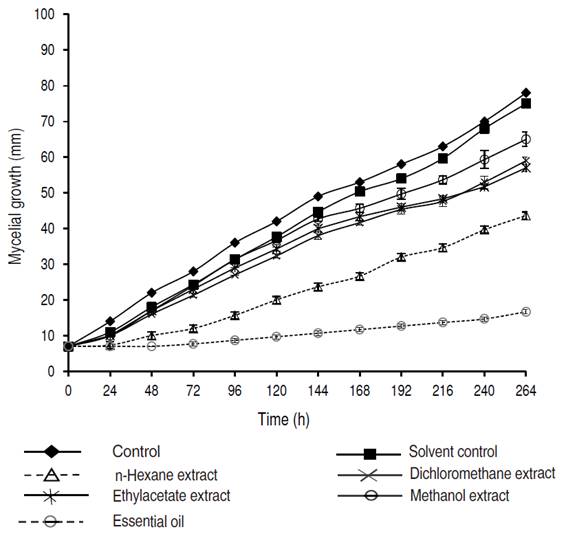
Figure 1 Effect of extracts and essential oil of Petroselinum crispum at 400 μg mL-1 on radial mycelial growth of Colletotrichum acutatum.
The essential oil of P. crispum displayed the highest inhibitory effect. At 400 μg mL-1, essential oil ofP. crispum inhibited the radial growth of C. acutatum completely during the first 72 h. Similarly, the inhibition percentage was slightly decreased at 78% after 264 h. Even at 100 μg mL-1, essential oil exhibited inhibition percentages of C. acutatum of almost 60% during the 264 h of the analysis (data not shown). Thymol and carbendazim® presented a significantly higher antifungal effect (inhibition percentages of 100%) as compared to all evaluated extracts. Carbendazim is commonly used for control of anthracnose disease, both in the field and postharvest. However, some plant pathogens, such as C. acutatum and C. gloeosporioides, have strains resistant to carbendazim (Zhang and Huang, 2007). Meanwhile, thymol has been recognized for its potent antifungal activity (Numpaque et al., 2011). The IC50 values calculated for the n-hexane extract and the essential oil of P. crispum were 316.7 and 185.1 μg mL-1, respectively. The high antifungal activity of essential oil of P. crispum found in the present study is in agreement with that reported by Linde et al. (2016). The chemical composition of essential oil and n-hexane extract from leaves and stems of P. crispum was analyzed by means of GC-MS. The detected constituents (relative amounts higher than 0.1%) are shown in Tables 2 and 3. Analysis by GC-MS led to the identification and quantification of about 23 components. Among them were 12 in the essential oil and 22 in the n-hexane extract, comprising between 93.5-99.1% of the total composition of the essential oil/n-hexane extract. Phenylpropenes were the main components detected in the essential oil (77.0%) and n-hexane extracts (48.7%) for P. crispum. Parsley- or dill-apiole (27.6 and 43.2% in n-hexane extract and essential oil, respectively) and myristicin or sarisan (18.7 and 30.8% in n-hexane extract and essential oil, respectively) were the major constituents on P. crispum. The phenylpropene safrole was also detected in low proportion.
Table 2 Relative abundance (%RA) of major compounds obtained by GC-MS (%) from n-hexane extract of P. crispum.
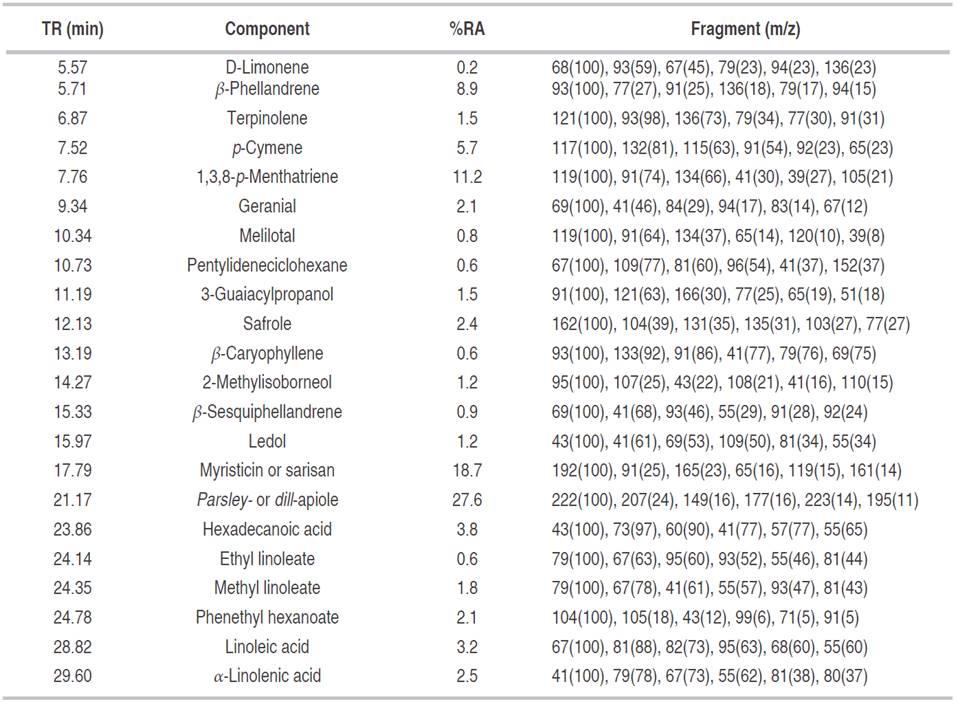
Table 3 Relative abundance of major compounds obtained by GC-MS (%) from essential oil of P. crispum.
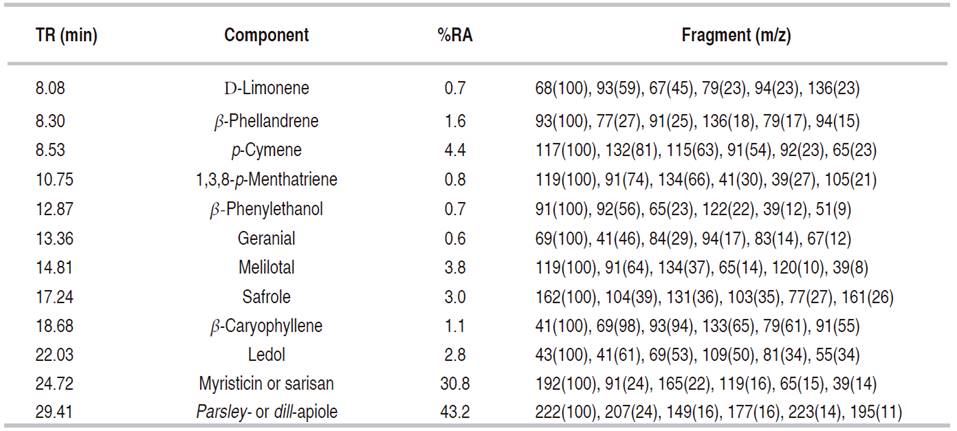
Monoterpenes (27.5%), fatty acids (11.9%), oxygenated monoterpenes (3.3%), sesquiterpenes (2.7%), and others (5.0%) were also found in the extract. Meanwhile, 7.5% monoterpenes, 0.6% oxygenated monoterpenes, 3.9% sesquiterpenes, and 4.5% others were detected in the essential oil.
Previous reports differ on the major constituents in essential oil of curley leaf parsley. Some authors have found that monoterpenes are the dominant compounds. Thus, El-Zaeddi et al. (2016b) reported that 1,3,8-p-menthatriene (38.4-48.8%) and β-phellandrene (22.2-29.5%) are the major constituents in the essential oil of parsley grown in Spain, obtained by hydrodistillation using a Deryng system (the Polish version of the Clevenger apparatus). Kurowska and Galazka (2006) found α-pineno (32.0%) to be the major constituent of the essential oil of P. crispum cultivated in Poland, also having β-pineno (19.0%), myristicin (18.3%), and apiole (10.1%). On the other hand, Camilotti et al. (2015) reported that phenylpropanoides were the principal compounds, comprising 52.07% of the oil and consisting mainly of apiole (41.05%) and myristicin (5.08%). According to Zhang et al. (2006), myristicin (32.75%) and apiole (17.54%) were the major constituent in essential oil of parsley cultivated in China. Stankovic et al. (2004) found that apiole (57.0%) was the major constituent of essential oil of P. crispum cultivated in Serbia and Montenegro. Linde et al. (2016) reported apiole (50.3%) and myristicin (14.0%) as major constituents in the essential oil of parsley cultivated in Brazil. The chemical composition of the parsley is also dependent on the variety analyzed; in the flat-leaf parsley (Petroselinum sativum) contents of myristicin and apiol have been found that vary between 25-47% and 18-23%, respectively (Romeilah et al., 2010; El-Zaeddi et al., 2016a). In this way, apiole and/or myristicin have always been among the major constituents of essential oils obtained from parsley. This diverse chemical composition could be a result of several parameters including geographical origin, vegetative stage of plant, parsley cultivar, storage condition, and extraction method, as well as their interactions with microorganisms and insects (Chatzopoulou and Katsiotis, 1995). In addition, abiotic factors like luminosity, temperature, rainfall, nutrition, time of the day for harvest, and post-harvest techniques are also important to the chemical composition of essential oil (Morais and Castanha, 2012). The results by Camilotti et al. (2015), Zhang et al. (2006), Linde et al. (2016) and Stankovic et al. (2004) agree quite well with the results of this study.
Then, the n-hexane extract and essential oil from P. crispum were submitted to chromatography on silica gel, using n-hexane and n-hexane:EtOAc (95:5). Further sequential purification by column chromatography using silica gel (eluted with n-hexane and n-hexane:CH2Cl2, 98:2) and Sephadex LH-20 (eluted with n-hexane:CH2Cl2:MeOH, 50:25:25) , which were identified through one-dimensional (1H and 13C) and two-dimensional (COSY, HSQC, and HMBC) NMR spectroscopic methods, in addition to EI/MS analysis.
Parsley-apiole: The compound was isolated as a yellowish oil; C12H14O4. EI-MS m/z: 222(100)[M]+, 207 (26), 195 (11), 191 (11), 177 (18), 149 (16). 1H NMR (300 MHz, CDCl3): δ 6.32 (1H, s, H-2), 5.95 (2H, s, -OCH2O-), 5.90-6.00 (1H, m, H-8), 5.08 (1H, dd, J = 1.5, 12.9, H-9b), 5.03 (1H, dd, J = 1.5, 9.6, H-9a), 3.89 (3H, s, -OCH3), 3.86 (3H, s, -OCH3), 3.31 (2H, dd, J = 1.5, 6.3, H-7). 13C NMR (75 MHz, CDCl3) δ: 138.9 (C-3), 138.6 (C-5), 137.2 (C-8), 136.1 (C-2), 135.0 (C-4), 125.6 (C-9), 115.1 (C-1), 108.1 (C-6), 101.3 (-OCH2O-), 59.9 (-OCH3), 56.7 (-OCH3), 33.9 (C-7).
Myristicin: The compound was isolated as a colorless oil; C11H12O3. EI-MS m/z: 192(100)[M]+, 165 (22), 161 (13), 119 (16), 91 (24). 1H NMR (300 MHz, CDCl3): δ 6.29 (1H, d, J = 1.4, H-2), 6.26 (1H, d, J = 1.4, H-6), 5.83 (2H, s, -OCH2O-), 5.77 (1H, m, H-8), 5.03 (1H, dd, J = 1.5, 17.0, H-9a), 4.92 (1H, dd, J = 1.5, 8.1, H-9b), 3.79 (3H, s, -OCH3), 3.21 (2H, d, J = 6.3, H-7). 13C NMR (75 MHz, CDCl3) δ: 144.9 (C-4), 143.6 (C-5), 137.4 (C-3), 136.7 (C-8), 125.6 (C-1), 115.9 (C-9), 107.6 (C-2), 102.7 (C-6), 101.3 (-OCH2O-), 50.9 (-OCH3), 33.3 (C-7). Spectral analyses were in agreement with those reported in the literature (Di Stefano et al., 2011; Razzaghi-Abyaneh et al., 2007). Chemical structures are presented in Figure 2.
Antifungal activity of isolated compounds was evaluated for C. acutatum. Results are presented in Figures 3 and 4. The myristicin (a phenylpropanoid with one methylenedioxy and one methoxy group) presented a moderate fungistatic activity for all the evaluated concentrations, as compared to thymol and the carbamate carbendazim®. However, it was observed that even at the concentration of 100 μg mL-1, myristicin presented a significant reduction of mycelial growth of the microorganism, as compared to the control and solvent control. In general, the fungistatic activity of myristicin was concentration dependent. At 200 μg mL-1, the inhibition percentage was greater than 35%, even after 264 h. At this time, the concentration of 400 μg mL-1 showed a 55% inhibition.
The moderate antifungal property of myristicin has been previously reported (Moreira-Valente et al., 2015; Meepagala et al., 2005). According to Moreira-Valente et al. (2015), myristicin at 0.3% inhibited Aspergillus flavus and A. ochraceus 85 and 80%, respectively.
Parsley-Apiole (a phenylpropanoid with one methylenedioxy and two methoxy groups) had a high fungistatic activity against the phytopathogenic fungus C. acutatum. The development of fungal colonies was initiated only after 24 hours of inoculation at a concentration of 75 μg mL-1 and after 48 hours for other concentrations evaluated. Growth inhibition percentages were found to be between 100 and 65% after 96 h at concentrations greater than 100 μg mL-1. The IC50 calculated for parsley-apiole was 40.0 μg mL-1.
In an earlier work on the antifungal properties of parsley-apiole, it was shown to have a varying degree of growth inhibitory effects against B. cinerea, C. acutatum, C. fragariae, C. gloeosporioides, and F. oxysporium (Meepagala et al., 2005). The authors showed that parsley-apiole exhibited high antifungal activity against B. cinerea but low activity against C. acutatum, C. fragariae, C. gloeosporioides, and F. oxysporium in the microdilution assay (Meepagala et al., 2005). This apparent discrepancy with respect to the present results could be attributed to the fact that different strains of C. acutatum and different methods were used to evaluate antifungal activity. In addition, parsley-apiole and myristicin have been reported as inhibitors of aflatoxin G1 production in Aspergillus parasiticus (Razzaghi-Abyaneh et al., 2007).
Both oxygenated phenylpropenes have an allyl side chain bonded to the aromatic ring which confers a lipophilic characteristic. Although not much is known about the mechanism of antifungal action of phenylpropenes, it has been reported that their lipophilic characteristic enables them to enter between the fatty-acid-chain constituents of the membrane lipid bilayers and alter the fluidity and permeability of the cell membranes (de Almeida et al., 2009). Remarkably, although the structural difference between parsley-apiole and myristicin is only seen in the presence of one methoxyl group on the aromatic ring, parsley-apiole was significantly more active than myristicin against C. acutatum. These results may suggest that electronic and/or steric factors in phenylpropenes might be important for antifungal activity. Further research to obtain information on the structure-activity relationship is required.
CONCLUSIONS
In the present study, the mycelial growth inhibition of Colletotrichum acutatum using essential oil obtained by hydrodistillation and four successive extracts (n-hexane, dichloromethane, ethyl acetate, and methanol) from fresh leaves and stems of parsley (P. crispum curly leaf) was studied. Essential oil and n-hexane extract exhibited the strongest antifungal activity against C. acutatum. GC/MS analysis demonstrated that the dominant components of both bioactive materials were phenylpropenes, specifically parley-apiole (or some isomer) and myristicin (or some isomer). Both constituents were isolated, and their structures unambiguously confirmed by spectroscopic methods. Then, antifungal activity against C. acutatum of these phenylpropenoids was evaluated. Parsley-apiole exhibited a strong fungistatic activity. Accordingly, parsley-apiole could be suggested as a structural template for developing new antifungal agents.