I. Introduction
Red clays are widely distributed in southwest and south central regions of China (Editorial of Engineering Geology Manual, 2007); accordingly, red clay is frequently utilized as a subgrade filling for fiscal and environmental reasons. Thus, studies of the mechanical properties of this subgrade encompass a high practical significance within traffic engineering. Several previous studies have undertaken in-situ mechanical testing on red clay foundations, with results indicating significant variation with respect to both the bearing capacity and deformation modulus of red clay bases in concurrence with varying mechanical properties, water content, particle composition, occurrence conditions, soil structure and interior fracture, among other factors (Long, Chen, Xiao, & Peng, 2009; Kong, Luo, & Yuan, 1995; Kong & Luo, 1993; Bai, Kong, Guo & Wan, 2010; Bai and Wan, 2010; Peng, Lin, Wang, & Wu, 2015). Moreover, studies have shown that the aforementioned red clay characteristics may exert considerable effects on both the safety and suitability of the subgrade when used as a subgrade filling (Editorial of Engineering Geology Manual, 2007; Kang et al., 2010).
The remolding procedure is typically employed to overcome the inherently weak engineering characteristics of red clays. Numerous previous studies have examined the static properties of remolded red clays. Results indicate that the remolding procedure can effectively eliminate adverse design factors including cracking and uneven distribution, thus enhancing friction, bite force and bearing capacity (Yang & Lu, 2011; Liang & Mo, 2010; Zhang, Jiang, & Yang, 2011; Kaynia Madshus & Zackrisson, 2000). However, within traffic engineering, the dynamic properties of subgrade materials are critical; to date, a paucity of data exist on the dynamic characteristics of remolded red clay, thus representing a significant knowledge gap in light of its frequent use as a subgrade material. The dynamic properties of geomaterials are directly related to dynamic loading which typically encompasses three elements, namely, waveform, amplitude, and frequency. Previous investigations have shown that the dynamic loading borne by the subgrade is analogous to a sine wave pattern. Thus, the sine wave has frequently been employed to simulate traffic loading within traffic engineering applications (Kaynia et al., 2000; Liu, 2010). The amplitude of dynamic loading exerts significant influence on the inherent dynamic properties of geomaterials, particularly those of saturated soils. An improvement in the magnitude of dynamic loading may result in significantly decreased dynamic subgrade strength, in concurrence with the accelerated development of accumulated plastic strain (Li, 2013; Chen, 2015). The influence of vibration frequency on the dynamic properties of geomaterials may be approximately divided into three types. Firstly, the dynamic strength of the geomaterial may be improved, in concurrence with a retardation of the accumulated plastic strain due to an increased vibration frequency (Duncan and Chang, 1970; Lin, 2011). Secondly, the effect of vibration frequency on the dynamic properties of a geomaterial may not be visible (Li, 2010; Zhou, 1998); lastly, the dynamic strength and ability to resist deformation may be reduced due to an increase in associated vibration frequency (Zhang, 2008). Thus, an investigation of the dynamic characteristics of geomaterials under cyclic loading is of critical importance. In the current study, several tests have been designed and undertaken to examine the dynamic properties of remolded red clays.
Furthermore, previous investigations have shown that loading history exerts significant effects on the dynamic characteristics of geomaterials. An increased consolidation confining pressure has been demonstrated to improve the ability of geomaterials to resist external dynamic loading, in concurrence with reduced dynamic strain (Lin et al., 2013; Gu, Wang, Cai, Yang & Gao, 2012; Zhou and Gong, 2001). Moreover, consolidation ratio also exerts a marked influence upon dynamic soil properties, albeit, with different types and magnitudes of influence associated with different geomaterials. For example, upon increasing the consolidation ratio within a sand or categorically coarse-grained soil, dynamic strength is observed to exhibit an initial increase, followed by a subsequent decrease. However, the associated turning points of various soils have been shown to vary (Zhang, 1991; He, 1998; Zhang, He, Fei & Gao, 2006; Lunne, Berre, Andersen, Strandvik, & Sjursen, 2006; Li, Chen & Jiang, 2015). Conversely, an increase of the consolidation ratio of saturated soils reduces the associated dynamic strength (Lunne et al., 2006); a significant consolidation ratio has been shown to promote the formation of a shear band due to the inherently low strength of saturated clays. Similarly, the effect of compactness upon the dynamic strength of geomaterials is clearly evident; upon compaction, bulk density is increased, thus increasing dynamic strength and retarding the development of accumulated plastic strain (Li, 2013; Li, Chen, & Jiang, 2013).
In the current study, a series of cyclic dynamic triaxial tests have been undertaken under varying load histories and dynamic loadings to investigate the dynamic properties of remolded red clay and the associated influencing factors. Accumulated plastic strain and dynamic strength of samples have been evaluated and compared, leading to the development of predictive mathematical models about both dynamic characteristics. Accordingly, the critical dynamic strength of remolded red clay is effectively examined, with empirical results presented for use in future engineering applications, thus representing significant utilities from both an academic and applied perspective.
II. Dynamic triaxial tests of remolded red clay samples
1. Dynamic Triaxial Apparatus
The employed dynamic triaxial test used a computer-controlled electro-hydraulic servo dynamic triaxial test system (SDT-10), as shown in Figure 1. Summarily, the system comprises a microcomputer-controlled electro-hydraulic SDT test system host, a standard triaxial pressure cell, a set of electro-hydraulic servo exciters for effective application of confining pressure, a set of digital multi-channel electro-hydraulic servo-controlled systems and a hydraulic source. The test system is capable of providing a maximal axial dynamic load of 10 kN, while the triaxial pressure cell is associated with a maximum confining pressure of 1 MPa. Deformation sensor precision is > 0.5%; the precision of measured strain is approximately 10-4. The provided axial and exciting lateral frequency is 0-5 Hz, while control type may be stress-controlled.
2. Test Sample Parameters
The remolded soil sample used was obtained from an area adjacent to the interurban railway connecting Wuhan and Xianning. Subsequently, the engineering properties and test parameters of the sample were confirmed with several laboratory analyses including grain size, liquid limit, plastic limit and compaction. Grain size analyses indicate that grain sizes associated with the test samples were all < 0.075 and thus categorized as being fine grained; measured soil proportion was 2.7. Determined liquid limit, plastic limit, and plastic index were 45%, 20.9% and 23.1%, respectively (Table 1). Accordingly, the study sample was shown to exhibit a relatively high plastic index, id est fine grain size in concurrence with high clay content. According to the 'Railway Engineering Geotechnical Test Regulations of China,' the study sample is categorized as cohesive clay with a high liquid limit. Compaction test results indicate an optimum moisture content of 20% and a maximum dry density of 1.616 g/cm3 (Fig. 2.).
3. Test Program
Applied dynamic load amplitude must remain static to comprehensively analyze the developmental accumulated plastic strain and dynamic strength of remolded red clay under varying long term cyclic loads. Firstly, varying load histories were applied to the study sample, after which different cyclic loads were applied; the dynamic mechanical properties of the test sample in the presence of varying load histories were continuously monitored; all undertaken analyses were stress-controlled. Four influencing factors were considered during test procedures, namely compactness, confining pressure, consolidation ratio and vibration frequency. Specific test programs are presented in Table 2.
4. Soil sample preparation and loading
All analyses were undertaken by the 'Geotechnical Test Regulations of China' to ensure test data reliability. Chiefly:
(1). All soil samples were prepared using the same method.
Before dynamic loading, varying confining pressures and consolidation partial pressures were applied to soil samples until deformation conditions were reached (axial deformation <0.005 mm in 5 minutes).
During the test procedure, varying cyclic loads were applied to soil samples in concurrence with continuous monitoring of dynamic σ- ε curves (Fig. 3.).
Test termination occurred when vibration time reached 2000 times or accumulated plastic strain reached 15%.
III. Test results and analysis
1. Test Curves of Long-term Cyclic Loading Tests
1.1. Accumulated plastic strain test curves
Several accumulated plastic strain curves under different load histories were obtained (Figs. 4 -7). As shown, under relatively small dynamic loads, the accumulated plastic strain of the test samples developed rapidly during initial loading, followed by gradual stabilization due to soil compaction. Under relatively high dynamic loading, accumulated plastic strain grows rapidly, exhibiting a more constant gradient during initial loading, with an elevated rate of development during later loading stages. Upon exertion of larger dynamic loading values, developing standards during all loading stages were observed as being relatively similar, with soil deformation occurring fairly rapidly.
1.2. Dynamic strength test curves
Dynamic strength of soils varies with loading rate and the cyclic impacting effects of dynamic stress. Typically, dynamic stress is associated with a particular deformation criterion in concurrence with the impacting vibration times of dynamic loading (Xie, 1986). In the current study, the definition of dynamic strength resulted in marked variation in parallel with diverse conditions. Furthermore, dynamic strength is closely related to the cyclic vibration time and the deformation criteria. Accordingly, accurate determination of deformation strain is necessary to an increased understanding of soil-related dynamic stress. The selected approach for determination of dynamic strength was when the accumulated plastic strain reached 5%. Dynamic strength variation on remolded red clay samples under diverse conditions are presented in Figures 8-11. As shown, under variable test conditions, dynamic strength (σd) was observed to decrease non-parametrically with vibration time. Under the effects of relatively large dynamic stress, soil deformation occurred rapidly in concurrence with reduced dynamic loading, gradually increasing deformation vibration
time and a stabilizing relational curve. Effectively, these conditions indicate that study samples exhibited a critical dynamic strength σcr. Thus, soil deformation does not occur when the measured dynamic stress was <σcr.
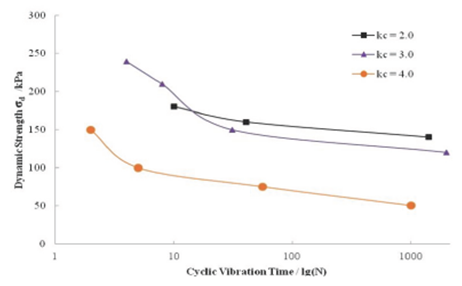
Figure 10 Dynamic strength results of remolded red clay samples (influenced by consolidation ratio).
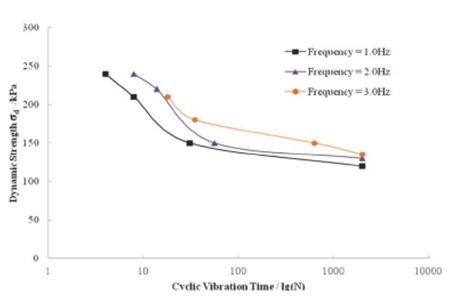
Figure 11 Dynamic strength results of remolded red clay samples (influenced by vibration frequency).
Additionally, results suggest soil dynamic resistance is improved upon enhancement of soil compactness, soil confining pressure and vibration frequency of dynamic stress. The association between measured consolidation ratio and dynamic strength was dissimilar to other influencing factors. Varying factors were shown to exert different effects on the dynamic strength of study samples. For example, measured soil compactness had a highly significant effect on the measured dynamic strength of study samples, while other potentially influencing factors (e.g. confining pressure and vibration frequency) were shown to produce more subdued effects. Overall, varying load histories demonstrated a marked impact on the dynamic strength of remolded red clays.
2. Critical Dynamic Strength and Equation Development
Dynamic strength is a first soil parameter regarding dynamic loading to reflect and relate the development of soil deformation in concurrence with fluctuating strength. Moreover, as previously outlined, a decreased dynamic stress is shown to be associated with and increased vibration time. Consequently, below a specified level of dynamic stress, soil deformation does not occur, with this specified level of stress defined as the critical strength (σcr) (Heath, Waters, Shenton & Sparrow, 1972), as previously demonstrated (Liu, 2010; Li, 2010; Zhou, 1998). Based upon results and figures about dynamic stress obtained within the current study, characteristic σcr for test samples are presented in Table 3.
While cyclic triaxial testing is the most frequently employed method for the determination of critical dynamic stress within a specified soil type or sample, its use on-site is problematic due to the necessarily high number of tests required for accurate approximation, the relatively high level of proof complexity and the potential presence of variable influencing factors. Accordingly, in terms of practical application, a generalized empirical equation comprising compactness, confining pressure, consolidation ratio and vibration frequency has been developed via fitting analysis of calculated critical dynamic strengths. The suitable effect of the developed empirical equation was appropriately high, with an RSQ of 0.98. Due to influencing factors on the critical dynamic strength of test samples, in concurrence with inherent limitations associated with test methods employed, it is recommended that use of the presented equation be confined to conditions analogous to those maintained in its development. The developed empirical equation is as follows:
3. Influencing Factor Analyses of Accumulated Plastic Strain and Critical Dynamic Strength
3.1. Accumulated plastic strain analysis
εp is shown to be influenced to varying degrees by dynamic stress, compactness, confining pressure, consolidation ratio and vibration frequency based upon developmental curves of accumulated plastic strain (Figs. 4-7); these variations are primarily due to interior soil conditions being differentially affected by dynamic stress and varying load histories.
Factors accelerating ep development:
Increased dynamic stress may accelerate intra-particle friction and promote the formation of sliding surfaces, thus accelerating εp development.
εp may be accelerated via enhancement of the consolidation ratio due to increasing partial stress, in concurrence with an increased consolidation ratio and relatively large partial stress thus causing soil structure deformation, an inherently decreased dynamic strength and an acceleration of the development of εp.
(ii) Factors delaying ep development
Soil εp development may be retarded via increased soil compactness and confining pressure; the effect of varying soil compactness on observed εp is particularly significant. Upon improvement of soil compactness and confining pressure, the void ratio is decreased, and friction between soil particles is increased, thus enhancing to soils ability to resist external dynamic loading.
In the case of stiff remolded red clays, the development pattern of εp may also be effectively retarded, due to the combination of compaction over a short time and a significant vibration frequency. Conversely, friction between soil particles will be increased in the presence of a high vibration frequency, resulting in increased resistance to external dynamic loading.
(ii) Influencing factor analysis of σcr.
As shown (Figs. 8-11; Table 3.), σcr is influenced to varying degrees by diverse loading histories; parametric associations exist between measured compactness, confining pressure, consolidation ratio, vibration frequency and critical dynamic strength of the remolded red clay samples tested in the current study. Accordingly, an improvement in soil compactness, confining pressure and vibration frequency will result in an increased critical dynamic strength increase. Conversely, an increased consolidation ratio, results in a decreased dynamic strength. It happens primarily due to the inherently increased soil density and a higher level of soil particle contact associated with increased soil compactness, thus leading to increased critical dynamic stress. Increased confining pressure, also associated with higher levels of soil compaction, resulted in a lower level of dynamic strength increase. It is theorized that upon increasing vibration frequency, the contact area between soil particles is significantly increased in concurrence with increased intraparticle frictional forces, thus improving the soils' ability to resist external loading.
When consolidation ratio was increased, measured critical dynamic strength was decreased; the exertion of high large partial stress results in localized soil deformation, leading to a reduced level of soil dynamic strength. Several large consolidation ratios were used to study the effect of the consolidation ratio on critical dynamic strength; an inversely proportional relation was found to exist between consolidation ratio and critical dynamic strength. In the case of consolidation ratios < 2.0, the authors recommend further analyses.
IV. Conclusions
Several dynamic triaxial tests were carried out on remolded red clays under varying load histories, thus permitting examination of the effects of dynamic stress and different load histories on the dynamic properties of these subgrade materials. Based on test results, the following conclusions have been developed:
(1) Load records were shown to directly influence the dynamic properties of remolded red clays, with diverse load histories exhibiting equally different levels of influence. Increased levels of compactness, confining pressure and vibration frequency, were shown to increase the test samples ability to resist external loading, thus retarding accumulated plastic strain development and improving the critical dynamic strength of the soil. Due to inherent soil properties, the effects of compactness and confining pressure are especially significant. Conversely, accumulated plastic strain development was accelerated in concurrence with decreased critical dynamic strength via improvement of the consolidation ratio, primarily due to the development of localized deformation and increased partial stress.
(2) An increased vibrational frequency was shown to result in significantly improved dynamic strength and an increased ability to resist external loading within test samples. Within categorically stiff unsaturated clays, the effects of increased vibrational frequency pore water pressure in soil are not significant. Therefore, soil dynamic strength and modulus remain under the influence of friction between soil particles.
Based on analyses of the effects of varying load histories on the dynamic characteristics of remolded red clay, an empirical equation for relating critical dynamic strength to these load records has been developed and presented; this may be utilized for future engineering applications.