INTRODUCTION
The northeast of Brazil is the largest domestic producer of shrimp, with 88.6% of the total farms and 99.4% of the production in the country. This venture represented an alternative for generating income for small producers and unskilled labor, especially for employees that survived extractivism. Local production is concentrated in the States of Ceará and Rio Grande do Norte, with 71.7% of the total production in 2018 (Vidal and Ximenes, 2016; IBGE, 2019).
Currently, the fishing industry is concerned with appropriate waste handling, avoiding harm to the environment (Moura et al., 2006; Gonçalves, 2011). Part of this waste is crushed and transformed into flour for the enrichment of animal feed, often used in shrimp farming tanks (Carranco et al., 2003). However, the final destination of the vast majority is soils or river effluents, with large environmental impacts (Bessa-Júnior and Gonçalves, 2013).
Processing residues and leftovers from beneficiation (removal of non-edible parts such as cephalothorax, abdominal segments, and tails, in addition to portions of retained meat) represent about 50% of the total weight (Decker et al., 2016), a high percentage for disposal. In addition, Brazil's production grew from 90 to 120 thousand tons of shrimp between 2019 and 2020 (estimate), respectively (Rocha, 2020). Thus, environmental legislation is needed for the treatment of solid waste from the shrimp processing industry, which leads to extra production costs (Vieira et al., 2011). The transformation of these residues into co-products with added value is vital to minimizing problems in production and achieving greater efficiency in processing (Beerli et al., 2004). Shrimp processing residues have been used for animal feed production, chitin extraction, carotenoid pigments, remediation of contaminated water, plant disease control, water stress and environmental impacts (Vieira et al., 2011; De Souza et al., 2015; Seabra et al., 2014; Núñez-Gómez et al., 2016; Ghini et al., 2006; Bezerra et al., 2017; Decker et al., 2016; Sumardiono and Siqhny, 2019).
There are several alternatives for using fishing residues, e.g. as fertilizers in agriculture, which provide nutrients such as nitrogen and phosphorus for microorganisms and plants, increasing productivity and improving physical conditions and soil chemicals (Decker et al., 2016). This alternative allows production systems that do not use chemical synthesis fertilizers to obtain organic fertilizers from the composting of fish waste and carbon sources (Sanes et al., 2011). New, promising substrates are used for better crop productions systems in greenhouses (Carini et al., 2018; Valle et al., 2018).
This study aimed to measure the potential of different concentrations of solid residues from shrimp farming in the production of tomato seedlings, seeking organic development and a sustainable alternative to the shrimp industry.
MATERIALS AND METHODS
This experiment was implemented and conducted in the greenhouse of the Department of Agricultural Engineering of the Federal University of Ceara (UFC); the shrimp residues were supplied by the company Maris Pescados®, which is located in the city of Aracati-CE.
The shrimp (Litopenaeus vannamei) residues were washed in running water to remove any adhered impurities and separated into the following parts: 1- cephalothorax; 2- abdomen and 3- cephalothorax + abdomen (Fig. 1), which were placed on plastic sheets in the shade to dry for 7 d. Afterwards, they were crushed and stored in dark plastic bags. Three samples of shrimp residues were taken to the Chemical and Soil Fertility Laboratory of the Department of Soil Sciences/UFC, where they were chemically analyzed (macronutrients and micronutrients) in triplicate using the methodology described by Malavolta (1997). Because of the richness of nutrients in sample P3 (cephalothorax + abdomen), it was used as the source for the experiments.
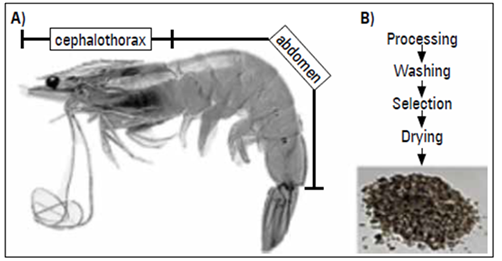
Figure 1. A) Parts of the shrimp (Litopenaeus vannamei) used as B) solid residues after processing (cephalothorax and abdomen).
Tomato cv. Santa Clara seeds were sown in trays containing a mixture of tanned manure, sand, and pine waste, with a 1: 1: 1 ratio, until the germination was uniform and development for transplantation was complete. Irrigation was carried out manually every day, during the morning and afternoon.
At 20 d after sowing, the seedlings were transplanted into 1,000 mL pots. Seedlings with greater vigor, uniform height that ranged from 10 to 15 cm, and 4 fully developed leaves were selected. The concentrations of shrimp residues (cephalothorax + abdomen) were mixed with coconut powder, creating the following treatments: coconut powder (control), 5, 10, 15 and 20 g of shrimp residue; each treatment was repeated 8 times.
At the 50th day, the following evaluations were performed:
Phytotechnical characteristics.All seedlings were evaluated for leaf area (cm2), stem diameter (mm), and fresh and dry weight of the shoot (g). The dry weight of the plant material was determined with drying in an oven at 60 ºC, for 72 h, until constant weight. At 7, 14, 21 and 28 d after transplanting, all seedlings were assessed for number of leaves and shoot length.
X-ray microanalysis.X-ray microanalysis was used to quantify nutrients, performed at the Central Analytical Laboratory/UFC. The leaves of the middle third were randomly collected from three different plants in the five treatments, totaling three leaves per treatment. These fragments were fixed in stubs with the help of double-sided carbon adhesive tape and identified. Then, they were analyzed using a scanning electron microscope (LEO EVO 40 XVP-Leo Electron Microscopy, Cambridge, UK) coupled to an X-ray microanalysis detection system (EDS X-Flash Detector 5010) (Bruker, Berlin), following the protocol described by Alves and Perina (2012).
Physiological characteristics. Physiological characteristics of the plants were evaluated using an infrared gas exchange analyzer (IRGA) Lci ADC-PLUS-5. Leaves that were completely expanded in three plants per treatment were selected, starting at 9:00 AM, with the density of photosynthetically active photon flow fixed in the apparatus chamber at 1,500 μmol m-2 s-1. The photosynthetic rate (μmol m-2 s-1), transpiratory rate (μmol m-2 s-1), stomatal conductance (μmol m-2 s-1) and internal carbon (μmol) were evaluated.
Experiment design and statistical analysis. The experiment design was completely randomized, with 5 treatments and 8 replicates. Each replicate had one pot containing one seedling. The data were submitted to analysis of variance, and, when there was significance, the means were submitted to a regression analysis, using the statistical program Assistat 7.7 (Silva and Azevedo, 2016).
RESULTS AND DISCUSSION
According to the chemical analysis, the three parts of the solid shrimp farming residues were rich in macro and micronutrients, such as calcium (Ca), phosphorus (P), potassium (K), sulfur (S), iron (Fe), zinc (Zn), magnesium (Mg) and sodium (Na) (Tab. 1). According to Bezerra et al. (2017) and Tacon (1989), shrimp head silage is a good source of several mineral nutrients, both for fertilization in plants and the manufacture of feed and food. Nocentini et al. (2021) stated that chitin residues generated from shrimp parts can be recycled for use in soils lacking in nutrients such as N and P.
Table 1. Chemical composition of nutrients of different parts of solid shrimp waste. P1) Cephalothorax, P2) Abdomen, and P3) Cephalothorax + Abdomen.
Parts | N | P | K | Ca | Mg | S |
---|---|---|---|---|---|---|
g kg-1 | ||||||
P1 | 0.42 b | 15.53 a | 3.84 a | 40.99 c | 3.16 b | 17.59 a |
P2 | 0.53 a | 16.14 a | 4.58 a | 43.20 b | 4.72 a | 18.28 a |
P3 | 0.59 a | 16.38 a | 4.77 a | 47.76 a | 4.87 a | 18.95 a |
Na | Fe | Zn | Cu | Mn | ||
mg kg-1 | ||||||
P1 | 4.56 b | 229.88 c | 81.51 c | 19.35 b | 6.10 b | |
P2 | 5.88 a | 262.15 b | 85.71 b | 37.88 a | 11.15 a | |
P3 | 6.58 a | 270.09 a | 90.33 a | 39.01 a | 12.04 a |
Means followed by the same letter in the columns do not differ according to the Scott-Knott test (P<0.05).
The P3 sample (cephalothorax + abdomen) was statistically superior to the other samples for Ca (47.76 g kg-1 - 4.77%), Fe (270.09 g kg-1 - 27.01%) and Zn (90.33 mg kg-1 - 9.03%). For P (~ 16.02 g kg-1 - 1.60%), K (~ 3.40 g kg-1 - 0.34%) and S (~ 18.27 g kg-1 - 1.83%), there was no statistical difference between treatments. In a study with dry silage flour from shrimp heads, Guilherme et al. (2007) obtained contents of 4.49 mg g-1 (4.49%) of Ca and 0.40 mg g-1 (0.40%) of P; the latter was much lower than that obtained in this study. Lima et al. (2007) found 4.70% Ca and 1.44% P in shrimp flour dry matter, very close to the instant findings.
Physical-chemical oscillations are common throughout the day and seasons according to the amount of available light and temperature in the environment (Arias, 2011). These possible differences may also be related to age, species and food management, varying by region. Silva et al. (2006) stated differences found in nutrients result from many food processing operations that can directly or indirectly alter the level or chemical form of nutrients or their association with other components of the food. Chaikaewet al. (2019) found that differences in the concentration of nutrients result from the type of system used in production, such as intensive and semi-intensive, which discharge more nutrients than the extensive system.
According to the phytotechnical evaluations of the tomato plants, the number of leaves increased by 68% when 8.0 g kg-1 of shrimp residue was incorporated into the substrate, as compared to the control treatment at 28 d of cultivation (Fig. 2). Bezerra et al. (2017) obtained similar responses when working with shrimp residue in sunflowers (Helianthus annuus), where the number of leaves increased by 37% with concentrations of 7 g and 14 g of the residue in the substrate, as compared to the control at 19 d of cultivation.
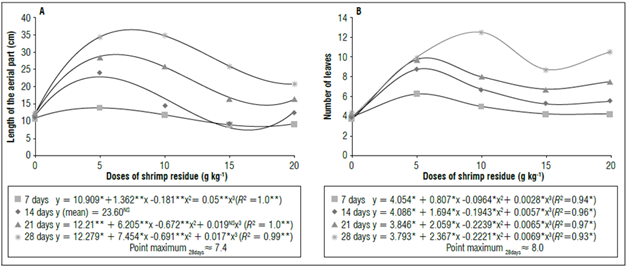
Figure 2. Doses of shrimp residue at 7, 14, 21 and 28 days in cv. Santa Clara tomato seedlings. A) Length of the aerial part, B) Number of leaves. *, ** and NS = significant at 1% (P<0.01), 5% probability (P<0.05) and non-significant, respectively.
The length of the aerial part obtained a considerable increase up to the concentration of 7.4 g kg-1, followed by a decrease (Fig. 2A), demonstrating the importance of studying the concentration of shrimp residues in the development of seedlings. According to Ghini et al. (2006), concentrations of shrimp residues above 20% reduce the development of ginger plants (Zingiber officinalis), possibly because of phytotoxicity since it is a nutrient-rich material.
The leaf area had a quadratic increase up to the concentration of 10 g kg-1 of shrimp residue, followed by a decline as the concentration increased (Fig. 3A). Monte et al. (2013) observed an increase in leaf area in tomato seedlings when grown under favorable humidity conditions. However, in this study, all seedlings were irrigated frequently, without a water deficit.
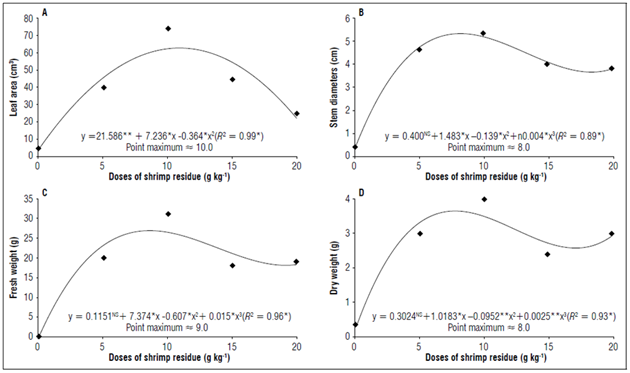
Figure 3. Phytotechnical characteristics in tomato seedlings cv. Santa Clara, at the 50th day, submitted to different doses of shrimp residues. A) Leaf area, B) Stem diameters, C) Fresh weight and D) Dry weight. *, ** and NS = significant at 1 % (P<0.01), 5% probability (p<0.05) and non-significant, respectively.
The diameters of the stems increased with 5 and 10 g kg-1, with a maximum point at 8 g kg-1 of shrimp residue (Fig. 3B). Subsequently, the diameters decreased in the other treatments. Armenta-Bojórquez et al. (2021) obtained similar results with tomato seedlings grafted in an integrated hydroponic system and shrimp cultivation, where the increase in salinity decreased the stem diameters of the plants. A larger stem diameter gives plants the ability to translocate a greater volume of nutrients and water to the aerial part, which would be used in vegetative growth, accumulation of biomass, and metabolic and photosynthetic processes (Mazzoni and Trufem, 2004; Albornoz et al., 2020). Greater tolerance to mechanical stresses, such as wind and precipitation, after transplanting to the field are also advantages of a larger stem diameter (Schwertner et al., 2013).
There was an increase in the fresh and dry weight up to the concentration of 9 g kg-1, demonstrating the importance of an adequate nutritional balance in the formation of seedlings (Silva Junior et al., 2014), which stimulates the physiological processes of plants, including photosynthesis (Cecato and Moreira, 2013).
Plants grown with different concentrations of shrimp residue showed a significant difference for the photosynthetic rate (A), transpiratory rate (E), and internal carbon (Ci). The stomatal conductance (gs) did not show significant differences. A and E were increased by adding 10 g kg-1 of shrimp residue, whereas the internal concentration of CO2 increased when using 5 g kg-1 of shrimp residue (Tab. 2).
Table 2. Gas exchange in tomato plants submitted to different doses of solid shrimp residues.
Treatments (g kg-1) | A | E | gs | C i |
---|---|---|---|---|
5 | 4.88 b | 3.43 ab | 0.185 a | 324.43 a |
10 | 16.33 a | 4.37 a | 0.200 a | 304.75 ab |
15 | 6.04 b | 2.93 b | 0.182 a | 306.50 ab |
20 | 7.64 b | 3.61 ab | 0.260 a | 297.33 b |
A = photosynthetic rate (μmol m-2 s-1), E = transpiratory (µmol m-2 s-1), gs = stomatal conductance (µmol m-2 s-1), C i = internal carbon (µmol). Means followed by the same letter in the columns do not differ according to the Scott-Knott test (P<0.05).
Increased photosynthetic capacity has been studies intensely because photosynthesis is the initial process for the production of biomass (Jiang and Xu, 2000). According to Morgado et al. (2013), leaf area affects not only the rates of photosynthesis and transpiration but also the growth rate, quality and fruit production in passion fruit (Passiflora edulis). A larger leaf area favors a greater light absorption capacity, and, consequently, the plant has a greater productivity gain, increasing profitability for the producer (Bispo et al., 2017).
For this variable, it was not possible to analyze the control treatment because the leaf area was much smaller than the coupling of the measuring device.
Photosynthesis corresponds to the basic energy of plant input, is essential for growth, and is directly linked to leaf morphology (Castro et al., 2009), which may vary according to the environment. The two main environmental limitations to photosynthetic rates are the availability of CO2 and radiation (Zhou and Han, 2005). According to Taiz and Zeiger (2017), the internal concentration of CO2 is important because plant productivity can be analyzed as the product of intercepted solar energy and CO2 fixed over a period.
Figure 4 shows the mapping of the elements of the adaxial surface of tomato leaves, showing uniform distribution of the elements. In the X-ray microanalysis, the concentrations of P, Ca, Mg, S, Na, K, carbon (C) and silicon (Si) were simultaneously detected in three samples. They are necessary for plant development and are found on the surface parts of solid shrimp waste. So, they can be used from the different parts (cephalothorax and abdomen), enabling good results since this material is rich in C, which favors increases in soil organic matter. According to Ghini et al. (2006), shrimp residues can contribute to nutrient cycling and sustainability of production systems, favoring increased organic matter and improving physical and chemical characteristics of soils.
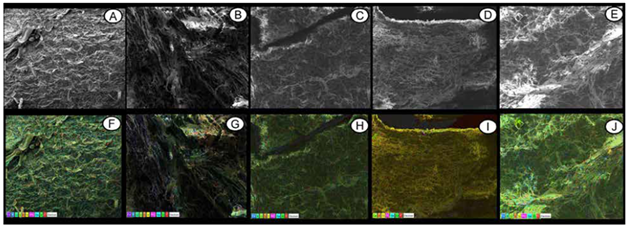
Figure 4. Scanning electron micrographs and mapping of elements on the adaxial surface of tomato leaves cv. Santa Clara at the 50th day of cultivation. A and F) control; B and G) 5 g kg-1 of shrimp residue; C and H) 10 g kg-1 of shrimp residue; D and I) 15 g kg-1 of shrimp residue, and E and J) 20 g kg-1 of shrimp residue. Bar = 250 µm.
In this analysis, Ca was found in all treatments and had a high amount in the 5 g kg-1 of shrimp residue (Fig. 5B). This result was explained by the fact that Ca is the third largest nutrient in shrimp residues according to the instant chemical analysis of the shrimp residues.
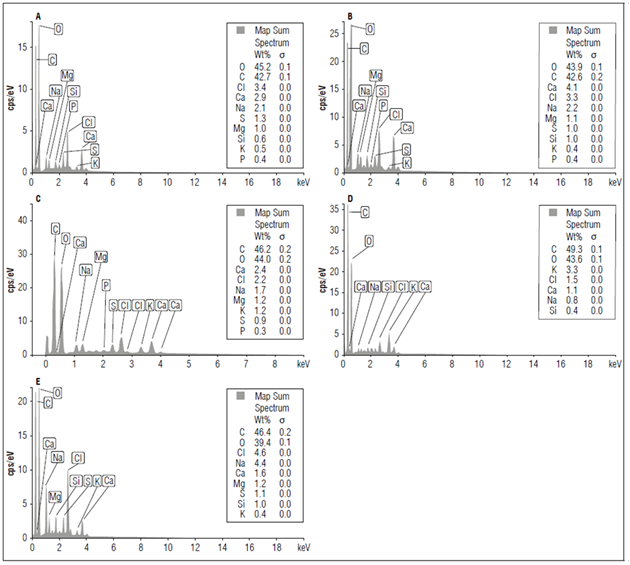
Figure 5. X-ray microanalysis of the adaxial surface of tomato cv. Santa Clara leaves at the 50th day of cultivation. A) control; B) 5 g; C) 10 g; D) 15 g and E) 20 g kg-1of shrimp residue. Scale of graphs: Total vertical 40 cps (captures per second).
Shrimp waste can be a new source of nutrients for tomato cultivation, with a lower risk of contamination by chemical waste. Furthermore, it is an easily available natural source with a low cost. However, other studies should be carried out on the influence of shrimp residues on fruit quality.
CONCLUSION
The use of P3 shrimp residue (cephalothorax + abdomen) is promising as a fertilizer on tomato plants, reducing the amount of material released to the environment and easing degradation.
The concentration of 10 g kg-1 of shrimp residue was efficient for the morphophysiological development of tomato cv. Santa Clara plants.