1. INTRODUCTION
The International Confederation of Thermal Analysis and Calorimetry 1 defines "Thermal Analysis" as a set of techniques where a physical or chemical proper ty is determined and controlled in function of time, temperature or heat flow, depending on the system temperature, that high enough (isothermal operation) it is maintained or amending linearly.
This definition and terminology associated had been also accepted by the International Union of Pure and Applied Chemistry (IUPAC) 2 and the American So ciety of Testing and Materials (ASTM E473-14).
Nowadays different procedures exist to examine and quantify the effect of the addition of heat to the physi cal system properties. Some of the techniques are the Differential Thermal Analysis (DTA), Thermogravi metric Analysis (TGA) and the Differential Scanning Calorimetry (DSC), these above are considered "clas sical methods" of thermal analysis (1). Other techni ques such as the Mechanical Thermal Analysis (MTA), Dynamic Mechanical Analysis (AMD), Dielectric Analysis (DEA), Thermal Microscopy and the Con duction Calorimetry are called "modern techniques" of thermal analysis. These techniques are counting with the developing of high technology instrumenta tion to perform combined methods, focusing on struc tural, thermal, electrical and magnetic properties of materials 3.
Thermal analysis is an appropriate methodology to assess a wide range of properties of substances, many of them associated with a particular technique 4, whereby, the identification is made, the physical and chemical stability is checked, changes of phase are de tected and fundamental kinetic studies are performed. Furthermore, thermal analysis permits to find transi tion points (melting, sublimation, solidification), make measurements of enthalpy and build thermal history, determine the heat capacity (specific heat), quantify thermal expansion (diagometria), establish mass loss (gravimetry), estimate voltages flow (rheology), per form studies of viscosity, recognize the processing rate, quantify the amount of water (fisiadsorbida, crys tallization, dehydroxylation), track processes of dena turation, evaluate the elastic modulus and damping capacity, examine the evolution of gases and volatile compounds, obtain phase diagrams, measure the crys tallinity, determine purity, investigate polymorphic transitions and make studies of hardness, tempering, annealing, tempering and recrystallization 5.
Given the different fields of application of the techni ques of thermal analysis can be seen in the bibliogra phical references in the field of natural resources and raw materials, thermal analysis is applied to charac terize wood and fiber, minerals, fuels (coal, tars and, soils (rocks, clays), food and natural products 6,7 (fats, carbohydrates, proteins). In the area of che mical, thermal analysis is used to determine reaction enthalpies, heat capacity, energy phase transition, heat of combustion, binding energy, purity, thermal stability of inorganic compounds, complexes and metal ions in coordination polymers etc 8.
In the context of science and materials technology, a close relationship between synthesis (preparation and processing), the structure (composition), the (physical and chemical behavior) properties and functionality (delivery and performance) is recognized. The ther mal analysis, in its various form, is a set of very useful and crucial tools in the characterization and proces sing of natural materials (wood, fibers, leather, bone, plaster, clay, zeolites), metals and alloys (ferrous and nonferrous), ceramics 9 (oxidic and non-oxidic) and glasses 10 (siliceous, metallic and non-siliceous), electric and magnetic, liquid crystals, polymers 11,12 and plastic (elastomers and adhesives), paints and coatings, inks and dyes, powders and explosives, se miconductors and superconductors, dielectrics, and composites (metal matrix, ceramic matrix polymer matrix).
For each and every of this applications the literature makes extensive reference to the thermal analysis as a set of techniques useful in the identification, characte rization, exploration and technical application of phy sical systems 13,14.
Currently, thermal analysis methods continue to grow in two directions: On the one hand, these techniques are moving towards achieving more accurate, faster and ever smaller samples measured; this will give va luable information on the interfaces, the microstruc ture and morphology of the substances in condensed phase. Second, the thermal analysis techniques can be conjugated with other analytical methodologies, such as diffraction and X-ray scattering, microscopy, mechanical testing, electrical and magnetic characte rization, and some spectroscopies, to perform in situ or in experiments operating conditions, structural and dynamic studies.
2. THERMAL ANALYSIS
When a substance (system) is supplied (heating) or is withdrawn (cooling) thermal energy as heat, various changes can occur sequentially or in parallel; these changes include the synthesis reactions, decompo sitions and phase transitions, among which nucleation and crystal growth. After identifying the target system, thermal analysis makes use of a heat source, dispenses heat flow, monitors progress of temperature and builds temperature diagrams, differential tempe rature and mass loss, depending on the system tempe rature (you can use an inert gas as reference) or heat flow. The diagrams obtained are called thermograms. Beyond the classic calorimetry, represented in the existence of different devices (adiabatic calorimeter, isothermal or isoperibolicos) which are used to mea sure the enthalpy and other properties that are asso ciated with different thermodynamic processes (state changes, reactions, dissolution, immersion, crystalli zation), thermal analysis has modalities represented in the differential thermal analysis (DTA) 15, ther mogravimetric analysis (TGA) 16 and differential scanning calorimetry (DSC) 17 that currently are powerful tools for characterization of substances; additionally, in these configurations, thermal analysis can be performed with the system immersed in an at mosphere can be inert, oxidizing or reducing.
Under the consideration that some solids, especially upon presentation of finely divided or porous solids exhibit adsorption phenomena of gases and vapors, thermal analysis also has applications in which the adsorbed amount or the amount which reacts evalua ted (NH3, CO, CO2, SO2) in function of temperature. Generally, these types of analyzes are called adsorp tion, desorption or temperature programmed reac tions (TPA, TPD, TPR) 18. Thermal analysis also has a variant dedicated evaluate the mechanical behavior (toughness, hardness, resistance, fatigue) of the subs tances off the transfer of thermal energy; thus, thermo mechanical analysis (ATM) 19 is set.
In a practical sense, thermal analysis is a set of tech niques by which a property of matter is evaluated in function of time (or temperature) to the extent that the system temperature (in a specific atmosphere) is mo dified by dispensing heat transfer 11.
Thermoanalytical methods arise from the need to as sess the effects of thermal energy on the physical and chemical properties of matter. the simplest example of monitoring the effect of heat on the appearance of a substance constitutes the heating curve of a pure com pound. At first, control experiment is satisfied whether the heat flow which is directed towards the substance remains constant or that there is a heat gradient which is uniform over time. In figure 1 the simple scheme is illustrated a device for heating curves.
To the extent that heat flows into the system, the tem perature of the substance, initially assumed solid, will increase with time by describing a slope whose value depends on the magnitude of heat flow or gradient (ramp) temperature the heat source 20. Experience shows that certain transitions occur, which in practi ce corresponds to equilibrium conditions, the sample temperature remains constant for some time and then start a new drift. In figure 2 typical heating curve for a pure substance shown; the coordinates are evaluated in this case are the temperature of the sample and the time (of exposure to heating). In a model case, star ting from the solid state, the heating curve will exhi bit three outstanding and two mesas that match the points melting and boiling.
Under the assumption of a constant heat flux, an event that corresponds to an isothermal source, it is clear then that the highest region of the curve (the gaseous state) can only be achieved if the temperature of the heat source exceeds the boiling point of the substance. Thus, it is evident that melting enthalpies of vaporiza tion, and are proportional to the length of the respecti ve mesas. Similarly, since conditions corresponding to a gaseous state, a substance can be cooled to the solid state whereby, following the sample temperature ver sus time cooling curve is obtained. There are howe ver a set of instrumental and inherent to the handling of samples that affect the quality of the thermograms factors; among these factors stand linearity of the tem perature ramp, the type of crucibles, the responsive ness of the thermocouples, the particle size (glass or added), the packaging of solid and nature of the pre treatments the samples.
The situation becomes more complicated to the extent that the system under study has more than one com ponent whose behavior is different from the heat, but where the phase rule known plays an important 21 paper; this is,
F = C-P + 2 (1)
Where F is the degrees of freedom (variance, in the sense of invariant, univariate, bivariate,), C represents the number of chemically independent species in the system (component), P indicates the number of exis ting phases and 2, corresponding to the pressure and temperature variables.
3. DIFFERENTIAL THERMAL ANALYSIS
When the thermal behavior (v. Gr. Response to hea ting) of a pure substance, but with reference to a stable solid structure and high melting point (v.gr. quartz, corundum, silicon carbide, graphite) is evaluated, two heating curves are obtained, a linear (reference) and another, which highlights certain changes (melting, boiling and other transitions); Subtraction of these trends, equivalent to subtract (in each element of time) the reference temperature (Tr) the temperature of the substance (TS), which is plotted as a function of sys tem temperature, produces a thermogram in the afo rementioned changes which appear as peaks (signals, bands) endothermic. So, if the TS and Tr temperatures are measured simultaneously and the amount. DEL TA. T = (TS - Tr) is plotted as a function of temperature (T) of the system or the time, a technique called diffe rential thermal analysis is set (DTA) 22.
For a system that is composed of a mixture of subs tances or in which the substance may undergo various transformations by heat and atmosphere, the thermo gram is expressed through endothermic and exother mic, narrow or flared peaks, which may even overco me and move, situation analysis difficult. In figure 3 a hypothetical thermogram of DTA is shown. The peaks of symmetrical appearance, emerging from a base line shown parallel to the axis of the temperature; In many actual cases, the signals are not symmetrical, well re solved not arise, shoulders and the baseline trend is observed not fully linear.
Therefore, the appearance and the usefulness of a thermogram of differential thermal analysis depend on several factors. The heating rate, ie the magnitude of the heat flow from the source to the system, which results in a temperature gradient system versus time, also determines not only the shape but also the possi bility of a good separation for the signs. The presence of oxidizing, reducing or inert atmospheres static or flow impinges strongly on the number and position of the bands. The chemical nature of the substance (lability, stability, reactivity) also affects the shape and position of the peaks. Finally, the shape of the ther mogram can be affected by the presence in the sample of volatiles (water), and the same substances retained morphology (finely divided solid blocks, monocrys tals and porous solids).
Common endothermic processes that may experience a solid substance are sublimation and melting. Howe ver, in a thermogram are also visible some endother mic transitions corresponding to loss processes fisiad- sorbida water in the sample, decomposition of hy drates, carbonates and decomposition dehydroxylation surface; peak exotherm are mainly identified by crystallization, crystalline transformations, reactions formation of new phases, oxidation and combustion reactions 23. In this context, under standardized con figurations the heat source, the temperature control, the arrangement of the test substances and reference, the nature and conditions of the atmosphere and hea ting rate (temperature gradient system), it is possible to examine different standard substances in order to apply the results to the analysis of systems of practical interest (substances chemical, raw materials, natural products, metals and elastomers metal alloys, cera mics and glass, polymers, plastics and semiconduc tors, liquid crystals, food, pharmaceutical and compo site products) 24.
The DTA also quantifies the amount of heat absorbed or follows a substance during a transformation. In par ticular, the area under the curve (of an endothermic peak or exothermic), which produces a phase transi tion of first order, is directly proportional to the mass of substance involved and depends on the enthalpy of the process (eg latent heat, reaction enthalpy), the heat capacity of the substance and certain geometric factors (shape, particle size and degree of compaction of substances); in general, a peak area (AC) is given by the equality 25:
Where k is an associated thermal conductivity and constant c is a parameter related to the sample geome try and m is the mass. For a given mass k' it remains constant if the heating rate, particle size and location of the thermocouples in the dishes, which support the samples, are carefully controlled. Calibration stan dards against evaluates k'; if this factor is unknown, it is possible to measure ΔH.
Known the area under the curve, the mass and heat supplied is feasible to estimate the thermal conducti vity and the average heat capacity of the test substance or otherwise, determine the proportion that causes the transition. Still, differences in temperature (ΔT) can be very small, so in addition to using reference substan ces with known thermal history, attention should fo cus on the sensitivity and accuracy of the temperature measurement of the test substance.
High purity metals are used for calibration purposes. Metals that have low melting point and enthalpy of fusion, which is known with high precision, are used in practice. Greater use of metal is the Indian ( ΔH F = 28, 4512 J g-1, 156, 4°C melting point). Under certain conditions of heating and sensitivity rate, the calibra tion factor is:
4. THERMAL GRAVIMETRIC ANALYSIS
In 1915, K. Honda developed a tool that allows you to follow continuously, the physical and chemical trans formations of a substance through mass changes at high temperatures without cooling; this device thermo balance was called and marked the origin of the theory and practice of thermal gravimetric analysis 26,27.
When a substance is labile temperature; ie decompo ses or volatilizes in the presence of gases reacts so that the composition is altered, it is possible to examine the loss or mass gain depending on the system temperatu re 26. This technique is known as thermogravimetric analysis (TGA) can overcome the differential thermal analysis (DTA), whereby a set of thermograms fur ther phase transitions describe the possible existence of chemical transformations is obtained. In the ther mogravimetric analysis, the sample mass is recorded, controlled atmosphere (N2 or Ar), depending on the temperature (25-1500°C) or time, to the extent that the system temperature increases linearly (with gradients of 0 to 200 K min-1).
An instrument thermogravimetry consists of a very sensitive analytical balance (5 to 20 mg), an oven, a temperature programmer, a control system of atmos phere and a microprocessor (which serves to control, capture, display and data analysis). The temperature of the sample is read by thermocouples that are placed nearest sample 28.
In addition to the decomposition, oxidation and re duction, thermogravimetric analysis allows evalua ting the adsorption-desorption of gases on porous solids; furthermore, the thermogravimetric analysis (TGA) provides information on the thermal condi tions to obtain stable forms, since analysis is feasible mixtures. With the help of data analysis system, it is possible to derive a thermogram and get more precise information on the various changes taking place in the sample under analysis information.
Thermogravimetric analyzes are carried out under controlled atmospheres. The oxidizing atmosphere may contain air or oxygen; hydrogen and carbon mo noxide are reducing gases; nitrogen and noble gases (helium, argon and krypton) are inert environments. In certain cases, security protocols adjusted designs, corrosive atmospheres consisting of halogens (fluori ne, chlorine, bromine, sulfur dioxide, hydrogen cyani de and ammonia) are used; in other situations, the site of the sample is surrounded by an atmosphere genera ted by gases from the decomposition of the substances (carbon dioxide, water vapor, ammonia, nitrogen and sulfur gases, methane) 29.
In the thermogravimetric analysis (TGA) thermo grams represents the change (loss or mass gain) de pending on the system temperature; in certain desig ns, the gaseous fluid moves and is carried to a detector (infrared or mass spectrometry), whereby, accedes to a successful identification of substances that evolve as a result of heat treatment.
From a thermogram, quantification of the loss or gain of mass for some change can be done by horizontal projections on the axis of mass but in many cases (simultaneous or overlapping transformations) the appearance of thermogram does not allow direct gra phical determination; moreover, it is not always easy, to set the inflection points of the curves, which is not simple to locate the transition temperature. Then it uses the representation of the derivative of the mass as a function of temperature. The derivative thermogra vimetric analysis (ATGD), which overlies the thermal gravimetric analysis, is of great importance because it allows you to set temperatures, determine the maxi mum rate of mass loss points and thus perform kinetic studies of thermal transformations.
Finally, typical thermal gravimetric analysis applica tions relate to the evaluation of the chemical stability of the substances, the effect of the nature of the envi ronment and the quantitative determination of com ponents which volatilize at temperatures characteris tics 30.
5. DIFFERENTIAL SCANNING CALORIMETRY
The differential scanning calorimetry (DSC) is a method of thermal analysis in which the difference is measured between the amount of heat transferred to a test substance and the amount of heat supplied to a reference substance depending on temperatu re that is controlled by a programmer (20,31. There are two ways or instrumental designs for differences heat transferred in differential scanning calorimetry 32: (a) the method of differential scanning calori metry power offset 33 and (b) the method of diffe rential scanning calorimetry of heat flow 34. In both methods, the test substance and reference are put into capsules, crucibles or dishes that are placed directly on the heat source; in the form of compensated power furnaces are very small (light) and are inserted into heating blocks with temperature control, sample sup ports and reference contact thermometers are based on platinum resistance.
The method determines the difference between the amount of heat flowing to the sample and the amount of heat flowing to the reference, to the extent that the temperature is ramped. The reference substance is a capsule or unoccupied crucible of the same material and mass as the dish containing the substance of inter est; because one of the capsules containing the sample and the other not, the excess solid in the crucible of the sample requires more heat to achieve the temperature increase at the same rate as the reference temperatu re. Through heat oven shown is supplied to the test substance through the furnace crucible reference to re ference, to maintain equal temperatures; this behavior extends if the test substance transitions (endothermic or exothermic) such as synthesis, decomposition, crys tallization and phase transformations occur; that is, the instrument compensates the thermal energy requi red to maintain the same temperature in the capsules sample and reference 35.
In the differential scanning calorimetry heat flow, the heat flows into the sample and reference specimens arranged in dishes, through a thermoelectric disc shown in figure 4. The temperature of the thermoelec tric disc is modified with the help of a programmer control using a linear gradient. The temperatures of the capsules or sample dishes and reference (which is empty) are read with the help of thermocouples that are below the dishes. Under the assumption that the thermal conductivity of the system (disk heating me dia, capsules) is kept constant, it is admitted that the difference of heat transferred is directly proportional to the electrical potential output thermocouples surfa ce being located below cymbals. This heat difference (1 mW = 1mJs-1) is plotted against temperature is re corded with the thermocouple is located beneath the sample plate.
Experimentally, the differential scanning calorimetry measures differences in heat capacity, without mecha nical agitation, in continuous operation of adiabatic type, at a constant rate of temperature variation as a general rule, one can say that all transformations which is produced or consumed energy, they can be measured with this thermo-analytical technique. In differential scanning calorimetry, as in differential thermal analysis, the instrument calibration is a key to determine the temperature and to convert the transfe rred, absorbed or dissipated energy into useful units (watt joules) matter. The classic forms of calibrating equipment differential scanning calorimetry are Joule, providing a measure of power to magazines and re ference sample quantity and energy through fusion enthalpies 36.
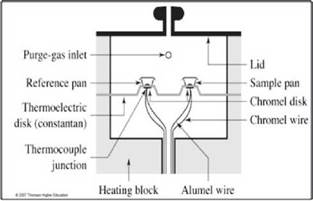
Figure 4 Simplified schematic of the sample chamber of a differential scanning calorimeter heat flow 37
Calibration by melting enthalpies involves the use of pure substances, which have phase transitions known temperatures; then, not only the amount of heat trans ferred but also the temperature is calibrated. Besides the substance must have a high degree of purity, the enthalpy of transition must be known precisely and must show no tendency to overheating. Additionally, to calibrate a wide experimental range of temperatu res is necessary to use several standard substances 38,39.
As suggested by equations (2) and (3), the peak area is directly proportional to the enthalpy change, through a calibration coefficient and the mass of the substance. Similar to the differential thermal analysis, differential scanning calorimetry in the term of enthalpy versus time has three components:
Where, the first element on the right represents the area under the curve, the second member contains contributions from the baseline and the third member is the slope of the peak; R is the thermal energy.
Among the various applications of the differential scanning calorimetry it is possible to cite measuring the apparent heat capacity, determining temperature characteristics of transition or transformation (glass transition paramagnetic transition, crystallization, polymorphism, melting, boiling, sublimation, decomposition and isomerization, etc.), thermal stability of substances and crystallization kinetics. Logically, to identify the type of transformation that takes place at a certain temperature, reference must be complemen tary to other experimental techniques.
6. CONCLUSION
In this literature review a basic outline of the different calorimetric techniques (DTA, TGA and DSC) and their applications in various fields is shown. It is in tended that this work is significant for those who wish to conduct research in the field of thermal characteri zation of materials input.