INTRODUCTION
Native to Brazil, Hancornia speciosa Gomes is a fruitful species of the dicotyledonous group, which belongs to the Apocynaceae family (Silva et al., 2017). The mangaba fruit exhibits great market potential in the tropical fruit sector, due to its wide variety of products and byproducts. It also has nutritional and pharmaceutical traits, such as a high vitamin C concentration (Santos et al., 2017). Moreover, studies show that mangaba has a high antioxidant activity (Rufino et al., 2009; Rufino et al., 2010), potentially reducing the incidence of cancer, as well as of cardiovascular and cerebrovascular diseases.
Plant tissue culture provides an alternative to the use of entire specimens to produce bioactive secondary metabolites, thus allowing for active environmental control, regardless of seasonal and geographical conditions (Cardoso et al., 2019). Alternatives to using plant material from natural resources are important, and the application of plant tissue culture techniques offers opportunities for a sustainable access to natural products (Wawrosch & Zotchev, 2021). One of the possibilities for producing valuable metabolites in vitro is the plant tissue callus induction method (Grąbkowska et al., 2016, Cardoso et al., 2019).
Plant growth regulators play a vital role in plant growth and development. Thus, 2,4-D (2-4 Dichlorophenoxyacetic acid), NAA (Naphthalene acetic acid), and other auxins are widely used for callus induction (Akram & Aftab, 2016). Studies have recently reported the in vitro formation of calluses in H. speciosa, concluding that NAA and BA regulators are superior for callogenesis induction when compared to others such as TDZ, IBA, and IAA (Prudente et al., 2016; Oliveira et al., 2016). However, information on the callogenesis potential of different mangaba accessions is scarce. Understanding the growth dynamics of plant cells is crucial in order to manipulate all related parameters, so that the potential of plants can be fully utilized (Puad & Abdullah, 2018).
The main objective of this research was to evaluate different combinations of 2,4-dichlorophenoxyacetic acid and benzylaminopurine for the induction of calluses in five mangaba accessions, establishing growth curves and performing histological analyzes.
MATERIAL AND METHODS
Location and plant material
Accessions from the Active Germplasm Bank of Mangaba, located in Itaporanga d’Ajuda, SE, Brazil (11°06'40''N and 37°11'15''E), were selected, given this bank’s ability to provide both mature fruits and information on the location of origin (Accessions: AB-Água Boa - Pará/0º46’31’’N; 48º31’05’’E; CA-Costa Azul - Bahia/11°33’32’’N; 47°47’06’’E; BI-Barra do Itariri -Bahia/11°48’39’’N; 37°36’40’’E; PT-Indiaroba - Sergipe/11°31’10”N; 37°30’47”E; TC-Terra Caída - Sergipe/11°46’31’’N; 37°30’47’’E). After harvesting the mature fruits of the selected accessions, they were pulped to obtain the seeds, which remained at room temperature for 24 hours. In a flow chamber, the seeds were submitted to asepsis with immersion in 70% alcohol for 60 seconds and in 2-2.5% sodium hypochlorite for 15 minutes, as well as to triple washing in sterile water. The sterile seeds were inoculated in an MS culture medium (Murashige & Skoog, 1962), with 30 g.L-1 sucrose and 3.5 g.L-1 Phytagel® (Ref. P8169, Sigma). The pH was adjusted to 5.8, and the medium was then autoclaved at 121 °C for 15 minutes. Explants obtained from in vitro aseptic seedlings were used to induce callogenesis.
The experiments were carried out at the Plant Tissue Culture Laboratory of Embrapa Tabuleiros Costeiros, in Aracaju, SE, Brazil, and at the Electronic Microscopy and Ultrastructural Analysis Laboratory of the Federal University of Lavras, MG, Brazil.
Induction of callogenesis
After 45 days of seed inoculation, internode and node segments (1 cm long) and foliar sections of the central leaf zone (0.5 cm2) were excised from the in vitro seedlings. For callus induction, the internode and nodal segments were inoculated in a longitudinal position, and the foliar section with abaxial side in contact with the MS culture medium. The medium was supplemented with 30 g.L-1 sucrose and 3.5 g.L-1 Phytagel® (Ref. P8169, Sigma) containing respectively different combinations of 2,4-dichlorophenoxyacetic acid (2,4-D-Ref. D7299, Sigma) and benzylaminopurine (BA-Ref. B3408, Sigma). The pH of the media was adjusted to 5.8 before autoclaving at 121 °C for 15 minutes.
The explants were weighed at 10, 20, 30, 40, and 60 days of in vitro culture, so the callus mass could be measured. The cultures were maintained in a growth room at 25±2 °C, in the absence of light to avoid phenolic exudation (Amente & Chimdessa, 2021) throughout the experimental period.
The experiment was completely randomized; it employed a 5 (accessions) × 3 (explants) × 5 (growth regulator treatments) × 5 (days) factorial design. Each treatment had four replicates, each containing one explant. The data were subjected to an analysis of variance, and a Scott-Knott test at 5% probability was performed on the means of the qualitative treatments, which were also subjected to a regression analysis.
For the statistical analyses, due to the absence of callus formation in T0 and their high oxidation in T4 treatments, only three combinations of growth regulators were considered for callus mass (g) evaluation. The SISVAR statistical program (Ferreira, 2019) was used.
Plotting the callus growth curves
To determine the callus growth curves originating from internode, node, and leaf explants, explants were grown in a culture medium able to provide maximum callus production.
The calluses were weighed on a precision scale, on the first day of inoculation (time 0) and then in 10-day intervals for 60 days, with three replicates per evaluation time. Each replicate consisted of a Petri dish with four explants. The growth curve was plotted from the average of the three measurements of fresh mass for each evaluation time (0, 10, 20, 30, 40, and 60 days).
Histological studies
This study followed the methodology of Alves et al. (2008). For the histological test, callus samples from different accessions and types of explants (node, internode, and leaf segments) were used. The samples were kept in Karnovsky’s modified solution (1965). Dehydration was performed using graded ethyl alcohol series. Then, the callus was infiltrated in pure resin and embedded in Leica® Historesin. The slides were analyzed under an optical microscope (Zeiss/Jenamed2, Germany), in which photomicrographs (Axiocam software, Zeiss, Germany) of the prepared slides were obtained.
The analysis of the callus samples under a scanning electron microscope also employed the methodology developed by Alves et al. (2008). To fix the samples, a glutaraldehyde (25%) (Ref. 104239, Merck), paraformaldehyde (16%) (Ref. P6148, Sigma-Aldrich), sodium cacodylate buffer (0.2 M, pH 7.2) (Ref. C0250, Sigma), CaCl2 (0.1 M) (Ref. C1016, Sigma-Aldrich), and distilled water (Karnovsky, 1965 modified) solution was used. For the assays, the samples were washed in 0.05 M cacodylate buffer and post fixed in 1% osmium tetroxide (Ref.251755, Sigma-Aldrich®). Afterwards, they were progressively dehydrated in acetone subjected to the critical point of CO2 desiccation in a BAL-TEC equipment, CPD-030 (Bal-Tec, Los Angeles, California, USA), fixed to a metallic support and covered with metallic gold (10 nm) (01O1003.01.AA, Synth ©) while using a BAL-TEC apparatus, SCD-050 (Bal-Tec, Los Angeles, California, USA). The sample was prepared, observed, and electromicrographed in a LEO EVO 40 XVP scanning electron microscope (Carl Zeiss AG, Germany).
RESULTS
In vitro callus induction
Regarding callus mass, a significant difference in interaction between the growth regulator treatment and the accession was observed. There was no significant effect of the explant type on callus mass. Among the accessions studied, no statistical difference was identified.
Callus fresh mass had a linear and positive behavior with respect to all factors combined. There was a greater mass increase over time in the 45.25 µM 2,4-D and 11.10 µM BA (T3) combination for all accessions (Table 1). The highest callus mass was observed in the BI accession at 60 days, in the presence of 22.62 µM 2,4-D and 11.10 µM BA. Under the same conditions, BI had a callus mass of approximately 1.4 g, in comparison with 0.1 g in the AB accession. In all treatments, a friable callus of light-yellow color was observed at all evaluation times.
Table 1 Effect of growth regulators on the formation of fresh callus mass (g) in mangaba accessions at 10, 20, 30, 40, and 60 days of in vitro culture. (T1) 4.52 μM 2,4-D + 2.22 μM BA; (T2) 22.62 μM 2,4-D + 11.10 μM BA; (T3) 45.25 μM 2,4-D + 22.20 μM BA. Accessions: Água Boa (AB); Barra do Itariri (BI); Costa Azul (CA); Pontal (PT); Terra Caída (TC).
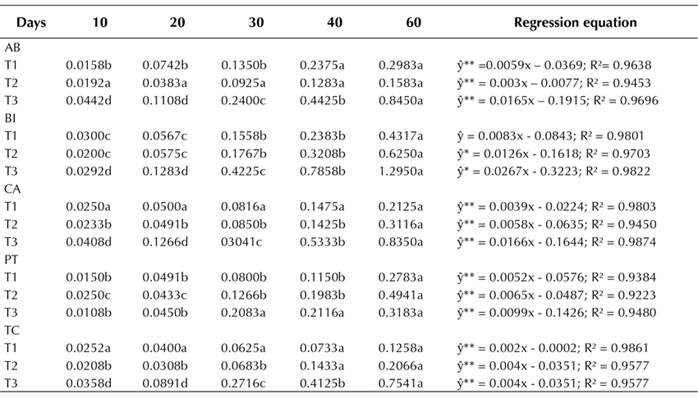
Callus growth curve
To plot the callus growth curve from different explants (leaf, node, and internode segments) evaluated for up to 60 days of in vitro culture, samples induced in the presence of 45.25 µM 2,4-D + 22.20 µM BA were used, as well as accessions with the greatest contribution to the accumulation of callus fresh mass (Table 1) as absolute values. Different stages of growth in induced callogenesis were evaluated in different explants during the 60 days of culture (Figure 1).
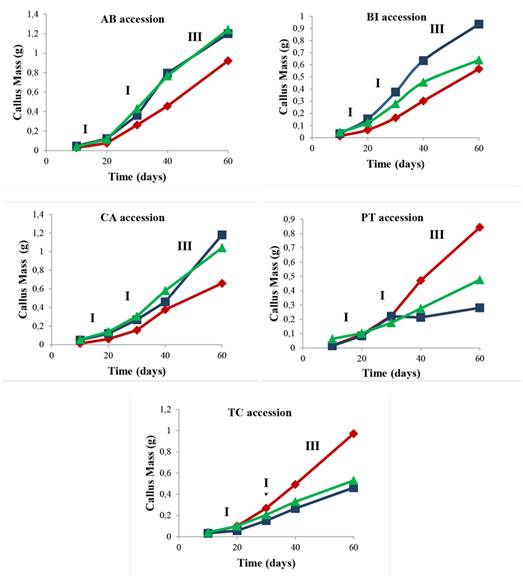
Figure 1 Callus growth in mangaba explants (Leaf ♦, Node ■, and Internode ▲) of AB, BI, CA, PT, and TC accessions in an MS medium with 45.25 μM 2,4-D + 22.20 μM BA at 10, 20, 30, 40, and 60 days of culture. I - Lag phase, II - Exponential phase and III - Linear phase. Accessions: Água Boa (AB); Barra do Itariri (BI); Costa Azul (CA); Pontal (PT); Terra Caída (TC).
There is a difference in the callus mass between the evaluated explant types. In all the studied accessions, the node explant had a greater callus mass formation. In the AB, BI, CA, PT, and TC accessions, phases I (10 to 20 days), II (20 to 40 days), and III (40 to 60 days) were reached within the same experimental periods. The stationary and decline or deceleration phases were not observed until 60 days had passed.
Histological analysis
The callus observed in all treatments were friable and yellowish and had increasing mass increments (g). The following morphological structures were observed in BI accession, which are also identified in Figure 2: round agglomerated cells (rac) - rounded cells, dispersed or associated with agglomerated cells; agglomerated elongated cells (aec) - elongated cells, dispersed or associated with cellular agglomerations; small agglomerated cells (sac) - formed by small and isodiametric cells; small scattered (sc) cells - comprised of small, dispersed cells; and vascular bundle (vb) - an anatomical structure of the stem. Cells in dedifferentiation were observed in all the studied accessions.
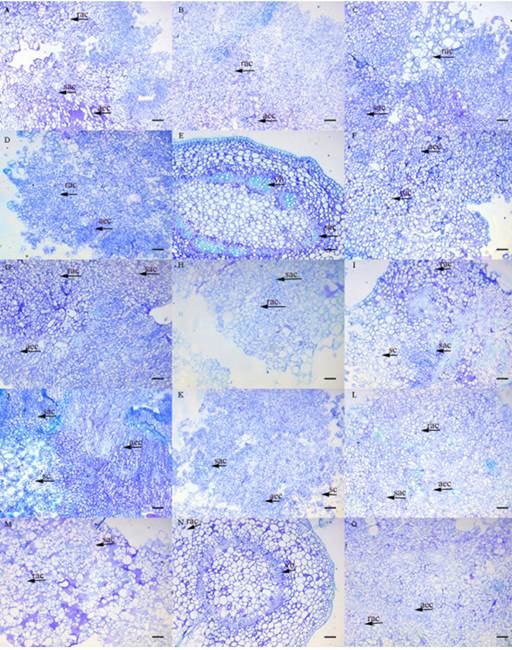
Figure 2 Anatomical structures in different mangaba in vitro cultures after 30 days in an MS medium supplemented with 45.25 μM 2,4-D + 22.20 μM BA. (A) foliar AB; (B) internode AB; (C) node AB; (D) foliar BI; (E) internode BI; (F) node BI; (G) foliar CA; (H) internode CA; (I) node CA; (J) foliar PT; (K) internode PT; (L) node PT; (M) foliar TC; (N) internode TC; (O) node TC. Bar 100 μm. Type: (rac) round agglomerated cells, rounded cells dispersed or associated with cellular clusters; (aec) agglomerated elongated cells, elongated cells dispersed or associated with cell agglomerates; (sac) small, agglomerated cells, small and isodiametric cells, forming agglomerates; (sc) small scattered, formed by small and dispersed cells; (vb) vascular bundle, structure of the vascular bundle of the stem. Accessions: Água Boa (AB); Barra do Itariri (BI); Costa Azul (CA); Pontal (PT); Terra Caída (TC).
In the leaf segment, rac and aec cells were observed (Figure 2D). The callus from the node segment (Figure 2E) had sac cells and a vb anatomical structure, with the beginning of dedifferentiated cell formation. This type of cell was also observed in all studied accessions of H. speciosa. Regarding the formation of callus from internode segments (Figure 2E, N), there was an initial development of dedifferentiated cells, and it was possible to identify the other anatomical structures present in the plant stem area. The callus exhibited a similar pigmentation and consistency across accessions, despite the fact that different treatments modified the yellow coloration intensity.
DISCUSSION
In vitro callus induction
In vitro callus induction depends on several biotic and abiotic factors such as culture medium composition, plant growth regulators, sucrose compositions, pH, and incubation conditions. These factors may influence the induction potential and morphology of callogenesis (Osman et al., 2016). Callus induction from different explants of Mandevilla guanabarica (Apocynaceae) (apical meristems, leaf, node segments, internode segments, roots, and epicotyl and hypocotyl) was performed by Cordeiro et al. (2014), who evaluated cell development in different explants and culture medium conditions. Their study is a good reference for the selection of the explants and growth regulators able to induce higher callus growth rates.
Mustafa et al. (2011) reported that friable callus is mainly used for cells under suspension and for the study of metabolites, given that, in a culture medium, the cells rapidly divide and disperse. Auxins are capable of initiating cell division and controlling the processes of cell growth and elongation (Perrot-Rechenmann, 2010). The combination of endogenous phytohormones and 7.74 µM 2,4-D in a WPM culture medium stimulated the cell division of H. speciosa parenchyma regions, forming callus from internode segments (Prudente et al., 2016). Callus induced from the leaf, young stem, and shoot tip of Melientha suavis Pierre were observed by Premjet et al. (2020) in an MS basal medium supplemented with various formulations of 2,4-D and 6-BA. Another promising auxin in the induction of callus in species of the family Apocynaceae is NAA. Studies with the apical parts of Rauwolfia serpentina L. in MS media enriched with NAA resulted in excellent callus development (Varnika et al., 2020). The balance between auxin and cytokinin fosters a greater callus mass in different segments of the plant. The color and texture of the callus varied depending on the type of explants, as well as on the concentrations of growth regulators used in both media (Das et al., 2018). Therefore, more studies are needed to define the growth regulator.
Callus growth curve
Callus growth curves have five phases: lag (I), exponential (II), linear (III), stationary (IV), and deceleration (V) (Bathia et al., 2015). In the AB, BI, CA, PT, and TC accessions, phases I (10 to 20 days), II (20 to 40 days), and III (40 to 60 days) were reached within the same experimental periods. The stationary and decline or deceleration phases were not observed until 60 days had gone by. Thus, determining the in vitro callus growth curve is important for understanding the time of maximum mass increment (in days).
In studies with Aspidosperma cylindrocarpon (Apocynaceae), cell suspension cultures showed a four-day growth lag phase for 15 days, and, after 20 days, the cells showed a stationary growth (Cornelio, 2018). The growth acceleration of Bletilla striata callus started after seven days, gradually decreasing thereafter until day 24 of the cultivation period (Pan et al., 2020). This result is an important contribution to in vitro studies on mangaba cell suspensions, and it indicates the high induction and proliferation capacity of in vitro callus mass over long periods of time.
Histological analysis
All studied cell types are more likely to form embryogenic callus in Byrsonima verbascifolia (Malpighiaceae) (Chiavegatto et al., 2015). Oliveira et al. (2016) also observed the formation of friable calluses on the basis of node segments with variation in callus pigmentation as a function of the growth regulator concentration. H. speciosa callus cultivated in WPM media in the presence of BA and 2,4-D were friable and easy to dissociate, and they initially exhibited a white coloration (Prudente et al., 2016).
In histological studies on callus of Asteracantha longifolia Nees, Kumar and Nandi (2015) observed that the meristematic regions were formed by small cells with densely agglomerated nuclei. These aec-like cell structures were observed in this research when inducing callus in the node segment of the PT accession (Figures B and C). Bhagya and Chandrashekar (2013) detected the initial formation of callus from the exchange region of C. peltata stems, with disorganized cells arranged around a prominent nucleus. The results obtained in this work for H. speciosa are important due to the lack of studies on callogenesis and histology in different accessions.
CONCLUSIONS
After 60 days of in vitro culture, all treatments exhibited a linear positive cellular growth. The BI accession showed the maximum increase in callus mass in the presence of 22.62 µM 2,4-D and 11.10 µM BA. The callus mass from the foliar section and the node and internode segments in the MS medium with 45.25 µM 2,4-D + 5.0 mg.L-1 BA induced morphological alterations in the AB, BI, CA, TC, and PT accessions.