Services on Demand
Journal
Article
Indicators
-
Cited by SciELO
-
Access statistics
Related links
-
Cited by Google
-
Similars in SciELO
-
Similars in Google
Share
Revista Colombiana de Química
Print version ISSN 0120-2804On-line version ISSN 2357-3791
Rev.Colomb.Quim. vol.35 no.2 Bogotá Jul./Dec. 2006
Mary Trujillo 1 Jesús Valencia 2 Julio C. Calvo 3
1Departamento de Farmacia, Facultad de Ciencias, Universidad Nacional de Colombia, sede Bogotá, Bogotá, Colombia. mtrujillog@unal.edu.co
2Departamento de Química, Facultad de Ciencias, Universidad Nacional de Colombia, sede Bogotá, Bogotá, Colombia
3Grupo PROTEOMA, Facultad de Ciencias y Educación, Universidad Distrital Francisco José de Caldas, Bogotá, Colombia. jccalvom@udistrital.edu.co
ABSTRACT
Solubility, structure and position of charges in a peptide antigen sequence can be mentioned as being amongst the basic features of adsorption. In order to study their effect on adsorption, seven analogue series were synthesized from a MSP-1 peptide sequence by systematically replacing each one of the positions in the peptide sequence by aspartic acid, glutamic acid, serine, alanine, asparagine, glutamine or lysine. Such modifications in analogue peptide sequences showed a non-regular tendency regarding solubility and adsorption data. Aspartic acid and Glutamic acid analogue series showed great improvements in adsorption, especially in peptides where Lysine in position 6 and Arginine in position 13 were replaced. Solubility of position 5 analogue was greater than the position 6 analogue in Aspartic acid series; however, the position 6 analogue showed best adsorption results whilst the Aspartic acid in position 5 analogue showed no adsorption in the same conditions. Nuclear Magnetic Resonance structural analysis revealed differences in the -helical structure extension between these analogues. The Aspartic acid in position 6, located in the polar side of the helix, may allow this analogue to fit better onto the adsorption regions suggesting that the local electrostatic charge is responsible for this behavior.
Key words: Adsorption, aluminium hydroxide, peptide analogues, solubility and adsorption, structure and adsorption, charge position and adsorption.
RESUMEN
La solubilidad, la estructura y la posición de las cargas en una secuencia de un péptido antígeno, se encuentran entre las características básicas de la adsorción. Con el fin de estudiar su efecto sobre la adsorción, fueron sintetizadas siete series de análogos de la secuencia de un péptido de la proteína MSP-1, reemplazando sistemáticamente cada una de las posiciones en la secuencia del péptido por ácido aspártico, ácido glutámico, serina, alanina, asparagina, glutamina o lisina. Las modificaciones en las secuencias de los péptidos análogos no mostraron tendencias regulares respecto a los datos de solubilidad y adsorción. Las series de análogos de ácido aspártico y ácido glutámico presentaron grandes incrementos en la adsorción, en especial cuando fueron reemplazadas la lisina de la posición 6 y la arginina de la posición 13. La solubilidad del análogo en posición 5 fue mayor que la del análogo en posición 6 en la serie del ácido aspártico; sin embargo, los mejores resultados en adsorción se obtuvieron al sustituir con ácido aspártico la posición 6, mientras que el análogo con el ácido aspártico en la posición 5 no presentó adsorción a las mismas condiciones. El análisis estructural por resonancia magnética nuclear mostró diferencias en la extensión de la estructura helicoidal entre estos análogos. El ácido aspártico en la posición 6, localizado en la cara polar de la hélice, podría permitir a este análogo ajustarse mejor sobre los sitios de adsorción, sugiriendo que la carga electrostática local es la responsable de este comportamiento.
Palabras clave: adsorción, hidróxido de aluminio, péptidos análogos, solubilidad y adsorción, estructura y adsorción, posición de carga y adsorción.
INTRODUCTION
Aluminium-containing adjuvants have been widely used because of their safety in humans and are currently the only ones being included in vaccines. Aluminium hydroxide or aluminium phosphate is commonly used as adsorbent for antigens. Hem et al have done a lot of excellent work during the last few decades on these adsorbents’ chemical structure and the conditions for protein adsorption (1-5). The aluminium hydroxide adjuvant used in vaccines has been identified as boehmite, a crystalline aluminium oxyhydroxide, and the aluminium phosphate as amorphous aluminium hydroxyphosphate (6). These gels’ properties (such as surface area, surface charge, chemical composition and chemical structure) depend very strongly upon the production process (7).
One goal in formulating and manufacturing vaccines containing aluminium hydroxide or aluminium phosphate is for the adjuvant to adsorb the antigen. Aluminium hydroxide adsorbing proteins has been neglected, even though aluminium- containing adjuvant has been used in vaccines for decades. Electrostatic attraction, hydrophobic attraction and ligand exchange represent the major mechanisms responsible for aluminiumcontaining adjuvants adsorbing antigens (4-6). Electrostatic attraction is the most frequently encountered adsorption mechanism, as both antigens and adjuvants have a pH-dependent surface charge. Knowing the antigen and adjuvant pI, as well as the pH, allows to predict the degree of adsorption that will occur when vaccines are prepared (1, 3). However, examining pI and pH values may not be useful in predicting a complete vaccines physical properties (8). Physical properties will likely depend on the antigen-adjuvant complexs surface charge characteristics.
Optimum formulation of vaccines containing multivalent antigens may require more than a simple type of aluminium- containing adjuvant. A positively charged adjuvant such as aluminium hydroxide can be used (1, 3) to maximize negatively charged antigen binding in some cases; a negatively charged adjuvant such as aluminium phosphate might be preferred in others if the antigen were positively charged (1, 9). The multivalent vaccine would therefore be prepared by combining individual monovalent bulks resulting in a suspension consisting of mixed aluminium-containing adjuvants (8). Questions arise regarding the potential for redistributing antigens and adsorbed phosphate anions as well as multivalent vaccine colloidal stability using different aluminium-containing adjuvants.
Many factors may influence the physicochemical antigen adsorption process in producing adsorbed vaccines. It has thus become necessary to develop new designs for introducing combined vaccines, and besides there are a lot of as yet unknown or not understood immunological interactions between these vaccine components.
In this work, seven analogues series were synthesized from a well known peptide sequence (10) by serially replacing each of the amino acids in the target sequence by aspartic acid (Asp), glutamic acid (Glu), serine (Ser), alanine (Ala), asparagine (Asn), glutamine (Gln) or lysine (Lys). Solubility and adsorption onto aluminium hydroxide were tested for each of the analogue series. No correlation was found between solubility and adsorption. Asp and Glu analogue series showed great improvements in adsorption, especially in peptides where Lysine in position 6 and Arginine in position 13 were replaced. The Asp-5 analogues solubility (analogue where the position 5 was replaced by Asp) was greater than the Asp-6 analogue (analogue where the position 6 was replaced by Asp); however, the Asp-6 peptide showed the best results in the adsorption test. CD and NMR structural analysis revealed no significant structural differences between these analogues, suggesting that the local electrostatic charge is responsible for this behavior.
MATERIALS AND METHODS
Analogue series design and peptide synthesis
Based on the malarial MSP-1 protein 1585 peptide sequence (EVLYLKPLA GVYRSLKKQLE), sets of peptides were designed by replacing each one of the 20 amino acids in the sequence by Asp (D), Glu (E), Asn (N), Gln (Q), Lys (K), Ser (S) or Ala (A). A total of seven peptide analogue series were obtained. Table 1 shows the Asp analogue set of peptides as an example. The same procedure was followed for the Glu, Asn, Gln, Lys, Ser or Ala analogue sets of peptides.
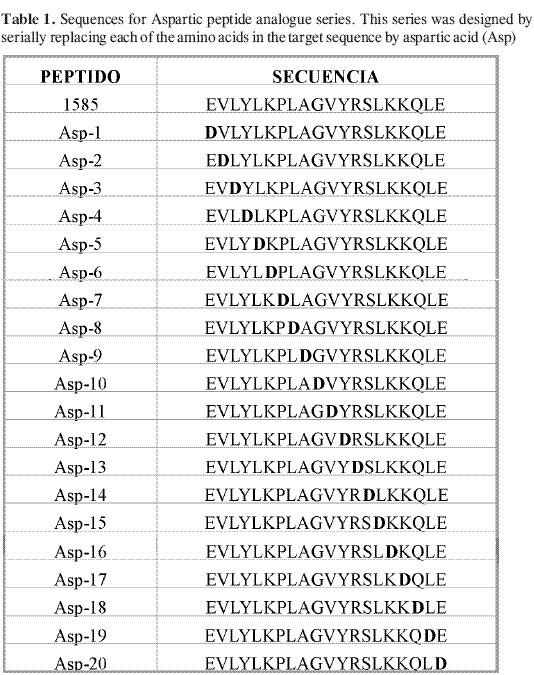
Peptide 1585 and its 20-mer peptide analogues were synthesized by using standard t-boc solid phase peptide synthesis (SPPS) (11) and crude peptides purified by RP-HPLC on a 218TP1022 Vydac column. Peptide purity was verified by RP-HPLC on an analytical Lichrosorb C18 (MERCK) using a 0%-70% B gradient over 30 minutes. Solvent A was 0.05% TFA in water and solvent B was 0.05% TFA in acetonitrile. The products molecular mass was determined by using a Bruker Protein Maldi-TOF mass spectrometer.
Solubility assays
Peptide solubility was determined by saturating 1 mL 0.9% saline solution at 25 °C, 7 ±0.1 pH. Dissolved peptide was measured by Multicelda Labsystem spectrophotometer using micro-bicinchoninic acid protein assay procedure (Pierce) (12). The standard protocol was used and calibration curves drawn for each peptide analogue.
Adsorption
Equilibrium time for peptide 1585 adsorption on aluminium hydroxide was determined by preparing 12 samples of a 4 mg/mL peptide solution in 0.9% NaCl, 7±0.1 pH. An aluminium hydroxide aqueous suspension (ALHIDROGEL) was added to each sample in quantities equivalent to 1.6 mg Al/mL (13), keeping the temperature constant at 273 K. The resulting mixtures were gently shaken at 150 rpm during the whole experiment. Samples were taken each 10 minutes during the first hour and then each hour until 6 hours had been completed to evaluate adsorption regarding time. The samples were spun at 8,000 rpm and filtered through 0.22µm membrane to determine peptide concentration before and after adsorption using bicinchoninic acid (BCA), as indicated in the solubility test. The quantity adsorbed was established in mmol/mg Al by the difference between these two concentrations.
Adsorption experiments for different analogue series were done in the already described conditions by shaking the mixture at 273 K for 30 minutes in a helicoidal agitator. Adsorption isotherms were established using concentrations of each peptide ranging from 0 to 3.2 mmol/mL in 0.9% NaCl, 7±0.1 pH and 273 K. Each sample contained a quantity of adjuvant equivalent to 1.6 mg Al/mL. Known amounts of peptide analogue and aluminium hydroxide were mixed in an end-over-end rotator for 30 minutes. The mixtures were then centrifuged and the protein concentration in the clear supernatant was measured by Multicelda Labsystem spectrophotometer using the micro-bicinchoninic acid protein assay procedure (Pierce) (12). The amount of adsorbed protein was determined from the difference between the peptide concentrations in solution before and after adsorption. The amount of peptide adsorbed per unit area of adsorbent surface was plotted against the peptide concentration in solution, following adsorption.
NMR spectroscopy
Samples for all NMR experiments were prepared by dissolving 8 mg peptide in 500 µL TFE-d3/H2O mixture (30/70 (v/v)). A BRUKER DRX-600 spectrometer was used to obtain 1H NMR spectra. Proton spectra were assigned by using double-quantum filter COSY (DQF-COSY) and TOCSY experiments. Interproton NOEs were determined by phase-sensitive NOESY. A mixing time ranging from 200 to 600 ms was used for the NOESY experiment.
These data were processed on an Indy computer (Silicon Graphics) equipped with XWINNMR software update. All experiments were performed at 295 K (except for temperature-dependent experiments) and water signal was suppressed by using Noesy Pr1D. All chemical shifts were referenced to a 4.45 ppm water peak.
Temperature coefficients
NH proton temperature coefficients were determined over a 285-315 K temperature range. Chemical shift varied linearly with temperature in all protons; temperature coefficients were determined as being the gradient of chemical shift function dependence over temperature (-ΔδHN/ΔT).
Structure calculations
The distance geometry (DGII) program (MSI) was used to generate 50 starting structures. NOE intensities, used as input for the structure calculations, were obtained from a NOESY spectrum recorded at 295 K with 400 ms mixing time. NOEs were partitioned into strong, medium and weak categories and then converted into distance restraints (1.8-2.8, 2.8-3.5 and 3.5-5.0 Å). Pseudo-atoms were used where diastereotopic protons could not be distinguished from each other and the corresponding corrections for restraints involving pseudo-atoms were applied. Hydrogen bond constrains were introduced for the low amide temperature coefficients; only < 3.5 -ΔδHN/ΔT ppb/K were used in structure calculations. Distance ranges involving this likely hydrogen-bound NHi ..Oi-4 were set at 1.8-2.5 Å.
The structures from DGII were refined using restrained simulated annealing (SA) software (Discover). All peptides were forced to trans and Cá chiralitys to L during the calculations.
The final representative family of lowenergy conformers satisfying the set of experimental distance restraints was determined by the energy and root mean square clustering method.
RESULTS AND DISCUSSION
Solubility of peptide 1585-analogue series
Figure 1 shows the solubility data for seven different analogue series. A general overview of the Asp-analogue series (Figure 1A) showed decreasing solubility values regarding the native sequence (peptide 1585). Nevertheless, positions 3 (Leu), 6 (Lys), 9 (Ala), 16 (Lys) and 17 (Lys) were critical. Each of these amino acids was replaced by Asp but there was no correlation between charge, size or polarity and decreasing solubility trend. The serine-analogue series (Figure 1F) showed similar behaviour to the aspartic- analogue series, with some differences in critical position. In this case, 3 out of the 5 critical positions were similar (positions 6, 16, 17), having 2 new critical positions, 7 (Pro) and 15 (Leu).
Asn-analogue series (Figure 1C) showed increasing solubility, except in position 9 (Ala), 17 (Lys) and 18 (Gln), which were the critical positions. Glu and Gln solubility data were similar. There were only two common critical positions in solubility test for all the analogue series: 16 (Lys) and 17 (Lys). The Lysine-analogue series presented unexpected behaviour. It was supposed that substituting a hydrophobic amino acid such as Val or Leu in a peptides sequence would increase its solubility a lot. This increasing solubility value only occurred when valine was replaced (Lys-2 and Lys-11 peptide analogues), but was not so in leucine (Lys-3, -5, -8, -15 and -19 peptide analogues). This unexpected result was observed for leucine replacement in all the analogue series (Aa-3, -5, -8, -15 and -19 peptide analogues).
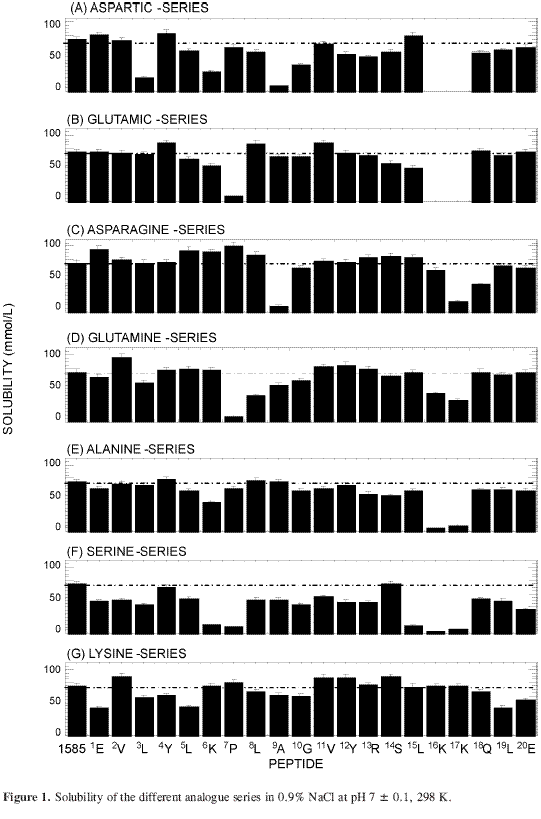
Peptide 1585-analogue series adsorption
The lysine-analogue series (Figure 2G) was designed as a negative control since rejection was expected between aluminium hydroxide and positively charged peptides. There was agreement between expected and found results. Lys substitution in position 6 by any other amino acid in all the analogue series (Figures 2A to 2F) gave an increasing value for the amount of peptide adsorbed onto the aluminium hydroxide. The same behaviour was found for arginine in position 13 (Figures 2A to 2F), but in lesser proportion. Very low solubility was found when lysine was replaced in positions 16 and 17 in the Asp-, Glu-, Ala- and Ser-analogue series, so it was not possible to analyse the adsorption for those analogue peptides (Figures 2A, 2B, 2E, 2F). Almost all the analogues decreased their adsorption respecting reference peptide 1585, except in position 6 where lysine was replaced by Asp, Glu, Ser, Asn, Gln, or Ala.
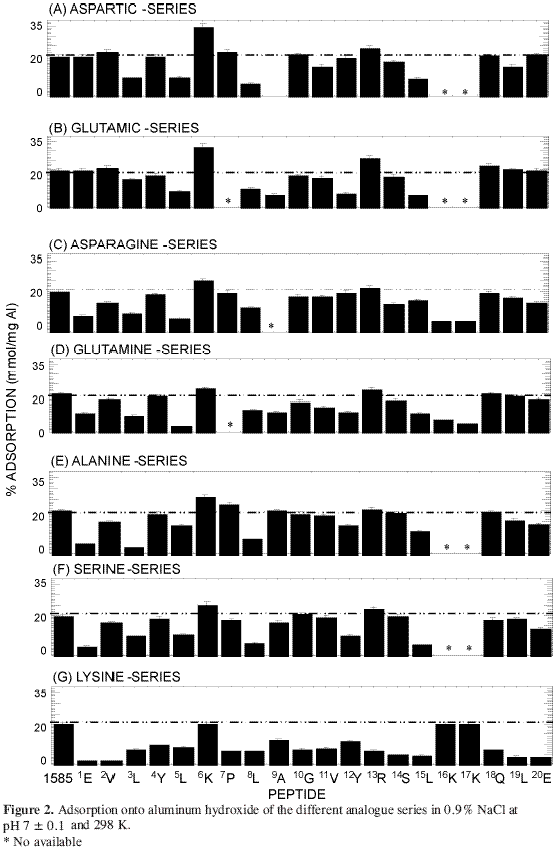
Irregular analogue peptide behaviour was observed in all series, the fact that positive Lys charge in position 6 was relevant in the adsorption process onto aluminium and that adsorption increased when Lys serially replaced the amino acids in this series sequence indicated that these peptides adsorption onto aluminium hydroxide was predominantly guided by electrostatic effects.
Larger adsorption was shown in all analogues peptides series where Lys (6) and Arg (13) had been replaced. The largest increase in adsorption was shown with the Asp-6 analogue (Lys in position 6 was replaced by Asp) peptide.
In view of the physicochemical similarity, having the same charge and a light structural difference, it can be expected that aspartic and glutamic amino acids affect in similar way the behavior of peptides. Nevertheless, glutamic acid gives analogue peptides with smaller adsorption capacity than the corresponding aspartic acid analogues. It can be explained because of the larger mass and volume of glutamic acid which generates a decrease in load density with regard to that of aspartic acid, in order to compensate for the revolting effect of the positively loaded residues. Furthermore, changes in chemical nature of adsorption site and in analogue structure should be considered which can influence an appropriate orientation of the molecule toward the adsorbent surface. Position 3 analogues, where the adsorption increase, had the opposite effect possibly because of glutamic acid compensates part of the lysine load in position 6, as already was established, is determinant for the adsorption on aluminium hydroxide.
The Asp-6 analogue peptide (having better adsorption) and the Asp-5 analogue (having very low adsorption) were chosen as positive and negative controls for NMR structural analysis.
NMR studies
The 1H chemical shifts were assigned according to Wüthrichs standard strategy (14). TOCSY and DQF-COSY spectra were used for correlating side-chain spin system with HN-CHα cross-peaks. The amino acids were sequence-specific linked by using CHα-HN, CHα-HN or NH-HN space connectivity obtained from NOESY spectra.
Structure determination
Medium-strength signals were found between spatial neighbours, such as dNN(i, i+1), dαN(i, i+3), dαN(i, i+4), dαβ(i, i+3) (Figure 3), suggesting the presence of a region having a helical tendency between residues 5L and 19L for Asp-5 analogue peptide and between 6K and 19L for Asp-6 analogue peptide.
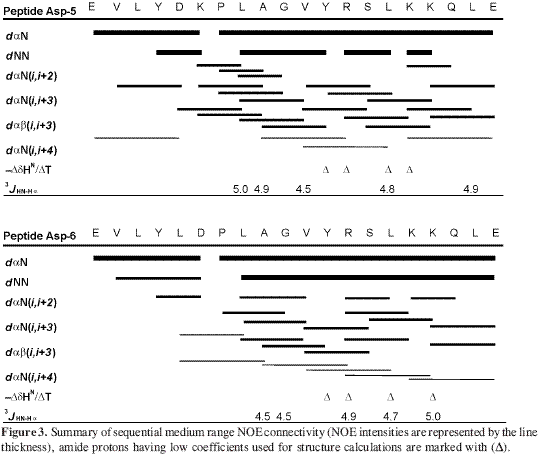
Amide proton temperature coefficients less than 4.5 ppb/K presented extremely slow exchange with solvent hydrogen and were possibly involved in hydrogen bonds. Asp-5 analogue peptide presented coefficients of less than 4.5 ppb/K in residues 12Y, 13R, 15L and 16K and Asp-6 analogue peptide in 12Y, 13R, 15L and 17K residues (Figure 3). This confirmed that the peptide had adopted an á-helix structure.
Table 2 shows that 26 out of the 50 resulting structures chosen for Asp-5 analogue peptide did not present distance violations greater than 0.35 Å or ω angles greater than 1.70°. An average RMSD for main-chain atoms equal to 0.27 Å was obtained by superimposing the structures between amino acids 5L and 19L resulting in a consensus structure having the least total energy. 24 out of the 50 resulting structures for Asp-6 analogue peptide were chosen which did not present distance violations greater than 0.29 Å or ω angles greater than 1.20°. An RMSD value equal to 0.24 Å was obtained by superimposing the structures between amino acids 6K and 19L. Kabasch and Sanders analysis of these peptides showed an α-helix structure between residues 5L and 19Lfor Asp-5 analogue peptide and between residues 6K and 19Lfor Asp-6 analogue peptide (Figure 4). The amino terminal regions were flexible in both peptides.

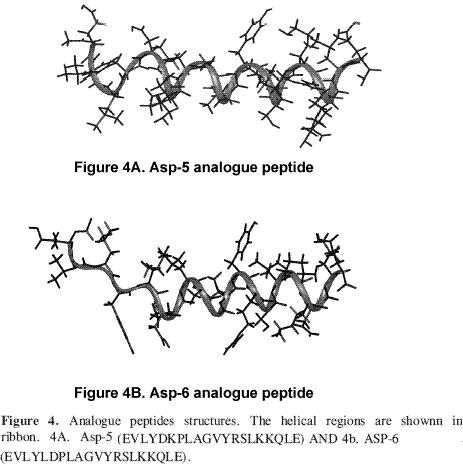
In summary, the unusual up and down values in solubility and adsorption data suggested that there were no regular tendency in the analogue series. No correlations between solubility and adsorption data were found. NMR analysis revealed differences in the -helical structure extension between these analogues. In Asp-5 analogue peptide the -helical structure extends from residue 5 to 19 and in Asp-6 analogue peptide from residue 6 to 19. In analogue Asp-5 (showing very low adsorption), the Aspartic acid is located in the hydrophobic face of the helix, while in analogue Asp-6 (showing maximum adsorption) the Aspartic acid is in the hydrophilic side. The fact that the Aspartic acid in position 6 is located in the polar side of the helix, may allow this analogue to fit better onto the adsorption regions. The fact that positive Lys charge in position 6 was relevant in the adsorption process onto aluminium hydroxide and the decreasing adsorption values in the lysine series indicated that these peptides adsorption onto aluminium hydroxide was predominantly guided by electrostatic effects.
ACKNOWLEDGMENTS
We would like to thank the Colombian Presidents Office and the Ministry of Social Protection for their financial support and Gladys Cifuentes forRMNspectres.
REFERENCES
1. Serna, C. J.; White, J. L.; Hem, S. L. Anion-aluminium hydroxide gel interactions. Soil Sci. Soc. Am. J. 1997; 41:1009-1013. [ Links ]
2. Shirodkar, S.; Hutchinson, R. L.; Perry, D. L.; White, J. L.; Hem, S. L. Aluminium compounds used as adjuvants in vaccines. Pharm. Res. 1990; 2:1282-1288. [ Links ]
3. Seeber, S. J.; White, J. L.; Hem, S. L. Predicting the adsorption of proteins by aluminium-containing adjuvants. Vaccine 1991; 9:201-203. [ Links ]
4. Al-Shakhshir, R. H.; Lee, A.; White, J. L.; Hem, S. L. Interaction in model vaccines composed of mixtures of aluminium- containing adjuvants. J. Colloid Interface Sci. 1995; 169:197-199. [ Links ]
5. Al-Shakhshir, R. H.; Regnier, F. E.; White, J. L.; Hem, S. L. Contribution of electrostatic and hydrophobic interactions to the adsorption of proteins by aluminium-containing adjuvants. Vaccine 1995; 13: 41-44. [ Links ]
6. Rinella, J. V.; White, J. L.; Hem, S. L. Effect of anions on model aluminium adjuvant containing vaccines. J. Colloid Interface Sci. 1995; 172:121-130. [ Links ]
7. Serna, C. J.; White, J. L.; Hem, S. L. Factors affecting homogeneous precipitation of aluminium hydroxide gel. J. Pharm. Sci. 1978; 67(8):1179-1181. [ Links ]
8. Matheis, W.; Zott, A.; Schwanig, M. The role of the adsorption process for production and control combined adsorbed vaccines. Vaccine 2002; 20:67-73. [ Links ]
9. Chang, M.; White, J. L.; Nail, S. L.; Hem, S. L. Role of the electrostatic attractive force in the adsorption of proteins by aluminium hydroxide adjuvant. PDA J. Pharm. Sci. Tech. 1997; 51(1):25-29. [ Links ]
10. Espejo, B. F.; Cubillos, M.; Salazar, L. M.; Guzmán, F.; Urquiza, M.; Ocampo, M.; Silva, Y.; Rodríguez, R.; Lyoi, E.; Patarroyo, M. E. Structure, Immunogenicity, and Protectivity relationship for the 1585 malarial peptide and its substitution analogues. Angew. Chem. Int. Ed. 2001; 40:4654-4657. [ Links ]
11. Merrifield, R. B. Solid phase peptide synthesis I. The synthesis of a tetrapeptide. J. Am. Chem. Soc. 1963; 85:2149-2154. [ Links ]
12. Smith, P. K.; Krohn, R.; Hermanson, A.; Mallia, K.; Gartner, F.; Provenzano, M.; Fujimoto, E.; Goeke, N.; Olson, B.; Klenk, D. Measurement of protein using Bicinchoninic acid. Anal. Biochem. 1985; 150:76-85. [ Links ]
13. World Health Organizn, mmuno - logical adjuvants, World Health Organization Technical Report Series: Geneva 1976; (595) 6-8. [ Links ]
14. Wüthrich, K., NMR of proteins and nucleic acids: New York John Wiley and Sons 1986. [ Links ]