Introduction
Preserving and protecting natural resources, such as water, is one of the nowadays main environmental concerns. The water effluents are polluted with high concentrations of heavy metals, pesticides, herbicides, synthetic dyes, soaps, salts, pharmaceuticals, and personal care products 1.
Synthetic dyes are aromatic compounds with structural diversity, high chemical stability, and resistance to microbial attack. Approximately 8 x 105 t/year of synthetic dyes are produced worldwide, with more than 100,000 dyes commercially available, which are extensively used in many industries, including the textile, cosmetic, pharmaceutical, and food ones. Thus, these industries produce large amounts of wastewater with high concentrations of synthetic dyes 2.
Large quantities of drinking water (150 L kg-1 of textile material) are consumed in the textile industry, due to inefficiencies in the dyeing process. These textile effluents polluted with synthetic dyes (concentrations between 10-15% w/v) are discharged into rivers 3. Contamination of water sources with dyes interferes with the photosynthetic processes of aquatic organisms and impacting the health of the population that receive contaminated water 4. Also, there are several studies related to synthetic dyes with cases of toxicity, carcinogenesis, mutagenesis, and teratogenesis in humans 5.
Azo dyes are the most common leachates in textile factories, accounting for 70% of their aqueous wastes 4. Due to its high chemical stability and resistance, Reactive Black 5 (RB5) constitutes 50% of the azo dyes used in the textile industry. However, these characteristics make it a toxic compound, recalcitrant to natural aquifers 6. In this context, it is necessary to develop studies that examine methods for transforming synthetic dyes, such as RB5, into less toxic compounds.
The chemical nature of synthetic dyes confers them resistance to degradation by conventional methods, including sedimentation, adsorption, oxidation with sodium hypochlorite, filtration, coagulation, flocculation, and biodegradation 7. Therefore, advanced oxidation processes (AOPs) have emerged as an alternative for the treatment of waters polluted with synthetic dyes and other substances. Within AOPs, heterogeneous photocatalysis with TiO2/UV is highlighted, due to its non-selective action, high oxidant power, and its efficiency for treating complex mixtures of contaminants 8,9. Additionally, it provides the possibility of using solar radiation as a primary source of energy. This character of sustainability gives significant environmental value 4.
In photocatalytic processes, others semiconductors such as zinc oxide (ZnO), stannic oxide (SnO2), zinc sulfide (ZnS), and cadmium sulfide (CdS) can be used as catalyst for the degradation of contaminants 10. However, TiO2 is the most photoactive semiconductor employed (300 nm < λ < 390 nm) 8, due to its high activity.
The mechanism of AOPs is based on the formation of transient species with high oxidizing power, mainly hydroxyl radicals (•OH, potential redox 2.8 V), which have a very high effect on the organic matter oxidation and are ideal for recalcitrant compounds treatment, i.e., synthetic dyes from industrial wastewater 11. Heterogeneous photocatalytic methodologies are based on the band model. For a semiconductor catalyst, the absorption of a photon with an energy amount (hv), equal or greater than its gap band energy, produces excitation of the electrons from the valence band (VB) into the conduction band (CB), leaving "holes" in the VB. The photo-generated electrons and positive holes cause the reduction and oxidation of the organic matter 9,12 , 13.
It has been reported that 1 kg of dye is enough to pollute a volume of water of 100 L or higher, that is a concentration of 10000 mg L-114. However, high substrate concentrations decrease the photonic efficiency and saturate the TiO2 surface, which leads to catalyst deactivation and a decrease of the degradation efficiency 15
,
16. The presumed reason is that at high dye concentrations the generation of •OH radicals on the surface of catalyst is reduced, since the active sites are covered by dye ions. Another possible cause for such results is the UV-screening effect of the dye itself. At a high dye concentration, a significant amount of UV radiation may be absorbed by the dye molecules, rather than the TiO2 particles, and that reduces the efficiency of the catalytic reaction because of the concentrations of •OH and decrease 16. In order to assess the effect of the dye concentration, several of the previous works that have been executed with AOPs report dyes concentrations between 10 mg L-1 and 200 mg L-117
,
18.
The objective of the present work was to develop a photocatalytic methodology with TiO2, for the remediation of aqueous solutions contaminated with RB5 (concentrations of 50 mg L-1, 75 mg L-1, and 100 mg L-1). This presents a contribution to the ongoing discussions on dye decolorization. An exhaustive statistical analysis to obtain suitable operating conditions to carry out the photocatalytic degradation of the dye, on a laboratory scale, was done. The objective of our research was not to identify the by-products of the reaction, but the evaluation of its cytotoxicity; this parameter indicates the degree of toxicity of the by-products. Besides, the cytotoxicity analysis has not carried out in most of the studies on this topic, but this type of analysis is important to determine the environmental impact of the treated water.
Materials and Methods
Chemicals
Reactive Black 5 (RB5, 85% w/w from DyStar, Germany), was donated by Fabricato-Tejicondor S.A., from the city of Medellín, Colombia. Titanium dioxide (TiO2, 91.5% w/w), NaOH, 100% w/w and HCl, 37% v/v were purchased from Merck, USA. Dulbecco's modified Eagle's medium (DMEM, reference D5030, reagent grade), dimethyl sulfoxide (DMSO, reactive grade), 3-(4,5-dimethylthiazol-2-yl)-2,5-diphenyltetrazolium bromide) (MTT, reactive grade) and doxorubicin (98% w/v), were obtained from Sigma-Aldrich, USA. Fetal bovine serum (FBS, reagent grade); Trypsin- EDTA (ethylenediaminetetraacetic acid, reagent grade, catalogue R001100), were provided from Invitrogen, USA.
Cell line
HepG2 cell line (ATCC HB-8065), was purchased from the ATCC (American Type Cell Collection, USA) cell bank.
RB5 decolorization kinetics by photocatalysis with TiO 2 /UV
A Box-Behnken experimental design was selected in the Statgraphics Centurion XVI® statistical program to study the effect of the combination of three factors on dye decolorization: TiO2 concentration (0.1, 0.175, and 0.25 g L-1), pH (3, 7, and 11), and RB5 concentration (50, 75, and 100 mg L-1).
Fifteen tests were performed, with three central points (0.175 g L-1 of TiO2, 75 mg L-1 of RB5, and pH 7), and five degrees of freedom for the experimental error (Table 1). Also, two control tests were performed, using two center point conditions: 1) without UV light and with TiO2, and 2), with UV light and TiO2 free. When fitting the model, residuals plot was checked, in searching of potential outliers.
The RB5 photocatalytic decolorization was performed in an aluminum photoreactor (R.A. Talleres), equipped with five lamps (Philips 30W, TL-D Actinic BL), with λ = 365 nm. According to the experimental design (Table 1, Figure 1), 250 mL beakers were used, with 100 mL of the dye solution and TiO2. The reaction mixtures were stirred at 100 rpm for 30 min; then, maintaining the stirring at 30 °C, the lamps were turned on for 14 h. The reaction was monitored every 2 h. The RB5 decolorization percentage was measured as the response variable. For the analysis, the reaction mixture was centrifuged at 3200 rpm for 15 min (Heraeus, D-37520 Osterode), and the supernatant was filtered through nitrocellulose filters (φ of 0.45 μm).
Degradation kinetics of the dye was determined by plotting the decolorization percentage of RB5 against the time of the photocatalytic reaction. As a part of the analysis of the results, the calculation of the area under the curve (AUC) was also taken into account, as it is a useful methodology when the progress of a process over time is tested. The total area was obtained by summing the area of the trapezoids under the curve, which is formed between pairs of decolorization percentage readings over time 19.
RB5 decolorization in aqueous solution
The RB5 decolorization was followed spectrophotometrically (Shimadzu UV-1800 spectrophotometer) at 598 nm. It was expressed in terms of decolorization percentage (D%) (Equation [1]) 20:
Where A 0 corresponds to the initial absorbance and A t to the absorbance after the photocatalytic reaction.
Optimization of RB5 photocatalytic decolorization
Because the concentration of TiO2 is a parameter that significantly affects the decolorization rate of the dye, it is essential to optimize it. TiO2 particles are likely to aggregate at high concentrations, which reduces the transmission of UV light, and hence the speed of reaction 8.
To optimize the photocatalytic decolorization of RB5, the results obtained after applying the Box-Behnken experimental design were analyzed using the Statgraphics Centurion XVI® statistical program. It was determined that the decolorization process could be improved using 0.25 g L-1 of TiO2, a pH of 3, and 50 mg L-1 of RB5. However, to determine the influence of the TiO2 concentration on the dye decolorization, two concentration values of TiO2 were applied: 0.25 g L-1 and 0.5 g L-1, keeping a fixed pH of 3, and a concentration of 50 mg L-1 of dye. Thereby, six additional trials (independent to the experimental design) were proposed, being stricter concerning to TiO2 concentration (see tests 16-21, in Table 2).
Cytotoxicity analysis
The MTT reduction method was employed to establish the cytotoxicity of the reaction mixtures corresponding to the tests with the highest percentage of RB5 decolorization, utilizing the HepG2 cell line 21. The cells were seeded in 96-well culture dishes, using DMEM medium with 10% FBS.
After 24 h, the mixtures from the photocatalytic reaction (100% w/v), were added to the culture dishes at serial dilutions of 50, 25, 12.5, 6.25, 3.13, and 1.56% w/v. The samples were incubated for 72 h at 37 ° C and 5% CO2. After this period, the MTT was added to the culture dishes, and after additional 3 h of incubation at the same temperature, DMSO was added. Finally, the absorbance at 570 nm was determined and the minimum lethal concentration (LC50) was found. The cells were observed trough an inverted microscope (Nikon ECLIPSE TS 100). The assays were run twice, in independent experiments, with two replicates per dilution. Doxorubicin was used as positive cytotoxicity control, as it is a cytotoxic agent intercalating the DNA; untreated cells were used as negative cytotoxicity control 22.
Results and Discussion
RB5 decolorization kinetics by photocatalysis with TiO 2 /UV
The effect of TiO2, pH and RB5 on decolorization percentage was evaluated, using a Box-Behnken design. Under the conditions of the 10th (0.175 g L-1 TiO2, 50 mg L-1 RB5, and pH 3), 13th (0.25 g L-1 TiO2, 75 mg L-1 of RB5, and pH 3), and 15th (0.25 g L-1 of TiO2, 50 mg L-1 of RB5, and pH 7) tests, 100%, 99.40%, and 99.6% of the RB5 were removed after 14 h, respectively (Figure 2. For best interpretation, see Table 3).
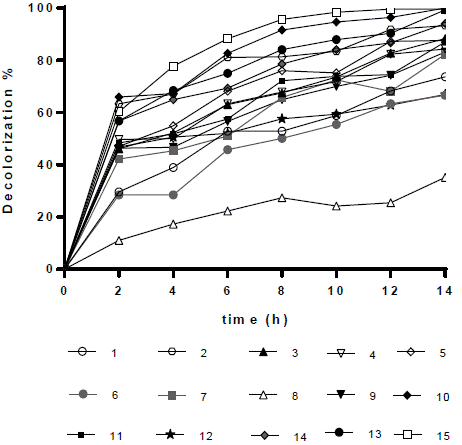
Figure 2 RB5 Decolorization in aqueous solution for 14 h. Results obtained after applying the Box-Behnken experimental design (Table 1).
Table 3 Decolorization percentage of RB5 for 14 h. Results obtained after applying the Box-Behnken experimental design.
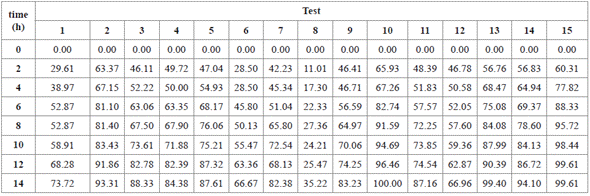
Particularly, for the 15th test, the degradation was almost complete (98.44%) after 10 h; hence, the process could be completed in this period with a representative removal percentage. Also, it was observed that under such conditions (15th test), the highest dye removal rate was achieved following first-order kinetics (R2 = 0.9819, ln C vs t, Figure 3), with a rate constant of k = 0.4223 h-1 (Equation [2]).
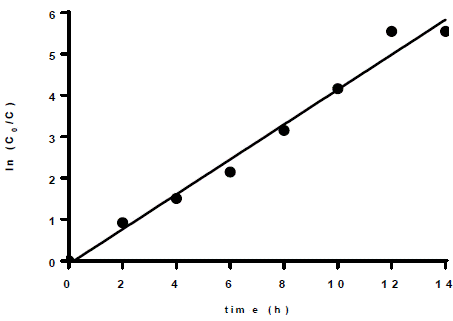
Figure 3 First-order kinetics for RB5 decolorization, under the conditions of test 15 (0.25 g L-1 of TiO2, 50 mg L-1 of RB5, and pH 7).
Where C o corresponds to the RB5 initial concentration and C relates to the RB5 concentration at time t.
The results obtained with the experimental data are consistent with those previously reported for different azoic dyes, such as Remazol Red RR, Remazol Yellow RR, Procion Crimson H-exl, and Procion Yellow H-ex, for which removal percentages > 90% were achieved 4, 23. Additionally, other authors have reported that the degradation of azoic dyes and other substances by heterogeneous photocatalysis, using TiO2 and UV, light can be followed by a first-order kinetics process 19 , 24. Furthermore, it has been reported previously that the degradation of reactive orange 16 (an azo dye like RB5), by heterogeneous photocatalysis with UV/TiO2 follows first-order kinetics 25.
Since the kinetics were evaluated only by the RB5 concentration, it is necessary to take into account that the evaluation of other involved species may be necessary, if the overall kinetic parameters are required 19. These species (e.g., intermediates) might include •OH, H2O2, and , as indicated by the following oxidative-reductive reactions (Eqs. [3]-[9]) 12
,
26:
In general, RB5 degradation was favored with low contaminant loads (50 mg L-1, see tests 2, 10, 14, and 15). However, removal percentages higher than 67% were achieved for an RB5 concentration of 100 mg L-1 (trials 6, 7, 9, and 11, Figure 2). These results were excellent compared to those reported by Puentes-Cárdenas et al.27, who achieved removal of 17% of dye when treated 80 mg L-1 of RB5 by photocatalysis with TiO2 particles, deposited on borosilicate. However, they also indicated that at low RB5 concentrations (10, 50, and 70 mg L-1), the decolorization was > 80% 27. Furthermore, it was observed that the degradation of 50 mg L-1 of RB5 using 0.25 g L-1 TiO2 and UV light was effective for the dye degradation, indicating that under such conditions the surface of the catalyst was not saturated and therefore TiO2 was active in the photocatalytic process.
Also, TiO2 can be reutilized up to four times and still degrades the RB5 (> 90%), which demonstrates its stability. This characteristic of TiO2 would allow treating several loads of RB5 using the same catalyst, and therefore a system of continuous degradation could be implemented.
Due to the fact that pH can affect the adsorption of dye on the catalyst surface, the point of zero charge (pzc) of the TiO2 must be taken into account; the pzc corresponds to a pH value of 6.9 12. In acid (pH < 6.9) or alkaline (pH > 6.9) media, the surface of the TiO2 can be protonated (positively charged) or deprotonated (negatively charged) (Eqs. [10] and [11], respectively). Besides, the RB5 in aqueous solution is negatively charged because it is a sulphonated dye 28.
Since the In this study, photocatalytic degradation is favored at pH 3. Under this condition RB5 degradation is faster because TiO2 particles are positively charged, so RB5 is adsorbed easier on TiO2 as a result of the electrostatic attraction of the positively charged photocatalyst with the dye 29. This behavior agrees with what has been reported by other authors who claim that the degradation of azo dyes increases with decreasing pH 13 , 16. At low pH, reduction by electrons in CB may play an important role in the degradation of dyes due to the reductive cleavage of azo bonds16.
Promising removal of the dye was also found at pH 7 (15th test), which is good considering that actual polluted effluents (e.g., from textile industries) have a high pH. Although, as reported Threrujirapapong et al.29, the diverse composition of synthetic dyes in the wastewater is an important reason for the different results.
Fitted model for AUC
A second-order response surface model for AUC on TiO2, pH and RB5 was fitted, using data shown in Table 4. All main effects and the interaction TiO2*pH were statistically significant (p-value < 0.05), as shown in Table 5.
Table 4 Area under the curve (AUC) of Concentration vs Time, for the RB5 decolorization after 14 h, by photocatalysis with TiO2/UV
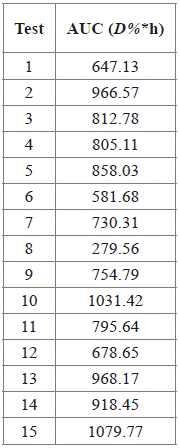
Table 5 Estimated parameters of the second-order response surface model for AUC on TiO2, pH and RB5, and their corresponding p-values.
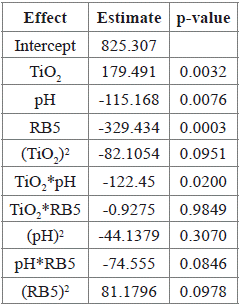
Using a numerical method implemented in Statgraphics, a maximum expected AUC of 1168.50 D%*h was found, which is attainable at TiO2: 0.3 g L-1, pH: 3.0, and RB5: 50 mg L-1. This is consistent with the results of the 10th and the 15th test (AUC: 1031.42 D%*h and 1079.77 D%*h, respectively), whose conditions were close to those for which the maximum AUC is predicted. The Figure 4 shows the evaluated region for pH and TiO2, with RB5 fixed at 50 mg L-1.
For the two test controls (with TiO2 and without UV light, and with UV light but TiO2 free), there were no changes, as the dye oxidation is mainly mediated by the action of the TiO2 semiconductor in the presence of UV light, which activates the catalyst. In addition, a low decolorization percentage (about 7%), was found in the absence of UV light. This result could be associated with the adsorption of the dye on the solid TiO2 surface, as reported by Chong et al.4.
According to the optimization tests, under the conditions of the 21st test (0.5 g L-1 of TiO2, pH 3, and 50 mg L-1 of dye), the highest decolorization percentage (99.51%) was reached in 10 h. The six trials (assays 16 to 21) resulted in decolorization percentages > 94% at 10 h. From the above, it is possible to conclude that the catalyst load used in the optimization tests is not a determining factor in the improvement of the process productivity (Figures 5 and 6).
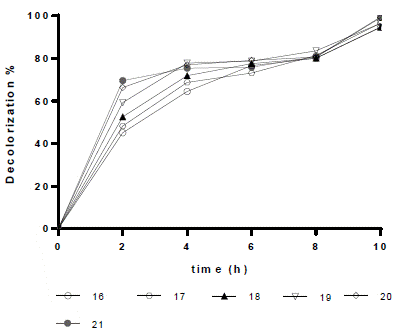
Figure 6 Optimization test: RB5 decolorization percentage in aqueous solution vs. time (for 10 h). Results obtained for six assays: 16 to 21 (Table 2).
With the optimization tests, the time required to achieve a decolorization percentage over 94% was reduced to 10 h (Figure 6). This behavior was also observed with the 10th and 15th tests (Figure 2). Besides, it was confirmed that the RB5 decolorization by photocatalysis with TiO2/UV was favored at an initial pH of 3. The decolorization percentages reached in the optimization tests did not differ significantly from each other. However, to reduce costs and to facilitate the catalyst separation, low concentrations of TiO2 (0.25 g L-1 - 0.35 g L-1) should be used. Further, it was found that at low dye concentrations (50 mg L-1), the degradation process is faster, similar to the findings reported by Puentes-Cárdenas et al.27, who obtained decolorization percentages higher than 80% in 10 h, when using dye concentrations of 10 mg L-1, 50 mg L-1, and 75 mg L-1.
Conversely, Chong et al.4 achieved 97% decolorization with a RB5 concentration of1 mg L-1 after 150 min oftreatment with a TiO2 concentration of 0.1 g L-1. In contrast, in the present work, under the conditions of the 16th test (0.25 g L-1 of TiO2, 50 mg L-1 of dye), a decolorization percentage of 94.6% was reached at 600 min (Figure 6). This result is due to the higher proportions of dye and catalyst that were used in this study, compared to those used by Chong et al.4. Besides, there have been previously reported lower times compared to those obtained for the RB5 degradation. Though, as mentioned above it is important to note that the concentrations of dye used in the present study (50 mg L-1) are higher than those reported by other authors; therefore, it would take more time to remove dyes (14 h). However, longer treatment times than those used in this work have also been reported in the literature, for instance, Threrujirapapong et al.29 reported a retention time of 2 days in a photocatalytic reactor for sufficient wastewater treatment.
Cytotoxicity analysis of RB5 degradation products
The cytotoxicity test was done in the 10th and 21st trials, as those had the best decolorization percentages. The evaluated samples did not show toxicity in the HepG2 cell line. All samples showed a lethal concentration of 50 (LC50) > 25% w/v, which indicates that concentrations of the reaction mixture higher than 25% w/v are required to cause the death of 50% of the HepG2 cells (Table 6).
Table 6 LC50 values in HepG2 cells, obtained for RB5 photocatalytic decolorization mixtures (tests 10 and 21), compared to doxorubicin (positive control).
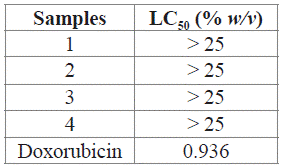
Table 6 shows the results obtained for the samples and the positive control (doxorubicin). It is important to note that LC50 was the same for all of the samples, i.e. LC50 had the same value for those samples corresponding to t = 0, as for those corresponding to t = 14 h, and t = 10 h, respectively. It is concluded that the photocatalytic process presented in this work do not generate cytotoxic by-products.
The biological evaluation is presented in Figure 7. The growth of the cells submitted to the different treatments was similar to that of the untreated control cells, i.e., the fact that the cells were in the presence of the resulting mixture of the photocatalytic process, did not affect their growth. Hence, the applied photocatalytic treatment is a viable alternative for the removal of azo dyes, since it makes it possible to achieve high decolorization percentages of RB5, with no formation of toxic products.
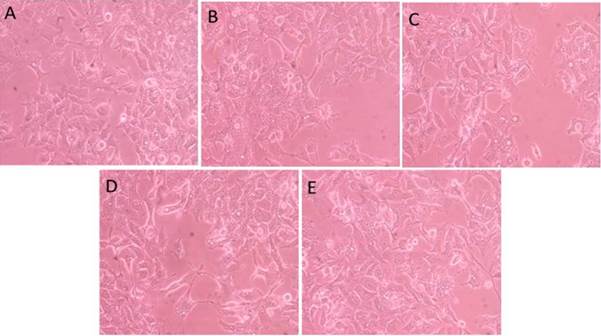
Figure 7 Biological evaluation of the HepG2 cell line. A: Sample 1 (10th test before photocatalysis, i.e. RB5 in solution, t = 0), B: Sample 2 (10th test after photo-catalysis, t = 14 h), C: Sample 3 (21st test before photocatalysis, i.e. RB5 in solution, t = 0), D: Sample 4 (21st test, after photocatalysis, t = 10 h), E: Control cells without treatment. 20x magnification, inverted microscope.
Similarly, Ferraz et al.30 evaluated the cytotoxicity of the final products of the degradation of different azoic dyes after the application of a photo-electrochemical treatment with TiO2 as a catalyst. In their study, they verified that after the process, the reaction mixture did not induce DNA damage or chromosomal mutation in the HepG2 cell line, with cell viability higher than 90%.
Conclusions
Photocatalysis with TiO2 and UV light can decolorize water stained with RB5. In particular, RB5 was removed by 99.61% after 14 h of treatment (0.25 g L-1 of TiO2, 50 mg L-1 of RB5, and pH 7). Additionally, after 10 h of reaction, a decolorization percentage of 94.66% (0.25 g L-1 of TiO2, 50 mg L-1 of RB5 and pH 3), was achieved. Thus, photocatalytic degradation of RB5 is faster in low initial dye concentration, reaching a maximum in acidic conditions at 10 h. Based on the results obtained in the optimization, it is possible to conclude that the catalyst loading is not a determinant of the decolorization process. Thus, it may be preferable to use low amounts of it to reduce operating costs. The applied photocatalytic treatment is a viable alternative for the removal of azo dyes; since it makes it possible to achieve high decolorization percentages of RB5, without formation of toxic products. The reaction mixtures evaluated were non-toxic to HepG2 cells, i.e., they do not reduce cell line viability.