INTRODUCTION
Functional groups (FGs) are groups of species with similar morphology and physiology that also respond similarly to a set of environmental conditions, without the individuals necessarily belonging to the same phylogenetic group (Reynolds et al., 2002). This model constitutes an efficient tool that better predicts the effect of environmental changes on the organisation of the phytoplankton community than taxonomy-based studies (Kruk and Segura, 2012; Sutherland et al., 2017).To predict changes in the environmental gradients of aquatic ecosystems, FGs was proposed by Margalef (1978) as an adaptation response of lifeforms to stability and nutrient content. Reynolds (2002) proposed a classification based on morphological and physiological characteristics, thus establishing 31 FGs, which were later updated by Padisák et al. (2009). Kruk et al. (2010) proposed a new classification of FGs based on morphology (MBFG), and divided the phytoplankton community into seven groups on the basis of morphological characteristics, such as volume and the presence of a flagellum or silica exoskeleton. Morphological characteristics are related to the functional properties of phytoplankton (Kruk et al., 2010). For example, the size and shape of phytoplankton cells affect growth, metabolism and resource acquisition (Litchman and Klausmeier, 2008).
Although FGs have been studied in natural environments, research has not yet been conducted on FGs in landfill treatment under American tropical conditions. Landfill leachate is a complex wastewater generated by the percolation of rainwater through waste and by the different biochemical reactions that occur within the waste mass of the landfill. Generally, landfill leachate contains biodegradable and non-biodegradable organic matter, nutrients, heavy metals and recalcitrant and xenobiotic compounds (Renou et al., 2008; Madera et al., 2015). Different technological alternatives have been used for landfill leachate treatment. However, little research has been conducted on the use of natural systems, particularly those that employ algal biomass.
Algal ponds are referred to as 'high-rate systems' based on improved biomass yield or productivity and their capacity to treat wastewater (García et al., 2000; Valigore, 2011). High-rate algal ponds (HRAPs) facilitate the treatment of wastewater through the high production of algal biomass and dissolved oxygen by means of a high photosynthetic rate. Thus, they provide a favourable aerobic environment for bacteria to degrade organic matter (Pagand et al., 2000; Park and Craggs, 2010; Sardi-Saavedra etal., 2016). In addition, nutrients, organic matter and suspended solids diminish as a result of the combination of physical and chemical and biological processes, such as sedimentation, precipitation and microbial transformation (Kumar and Zao, 2011).
Millán et al. (2014) analysed FGs on the basis of the functional characteristics of phytoplankton communities associated with two pools intended for the treatment of fish-farm wastewater. A total of 11 Reynold's FGs groups were identified, of which F and P were the most representative. The researchers also noted that the number of FGs decreased at the outlet of the treatment systems, where S1, W1, and H1 were conserved, because they were characteristic of eutrophic environments. Li et al. (2015) found 22 FGs on the basis of functional characteristics in a eutrophic reservoir, where groups S1, D and J predominated. Southerland et al. (2017), suggested that microalgal functional groups helped to understand the ecology of wastewater treatment and biomass production in full-scale HRAP better than individual species did. They found that group J, consisting of mainly non-gelatinous, non-motile Chlorococcales, was the dominant group in the HRAP. However, this type of research is still emerging, and further analysis is needed.
In High-Rate Algal Ponds, algal organisms perform contamination-treatment processes during which organic matter and nutrients are utilised by bacteria, algae and other organisms (Peña et al., 2005). However, the dynamics of the algal community is unknown. Additionally, although Sardi-Saavedra et al. (2016) applied an appropriate ecological approach, a number of areas in this field require clarification ( Peña et al., 2012; Sardi-Saavedra et al., 2016), including the analysis of FGs in the treatment of landfill leachate. Phytoplankton functional groups have not been studied in constructed systems such as a HRAP treated intermediate landfill leachate. In these hypereutrophic systems, phytoplankton community is specific, but its ecological function is not well known, so the application of models of functional groups helps to understand their dynamics. This study will allow understanding the functional ecology of these systems and will provide better information for further best design. In addition, it allows a fast evaluation of the functioning of the systems without the necessity to measure other variables that take time and are costly. Thus, the purpose of this study is to assess for the first time the dynamics of phytoplankton communities in an HRAP used for the bioremediation of landfill leachate through the identification of FGs and the correlation of such groups with the physical and chemical water characteristics.
MATERIALS AND METHODS
Study area
An HRAP was installed within the premises of the Regional Presidente landfill, located in the municipality of San Pedro, Valle del Cauca, Colombia (3°56x01.54" N and 76°26'26.05" W) (Fig. 1). The area has an average temperature of 24.3 °C, a precipitation of 2.3 mm.d-1 and a relative humidity of 74.8 %.
The HRAP was designed with the characteristics shown in Table 1. The system received the effluent of a technological coupling that consisted of a High Rate Anaerobic Pond (HRAP) and a subsurface horizontal flow constructed wetland on a pilot scale that treated leachate (2 m3d-1) from the Regional Presidente landfill. The landfill receives approximately 490 T d-1 of solid waste, of which nearly 77 % corresponded to organic matter. In addition, in the landfill, between 2 and 5 L s-1 of leachate were generated (Bugaseo, 2009).
Physical and chemical characteristics
HRAP sampling and data generation were performed at a depth of 0.05 m from the pond surface. The following physical and chemical parameters in the water samples were measured: phosphates (PO4 -3 -P), total suspended solids (TSS), volatile suspended solids (VSS), dissolved organic carbon (DOC), total chemical oxygen demand (COD) and filtered COD (filtered COD), total Kjeldahl nitrogen (TKN), N-NH4+ (ammoniacal nitrogen) and N-NO3 - (nitrates) in accordance with APHA (2012) and IDEAM (2009). Similarly, data related to temperature, pH, and dissolved oxygen (DO) were recorded in situ using a WTW 340i multi-parameter portable meter. Finally, chlorophyll a was determined using an AquaFluor® Handheld Fluorometer and Turbidimeter.
Biological samples
Algal samples were grabbed fortnightly between January and July 2013 except in March and April because the operating HRAP system was not working. These systems are not controlled by the seasonal regime, and their dynamics is associated to the operation of the treatment systems. Approximately 500 ml samples were collected in plastic containers at the pond's centre. We filtered 500 ml of sample and we homogenized this amount of volume to be able to be count. The samples were fixed with 10 ml formaldehyde (4 %) as a preservative following the recommendations by Zaixso (2002). Relevant organisms were identified using the keys and taxonomic descriptions provided by Tell and Conforti (1986), Wehr and Scheath (2003) and Bicudo and Menezes (2006) up to the lowest possible taxonomic level. Additionally, photos were taken and sent to specialists for taxonomic confirmation.
Phytoplankton biovolume was estimated according to the geometric equations proposed by Hillebrand et al. (1999). For phytoplankton quantification, a Sedgwick-Rafter camera was used, and counting was performed using a Nikon Eclipse TS100 inverted microscope following the methods employed by Villafane and Reid (1995) and Wetzel and Likens (2000). To count phytoplankton, the formula proposed by Wetzel and Likens (2000) was used:
Where:
N: Number of organisms /ml
C: Number of organisms
A: Area of the counting chamber (mm2)
a: Area of the ield or band (mm2)
S: Number of ields or bands counted
V: Volume of the counting chamber (ml)
To classify the phytoplankton organisms into the morphology-based functional groups we followed the method proposed by Kruk et al. (2010).
Data analysis
We considered the physical and chemical parameters established in the Colombian environmental regulations (Resolution No. 0631 of 2015, Ministry of Environment and Sustainable Development) to assess the relationships with the biovolumes of the algal populations. Additionally, we conducted an exploratory data analysis and canonical correlation analysis (CCA) to identify associations between a first set of Y1 independent variables (i.e., physical and chemical variables) and a second set of Y2 variables (i.e., total biovolume of each MFFG in one sample) in the seven month of the study. For the implementation, the tools available in XLSTAT 2018.5 (2018) software were applied.
RESULTS
Water quality
The results for the physical and chemical parameters are summarised in Table 2. Water pH was always alkaline, the values remaining over 8.1 all times along the study period. Additionally, the temperature was suitable for algal growth (between 25° C and 33° C). The pond exhibited aerobic reactor behaviour, reaching an average DO concentration level of 9.1 mg L-1. Chlorophyll a monitoring during the time of the study revealed a stable system, reaching concentrations up to 3659 ug L-1. Nutrients exhibited high levels: PO4 3- up to 5.28 mg L-1, NH4+ up to 131 mg L-1 and NO3 - up to 15 mg L-1. Because the HRAP treated a medium-age leachate, whose main characteristic is a high concentration of nutrients, these conditions were expected (Renou et al., 2008) and obtained in the present study.
Table 2 Physicochemical variables in the HRAP during the study period. Where: DOC (Dissolved organic carbon), PO43- (Phosphates), COD (Total chemical oxygen demand), Filtered COD (chemical oxygen demand in the filtered water), TSS (total suspended solids), VSS (volatile suspended solids), TKN (total Kjeldahl nitrogen), NH4+ (ammoniacal nitrogen), NO3- (nitrates), DO (dissolved oxygen) and T (temperature).
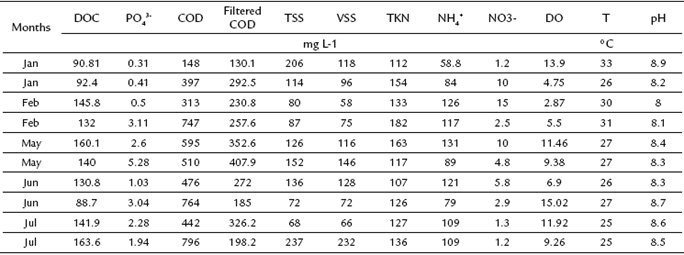
Morphology-based functional groups
Overall, 26 morphospecies were recorded, distributed into six phyla and 20 genera (Table 3). Among the seven MBFGs proposed by Kruk et al. (2010), only four groups (IV, V, IV and VII) were found in the HRAP (Table 3). Of these MBFGs, Group V exhibited the largest number of species (14), followed by Group VI (six), Group IV (five) and VII had one specie. When considering abundance alone, Group V was dominant throughout the study period, accounting for 98 % of the total individuals sampled. In terms of biovolume, Group V exhibited the same dominant pattern (Fig. 2).
When assessing the dynamics of the biovolume of the MBFGs throughout the study period, Group V showed a high fidelity, being present in ten samples. Group V was also the dominant group, its biovolume accounting for more than 65 % in each studied month. In contrast, Group IV was present in five samples and groups VI and VII occurred in four of the samples. Group VII appeared in less than 5 % of all samples, whereas Group VI reached 31 % of the total biovolume for January and 27 % for February (Fig. 3).
Correlation between the MBFG and the physical and chemical water characteristics
The first two factors of the CCA factorial decomposition summarized the variables analysed. They accounted for 82.3 % of the total observed variability (F1 36.3 % and F2 45.8 %). Figure 4 shows the simultaneous representation of a set of Y1 and Y2 variables in the two factorial axes. The two factors, F1 and F2, display a negative relationship with all the variables of the study. The graph also shows that the biovolumes of MBFGs V and VII relate to the periods during which the pond had low levels of DOC. The biovolumes of the MBFG VI related to low levels of fil COD and NH4+. Additionally, the biovolume of IV relate to TKN increases in the pond.
DISCUSSION
The richness of MBFGs recorded in this study was lower than that observed by other authors, such as Petar et al. (2014), Santos et al. (2014) and Mihaljevi et al. (2015). In this regard, Santos et al. (2014) and Mihaljevi et al. (2015) reported seven FGs, whereas Petar et al. (2014) reported five groups. In our study, we reported only four groups (Table 4). This lower MBFG richness may be related with the type of treated water, while the previously mentioned studies were performed in rivers and natural lakes in temperate climates. In the present study, landill leachate was treated under American tropical conditions and thus involved a substantially different between the physical and chemical characteristic of landill leachate compared with natural water bodies.
In our research, the pond was alkaline throughout the entire study period, this situation can be related with the biological activity inside the landill and also indicates high photosynthetic activity. Consequently, high DOC concentrations of were found, which facilitated the continuous growth of important heterotrophic bacteria for the degradation of organic matter (Park and Craggs, 2011). In addition, high phosphate, nitrite and nitrogen levels resulted in an increase in the primary production and potential inhibitions that potentially affected the function, structure and dynamics of the pond's algal community. Similarly, it can contribute to high algal productivity, which could be exploited as animal feed or for biodiesel production once the material is harvested.
The HRAP was dominated by Group V, which consists of phytoflagellate species, many of which exhibit mixotrophic behaviour, acting as heterotrophs or autotrophs depending on the conditions. This behaviour represents a significant advantage with respect to the reduction of the organic matter and nutrients present in leachate. In addition, mixotrophic mobility favours movement towards light when this resource is limited or away from light when this resource is abundant (Litchman and Klausmeier, 2008). However, the growth of these organisms reduces light availability for other species. This phenomenon would explain the abundance of Group V throughout the study and the presence, although in smaller proportion, of Groups IV, VI and VII, which are adapted to survive at low light levels (Litchman and Klausmeier, 2008).
Group V is characterised by species that adopt different strategies. For example, some species of the phylum Cryptophyta are regarded as C-strategists. That is, they are small organisms capable of quickly exploiting the available resources and tolerating environmental changes (Reynolds, 1988). In contrast, the S-strategists Chlamydomonas and Euglenophyta grow in more stable, warmer conditions and proliferate in extremely high concentrations of organic matter, such as wastewater (Padisák et al., 2009). In our study, the prevalence of Group V species coincided with the high contaminant loads present in the leachate. It is possible that these strategic differences reflect different hydrological conditions throughout the study period, which can be regarded as a disadvantage when predicting changes in the environmental conditions.
Although represented by few individuals, the other groups displayed adaptation mechanisms that enabled them to resist the high nutrient concentration in the pond. Group VII individuals had mucilage that acted as a mechanical and chemical defence against predators, and their decreased nutrient absorption acted as a population regulatory mechanism while offering floating properties (Margalef, 1978). This group has a preference for high-temperature lakes with a high trophic status (Kruk and Segura, 2012). Group VI, which consists of diatoms, depends on water turbulence (Litchman and Klausmeier, 2008) and easily adapts to low-light conditions and eutrophic environments (Reynolds et al., 2002). Although Group VI exhibited an ample range of responses to trophic status (Reynolds et al., 2002), its members are better competitors in lower temperature conditions (Kruk and Segura, 2012). In contrast, Group IV would not be dominant but can survive under such conditions of high turbulence, excess nutrients and low-light levels (Kruk and Segura, 2012).
Finally, when correlating biovolumes with the physical and chemical characteristics of the water pond, we found that MBFGs V and VII were associated with the periods during which the pond had low levels of DOC. This correlation indicates that under low contaminant concentration conditions in leachate the HRAP new groups appeared, increasing its capacity to reduce such contaminants. Therefore, it is possible to produce an effluent with a low pollution load, which may entail a better performance of the pond. In addition, the biovolume of Group IV was favoured by an increase in TKN in the pond, possibly implying a better capacity for nitrogen assimilation in this group. Lastly, different analyses revealed no association between the levels of fil COD, NH4+ and DOC and the levels of TKN, which indicates that the MBFGs that are favoured by high levels of these variables may well be present simultaneously.
CONCLUSIONS
The HRAP was inhabited by four phytoplankton MBFGs (IV, V, VI y VII) during the study period. These groups were associated with the physical and chemical characteristics of the landfill leachate. Group V formed by the phytoflagellates, was the group with more fidelity and biovolume in this study. This group plays a fundamental role in this system because it is resistant to high concentrations of organic matter and can thrive in this environment. Therefore this group could generate favourable conditions for the reduction of organic matter and nutrients that contribute to the transformation of the contaminants present in the leachate. On the other hand, the HRAP used to treat medium-aged leachates displayed a high richness of MBFGs, which contributed to the reduction and transformation of pollutants. This MBFG richness was related to and depended on the physical and chemical quality of water. Finally, the MBFG technique is useful for understanding the dynamics of the phytoplankton community in the HRAP and also use as a potential tool for influent water quality.