1. Introduction
The development of thermoplastic matrix compounds with mineral fillers is a common practice in the industry due to the low cost of producing molded products. The incorporation of elements such as calcium carbonate (CaCO3) [1-3], talc [4-6], rice husk ash [7], kaolin and mica [8-11] as well as some organic fillers [12-13] have been shown to improve and modify drastically characteristics of strength, stiffness, durability, hardness as well as its thermal stability [14]. These properties in the polymers with particulate material are linked to their composition and structure, which in turn are influenced by interfacial interactions, which depend on the size of the interface and the strength of the interaction [3]. This explains the incorporation of additives such as coupling agents [15], surface treatments and decrease in the size of the particles of the charges [16,17] to enhance their properties. However, the development of this type of materials becomes a technological challenge to promote industrial applications, since in most cases it is required to ensure optimum dispersion of particles to avoid agglomerates and, in other cases, control the formation of aggregates [18-20] Currently, there is a notable trend towards the production of nanoparticles that, despite having exceptional properties, relegates their use in the industry, particularly the automotive, electrical, electronics and construction, due to the high costs and demand for complex pre-treatments [21]. There are numerous studies on the behavior of different types of fillers, sizes and treatments to favor coupling; however, these factors have few studies during reprocessing cycles that simulate the recycling of PP compounds with mineral fillers of talc and carbonate calcium. The degradation by reprocessing of PP [22] compounds with fillers has been studied by several authors including Elloumi et al. [23] who reported on the effect caused by multiple injections of a polypropylene [PP] of impact on the thermal, rheological, and mechanical properties. The values of percent of crystallinity were directly proportional to the molecular weight, which decreased due to the chain scissions. Young's modulus and tensile strength were kept constant in virgin PP matrices and recycled with the incorporation of CaCO3. The mechanical properties of the nanocomposites were strongly influenced by the intrinsic resistance of the matrix, the concentration, and the dispersion of the filler. Beg and Pickering [24] studied the degradation of PP compounds with wood fibers, they found a gradual decrease in the mechanical performance through repeated cycles of processing of the compounds. Similar results were reported by Bourmaud and Baley [25] who compared the thermal and mechanical properties of PP reinforced with hemp, sisal and glass fibers after reprocessing. Microscopic analyses showed a reduction in the length and in the diameter of the natural fibers, while the glass fibers presented rupture transversally to the fibers only. There was a 40% decrease in the elastic modulus of the compounds during the reprocessing due to the detriment in the nucleation of the fibers, as well as a lower influence of polypropylene-graft-maleic anhydride [PP-g-MA]. Tocháček et al. [26-27] in several studies investigated the effect of multiple extrusion processing on the impact properties of composites of polypropylene. Most of the degradation found by means of the Charpy impact testing corresponded to those presented in the homopolymer phase. The increase in the extrusions cycles revealed a lost in the capacity of energy dissipation in charge of moderating the propagation of the crack in the domains of the copolymer ethylene-propylene [rubber], that in turn were responsible for the cross-linking that manifested as an increase in the average of molecular weight. Similarly, Sarrionandia et al. [28] studied the morphology and mechanical properties of a terpolymer composite of polypropylene/ ethylene-propylene-diene with talc with, after, five injection molding cycles. Reprocessing did not modify the chemical structure nor the thermal behavior of the composite material, but led to a slight reduction in the molecular weight induced by the shear stresses. The size distribution of the terpolymer filler decreased, however the Young's modulus and the impact strength of the composite material did not change significantly, although the deformation at break decreased steadily after five cycles. According to the above statements, and the need to compare high consumption of commercial fillers, the following aims are proposed: First, to study the influence of CaCO3 and talc fillers on a thermoplastic matrix (PP) that has been reprocessed for 6 injection molding cycles. And second, to compare the physicomechanical properties of PP compounds with mineral fillers (talc, CaCO3) reprocessed under the same conditions.
In this paper, rheometry studies obtained during the processing of the composites are presented, as well as the morphological evolution and dispersion of the particles by SEM, the viscoelastic behavior is determined to obtain an indirect molecular weight ratio. Thermal stability and polymeric crystallinity through the TGA and DSC, respectively. Finally, mechanical performance on a macro scale is determined by tensile and bending tests.
2. Experimental methodology
2.1. Materials
Sabic QR6701K polypropylene pellets of random copolymer with a melt flow index of 10 g/10 min, at 230 °C, a load of 2.16 kg, and a density of 0.905 g/cm³. Maleic anhydride grafted polypropylene (from PP-g-MA licocene 7452), with an acid value of ~41 mg KOH/g, a density of 0.93 g/cm3, and softening temperature of ~159 °C. Calcium carbonate (Carcal-75C) with an average particle diameter of 50% of 20.80 μm and talc (impatal-45) with residue values in 45 micron (0.5% max) sieve and 38 mesh sieve μm (0.70% max) produced by IMPADOC S.A.
The methodology of the process is summarized in Fig. 1 and the successive stages are detailed below.
2.2. Injection, grinding and extrusion cycles
The PP was injection molded six times with an 150 ton injection molding machine DEMAG model 1991. The temperature profile was 200 °C - 210 °C - 220 °C and 230 °C for the nozzle. The injection pressure was kept constant at 75.6, the mold temperature was set at 45 °C, and a constant injection rate of 45 cm3.s-1 was applied. The injection parameters were the same for each cycle. The specimens obtained by injection were type 1b "bone" according to the 527-2 ISO standards. Then, the specimens were ground to granules with a diameter of about 8 mm in a Rotrogram Mold-tek blade mill at 1745 rpm. In order to obtain composite materials, the components were mixed in a Thermo Scientific Haake Rheomex PTW OS double-screw extruder with a co-rotating configuration under the following operating conditions: temperature profile with gradual increase of 5 °C from 165 °C in the first feed zone up to 210 °C at the end of the screws and nozzle. For this, the barrel of the extruder was divided into 10 zones.
The samples were labelled as follows: PPnMn, where PP indicates the matrix of polypropylene used and M the mineral charge incorporated (C: calcium carbonate, or T: talc), n subscript corresponds to the number of reprocessing cycle. For example, PP3C is a polypropylene composite obtained in the third reprocessing (PP3) and mixed with calcium carbonate (step 3, Fig. 1); PPC3 is a composite of virgin PP mixed with calcium carbonate that has been reprocessed thrice, three folds, three times (step 3b, Fig. 1). To all the compounds were added 1.5% by weight of PP-g-MA.
2.3. Characterization
2.3.1. Rheological analysis
Rheometry tests were performed in a Thermo Scientific equipment HAAKE Rheomix mixer at different temperatures using roller type rotors. The calculation of the weight of the fillers was made with the following equation (1):
Where w𝑐 is the weight of the compound, V n is the net volume of the mixing chamber, ρ c is the density of the compound, f d is the filling factor and x m is the mass fraction of the component.
2.3.1.1. MFI and complex viscosity
The melt flow index was determined for each PP generation of the particulate material using the ATLAS MFI Extrusion Plastometer at 230 °C, 2.16 kg for four times. The viscosity was determined by a rotational rheometer (DHR-2, TA Instruments) with controlled stress and parallel plate configuration using the equilibrium flow test. The rheological measurements were performed at 190 °C and the shear rate was in the range of 0.001 to 300 s-1. The dynamo-mechanical tests settings were: the percentage of deformation between 1 and 10%, with a frequency range of 0.1 to 628.10 rad/s, to determine the storage modulus and the loss modulus.
2.3.2. Thermogravimetric analysis (TGA) and differential scanning calorimetry (DSC)
Thermal properties were determined in a TGA/DSC 2 STAR System thermogravimetric analyzer, Mettler Toledo. Samples (10 ± 0.5 mg) were placed in alumina crucibles at a temperature range from 25 to 550 °C under a nitrogen atmosphere (50 cm3/min). The experiments were conducted according to E1131-98 ASTM and D3418-12 ASTM standards, respectively. The degree of crystallinity (Xc) was determined eq. (2):
Where f p is mass fraction of the PP in the composites, ΔH m is the melting enthalpy of the sample and ΔH° m is the melting enthalpy of a 100% crystalline isotactic standard of PP (207 J/ g) [29]. In this work, the melting enthalpy (ΔH m) was estimated from the area (A) and the heating rate (dT/dt) eq. (3):
The area corresponds to the calculation of the integral of the heat flow (dt(T)/dt)) along the melting interval (T 0 →T f ), as follows eq. (4):
Also, the normalized melt enthalpy of weight is reported.
2.3.3. Mechanical properties
2.3.3.1. Tensile and flexural strength
Measurements of tensile mechanical properties were performed in a GOODBRAND universal mechanical testing machine in accordance with D638 ASTM standard using a test speed of 50 mm/min, and a 500 kgf cell. The values of elongation at break and tensile strength were determined. A DIES environmental chamber, a Baker king caliper, and an OAKTON thermohygrometer were used. The flexural tests were performed in an INSTRON 5500R universal testing machine in accordance with ASTM D790-10 standard using a test speed of 5 mm/min, and a 50 kgf cell. The modules, strengths and percent elongation were obtained. Five specimens were analyzed for each PP generation and the average values were calculated.
2.3.4. Microstructural analysis
The surfaces of the fractures were examined, and the micrographs were digitally captured using a scanning electron microscope JEOL, JCM 50000. A voltage of 10 kV was applied. Prior to the tests, both specimens were sputter coated with a layer of gold. Magnifications of 600x and 99x of the fracture surface were taken.
2.3.5. Determination of the color index
The samples were analyzed with a Minolta CR-400 colorimeter (D65, 2°, Y = 89.5, x = 0.3176, y = 0.3434) in order to know the surface color. Data were collected in the CIELab and values of L* (brightness), a* (ranging from red to green) and b* (ranging from yellow to blue) were recorded during each triplicate test. The color (C*) was calculated as follows eq. (5):
While the color deviation was calculated using the following equation (6) (ISO 11664-4: 2008/CIE S 014-4/E: 2007) [30]:
It should be mentioned that all the samples were conditioned in an environmental chamber at 25 °C and a relative humidity of 50±5% for 48 h.
3. Results and discussion
3.1. Rheometry
To determine the reological behavior of the mixtures in the torque rheometer, the mass of the components in the mixtures (PPT and PPC) was calculated. The net free volume of the chamber corresponds to the difference between the total volume and the volume occupied by the rotors. In addition, the value for the filling factor (70%) was kept constant; the values are presented in Table 1.
The density for each mixture (PPT and PPC) corresponds to the addition of the individual densities by the fraction of the components, according to the rule of mixtures; these results are presented in Table 2 and 3. In addition, the result of the mass determined from equation 1, Section 2.3.1.
Once the components of the composite were added to the mixing chamber of the torque rheometer, it was operated at a rotor speed of 45 rpm and temperatures of 200 °C, 230 °C and 250 °C for 5 minutes. The behavior of the blends during the processing are shown in Fig. 2.
In general, for all the samples, at around the first quarter of a minute, an increase in torque is observed in the rheogram because part of the PP has not been melted; this also leads to a momentary reduction in temperature. The values in the maximum torque for PPT samples were lower than the torque of the PPC composites except at 250 °C where it decreases to lower values, reaching 5.96 Nm. Afterwards, there is a stabilization of the torque, because all the PP has been melted. According to the rheometry results, it can be inferred that the optimum time to obtain the mixtures is around the first minute, and since the stabilization does not generate significant differences at temperatures between 230 °C and 250 °C, this minimal is established as a parameter of the transformation processes used.
3.2. Melt flow index and viscosity
The melt flow index in the polypropylene (PPn) generations showed a proportional increase due to the type of degradation (chain scission) that occurs in polypropylene as a reprocessing effect [22]. Fig. 3 illustrates the comparative results for the samples developed. The compounds with calcium carbonate and talc from recovered material (PPnT and PPnC) have a disadvantage compared to the reprocessed compounds (PPTn and PPCn), because the firsts maintain the value of melt index close to the virgin polypropylene and its homologues. This shows the fillers limitation to recover the polymer through nucleation, while the reprocessing of the polymer matrix with the mineral filler preserves the flow characteristics.
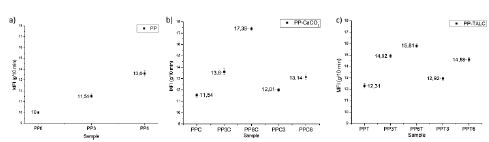
Source: The authors.
Figure 3 Results of flow index of the generations of a) PP, b) PP- CaCO3 and c) PP-Talc.
The typical shear behavior for the complex viscosity observed in PP resins and their compounds is showed in Fig. 4 (a). Compositions of up to 20% by weight of the added fillers tend to produce increase in viscosity; talc content fillers show significant viscosity increases attributed to moistening of the filler (polymer/charge interaction). In general, the compounds showed a significant and similar drop in viscosity at high frequencies (100-1000 s-1). So, they do not represent a problem for their processing. An important aspect to be observed from Fig. 4 (b) on an enlarged scale is that the compounds become more dependent on shear rate in the low frequency range because of the type of filler and the thermal history of the matrix. This shear thinning behavior can be attributed to a higher degree of polymer-filler interaction, which requires higher shear stresses and longer relaxation times for the composite to flow.
3.3. Thermal properties
The results of the thermogravimetric analysis of the starting materials and the reprocessed products used to evaluate the addition of talc and CaCO3 into the polymer matrices are shown in Fig. 5. The values of the degradation temperatures (Fig. 5a) are in all cases superior to the temperatures of the process used for the development of composite materials which gradually decrease from 314 °C to 291 °C for PP and PP6, respectively. In addition, melting temperatures and enthalpies were determined by differential scanning calorimetry analysis (Fig. 5b). Melting temperature values around 154 °C are attributed to crystals found in β-phase, while values around 167 °C are attributed to the fusion of crystals in α-phase. According to the above, generation 3 is believed to be favored by a higher concentration of crystals in the α-phase. Transitions around 125 °C due to PE crystals were identified. Mineral fillers are not discussed because the decomposition temperatures are higher than 800 °C.
The degradation and melting temperatures for the talc and CaCO3 composites presented in Table 4 show the positive influence of the filler on the different generations. The significant differences according to the type of conformation of the material with which it is evidenced that the reprocessing of the composite material encourages the interaction of the material incorporated in the matrix probably by the increase of the surface area that is achieved with the decrease of particle size. In general, the curves obtained in TGA for the compounds show a similar slope from which a gradual relationship between the T10, T50 and Tcut off values are obtained. The melting temperatures were maintained at values close to 152 °C, and the degree of crystallinity of the compounds was between 17% minimum for PPT6 and 24% maximum for PP3T. The fillers used did not promote the change between the crystalline phases of the PPn.
3.4. Mechanical properties
The maximum tensile strengths of reprocessed polypropylene products shown in Table 5 did not exhibit significant changes in their values. These results remain constant within the experimental error and indicate that there is no degradation effect on the mechanical properties of the polypropylene polymer matrix because of the six reprocessing cycles.
The above results allow to establish comparisons between the mechanical properties of the composites developed in the present work; these values are presented in Table 6. The addition of mineral charges such as talc and CaCO3 in the matrix leads to improvements in tensile strength, as they promote rigidity. However, the plastic zone of these materials exhibits an adverse effect on ductility because they drastically decrease the elongation response, although it considers higher bursting stresses. In PPT and PPC, the elongation in the traction test was maintained with respect to PP, which can be attributed to the formation of fillers charge aggregates that are constituted by particles that remain attached with forces higher than those presented by the agglomerates. The aggregates are easily separated from the matrix and tend to reduce the strength of the composite material while increasing its elongation. In contrast to the compounds of CaCO3, a better elasticity/ plasticity ratio is found in the composites with matrices that have a higher number of process cycles longer shear rates there by infers that fillers achieve greater dispersion.
Table 7 shows the results obtained from the flexural test. The talc compounds with recycled matrices have an excellent relationship between the flexural stress and the flexural modulus compared to PP; this result is consistent with the previous inference which indicated greater dispersion of particles. The composites with CaCO3 have lower values, but the effect with recycled matrices continues to mark the trend. The reprocessed talc products (PPTn) are not favored in this property, although the values remain constant between generations 3 and 6.
3.5. Microstructural analysis
The morphology of the fracture surface of the prepared CaCO3 and talc composites was studied; the SEM images for the PPMn series are presented in Fig. 6. The statistical report of particle length distribution is shown in the supplementary information (Table S1) the particles were measured through Image J software, for which a total of 50 randomly chosen particles in a micrograph were taken as the sample to measure the sizes and calculate an average diameter. The reprocessed compounds of PPT3 and PPT6 showed a flat surface fracture, typical characteristic of brittle composites. For this case, the matrix absorbs few energies through the cracks of the compounds [31]. Particles sizes were observed between 0.43 and 21.56 μm for PPT3, whereas for PPT6 particles up to 89.01 μm were found as well as nanoparticles (0.011 µm). The formation of agglomerates responds to the loss of the effect of coupling agent and dispersant (PP-g-MA) by reprocessing. Failing this, PPT samples showed and ductility, the SEM images showed a good distribution of the matrix particles and filaments in the deformation. Similarly, the PPC, PPC3 and PPC6 composites had a greater interfacial resistance with the increase of the generations, since they showed an evident gap between the particles of CaCO3 and the matrix. The particle size decreased to 0.846 μm as a result of reprocessing.
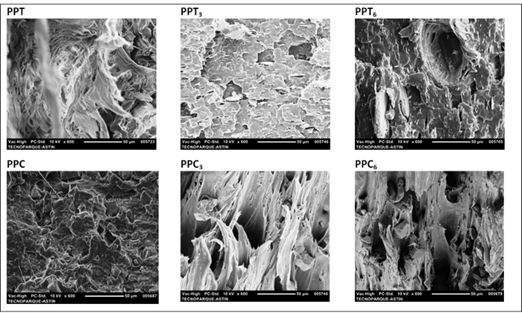
Source: The authors.
Figure 6 SEM micrographs taken from fracture surfaces of PPMn composites at a magnification of 600x.
As shown in Fig. 7, the addition of mineral fillers to recycled PP matrices with a higher melt flow index led to an increase in the distribution and dispersion of the particles. This result is in agreement with the one reported by Wang [32]. However, a limited plastic deformation of the PPnM composites compounds is observed due to the thermal history of the starting material. The measured lengths of de particles were similar for PPT, PP3T and PP6T; these varied between 8 and 20 μm, showing a positive incidence of the co-rotating extrusion process used in the preparation of the composites. Micrographs of compounds PP3C and PP6C showed low particle-matrix interaction, and regular distribution compared to composites with talc.
3.6. Determination of color index
The color analysis carried out in the polymer samples allowed to quantitatively establishing the influence of reprocessing in the PP matrix and the composites that incorporate different fillers on that involves thermal degradation [33,34]. Table 8 presents the results of luminance (L*), color saturation index (C*) and color deviation (ΔE*), the latter being calculated regarding of the homologous series (PP, PPT and PPC). From the above, it is observed that the sample PP6 compared to PP passes from a transparent white color to an opaque yellow color. The increase in the value of b* reflects the saturation gain of yellow, as well as the decrease of the brilliance determined by L*. Also, the CaCO3 composites in the recycled matrix (PP6C) continues to show drastic changes in the L* and b* parameters. This important evidence shows that the addition of 20% fillers by weight does not mask the yellowing of the matrix. The reprocessed compounds PPT6 and PPC6 showed a significant reduction in this indicator. This result coincides with the report of thermal stability for the said composites. Finally, it should be noted that the fillers generate a change of color that is perceptible to the human eye, which undoubtedly entails restricting its application in sectors where transparency is required.
4. Conclusions
The quantification of the effect of thermomechanical degradation induced by the successive reprocessing cycles represents an advance in the development of materials, mainly in the chemistry and processing of the polymers. The reprocessing of PP by injection and the composites with 20% by weight of inorganic material micro-particulate of talc and CaCO3 were studied; the preparation of these materials was performed in twin screw extruder equipment which has proven to be highly effective for dispersing the fillers at high concentrations. Also, the rheological behavior showed a tendency to increase the viscosity of the matrices recycled with the addition of the fillers, generating advantages in the physical-mechanical properties measured. Besides maintaining the processibility conditions of the composites, since the addition of these charges did not lead to an increase in the processing temperature, indicated according to the values obtained for Tm. A slightly higher tensile strength was found in the developed composites compared to the PP matrix, being the mechanical performance of the non-recycled composite materials (PPC and PPT) the highest, as they showed an elongation at break comparable to PP. The morphological behavior of the compounds PPC6 and PPT6 is mainly influenced by the presence of agglomerates, due to the loss of PP-g-MA effect (1.5% added). In contrast, the compounds PP6C and PP6T achieved excellent dispersion of the particles because of the low viscosity of the recycled matrix; however, the nature of PP impinges on the low consistency of fibrillation which decreases ductility. The reprocessing also contributed to the production of nanoparticles (0.011 µm for PPT3), which could be evidenced in the micrographs; these nanoparticles are responsible for enhancing physical and chemical properties in the series of composite materials (PPMn). In this way, the thermal analysis indicated that the fillers stabilize the system. This fact was able to support and correlate with the colorimetric variation. Color determination is a semi-quantitative parameter of the progress in the thermal degradation of reprocessed samples, which can be established as a quality control technique of the product. Finally, it was possible to determine the effect of recyclability on the morphological, thermal, rheological and mechanical properties of PP with talc and CaCO3. The natures of different reprocessed compounds allow extending the perspective of the recycling of materials with polypropylene matrix widely used in the construction and automotive industry.