1. Introduction
Constructed wetlands (CW) have been used in the treatment of domestic, industrial, agricultural, leachate, hospital, stormwater effluents, among others [1-3]; they are effective in wastewater treatment with low construction and operation costs compared to conventional systems due to their low energy and labor requirements, being a good treatment alternative [4,5]. CWs work with the interaction of macrophyte vegetation, microorganisms and support media, the latter can be clay or broken stone among other materials, although it is recommended to use stone materials from the region where it is intended to be installed [6].The support media within the CW are colonized by large microbial populations, which, when feeding, remove suspended solids, dissolved solids, nutrients, inorganic compounds, putrescible and synthetic organic compounds from the wastewater [7,8].
Interest in CWs has increased in recent decades because they have a rich diversity of design and operational characteristics that can be customized for the treatment of various effluents, using various types of wetlands such as free-flow (FFCW), subsurface flow horizontal (SSFCW), vertical flow (VFCW) or various types of species (Phragmites australis, Cyperus difformis, Dracaena sanderiana, Cyperus papyrus, Echinodorus cordifolius, Ludwigia adscendes, Typha augustifolia, Typha latifolia), focused on contaminant removal [9-11]. Plants play an important structural and functional role, oxygenating the environment for microorganisms, removing pollutants and nutrients, providing food and habitats for organisms, among others, but the kinetics of degradation remain largely unknown for each species with the type of CW used [12]. Phytoremediation is the use of plants and trees to clean water and counter- mined soils, it is a passive technique where plants are grown and harvested in a soil or water to remove contaminants (metals, pesticides, solvents, explosives, crude oil, polyaromatic hydrocarbons, leachate and water contaminants) using solar energy and some mechanical cleanup methods [13,14].
In Peru, the contaminant removal efficiency of 2 artificial wetlands operated with Shoenoplectus was evaluated. californicus (cattails) and Nasturtion officinale (watercress) in effluents from a pig farm in Huancavelica, having chemical oxygen demand (COD) and dissolved oxygen (DO) as response variables. Obtaining a removal of 78.88% for the wetland operated with totora and 78.91% for the one operated with pigtails. [15]. In Canada, Typha species were studied. sp. and Lemna sp., in the removal of total phosphorus (TP) and soluble reactive phosphorus (SRP) in a FFCW (for 5 years) that treated wastewater from the dairy industry (milk washing water and liquid manure), concluding that in the wetland negatively influences the maximum extraordinary flow and the removal of TP and SRP was 53.7 and 52.7% respectively, with prolonged HRT high rates of nutrients can be removed [16].
In Karnataka, India, Pennisetum species pedicellatum and Cyperus rotundus were evaluated in SSFCW and FVCW on a pilot scale, as a tertiary treatment with TRH for 12 h and 24 h, reporting that the average removal efficiencies for SSFCW and FVCW were BOD, 77 and 83%; COD, 60 and 65%; NH4+ -N as ammonium, 67 and 84.47%; NO3 - N as ammonium, 69 and 66.75%; and PO4 - P as phosphorus, 85 and 90%, respectively, with VFCW being superior by 7.14% [17].
In Tabasco, Mexico, pilot-scale CWs fed with domestic wastewater operated with different species (Thypa latifolia, Paspalum paniculatum, E. crassipes and C. articulatus) and type of wetland (FFCW and SSFCW), observing that each species has its own phytoremediation capacity [18] so it is important to evaluate/estimate the potential of Sagittaria latifolia for use in wastewater treatment. In southeastern Mexico, there is information available on the abundance and distribution of species that are established in natural wetlands and that can be used for wastewater treatment, particularly in the Pantanos de Centla Biosphere Reserve [19]. Sagittaria latifolia in particular has few studies in wastewater treatment and has been evaluated in SSFCW as tertiary treatment [20], in nutrient removal [21], and in FFCW as treatment. secondary [22] for this reason the possibility of applying and installing EF units for families in decentralized communities such as the particular situation of Centla, Tabasco, which lacks this vital service, pouring wastewater into bodies of water without any treatment. By implementing Sagittaria latifolia, which is a small plant that is easy to handle and transport, construction, operation and maintenance costs can be reduced in family units. Due to the above, in this article Sagittaria latifolia was evaluated in the removal of basic contaminants from domestic wastewater (pH, temperature, total dissolved solids, color, turbidity, dissolved oxygen and chemical oxygen demand), comparing its efficacy and adaptation in FFCW and SSFCW at pilot scale.
2. Materials and methods
2.1. Selection, collection and planting of the species
The species was extracted from natural wetlands of the Pantanos de Centla Biosphere Reserve (18° 18.952' north latitude and 92° 32.376' west longitude). Forty young specimens of Sagittaria latifolia (Swallowtail) were collected for each wetland, three FFCW and three SSFCW installed at the Universidad Juárez Autónoma de Tabasco, Academic Division of Biological Sciences (DACBiol-UJAT) (17° 59.466' north latitude and 92° 58.438' west longitude). Care was taken not to spoil the species during transfer and planting under the recommendations of Crites and Tchobanouglus [23] and CONAGUA [24]. Of the 40 specimens collected, only 15 were planted in each HA (the largest and in good health), being placed in five rows with three plants. A total mass of plants (average ± SD) of 24.3 ± 1.6 kg was placed in the HA, the 90 plants of the study presented stem height of 114.5 ± 0.8 cm, leaf width 20.67 ± 2.3 cm, leaf length 23.10 ± 8.4 cm and humidity of 61±5%. The specimens were placed inside the HA for their adaptation for 25 days, supplying them with domestic residual water from DACBiol-UJAT, observing survival and adaptation to the new conditions that were exposed by monitoring pH, temperature, TDS, Color, Turbidity and COD. The stabilization phase lasted approximately three months, until the species began to reproduce. Dead plants and litter that end their life cycle are removed from the CW to avoid the release of removed contaminants, these removed plants are renewed by new ones that are born within the CW, leaves that are damaged are also removed, avoiding the spread of pests
2.2. CW operation
The CW of the experiment were constructed under the criteria of López et al. [25], they are 10-gauge carbon steel, with dimensions of 1.2 m wide, 2.5 m long, 1 m high, with a treatment capacity of 200 L day-1, the exterior and interior are covered by anticorrosive alkyd enamel. and inside it is protected with elastomeric waterproofing, with five layers of textile fiber. All of them are equipped with PVC hydraulic pipe (0.05 m), to distribute the flow of residual water. The FFCW operated with a 0.1 m gravel tie and the SSFCW with 0.5 m, which works as a support for the plants. The mixed gravel (crushed round pebble river rock) had a diameter of 19.5±6.8 mm, a density of 1480±60 kg m-3 and a porosity (n) of 0.53±0.01. The source of residual water comes from restrooms and cafeterias of the DACBIOL-UJAT. The wastewater flow was supplied from the sump-cistern (pretreatment unit that removes larger sedimentary and suspended solids) to the two 0.2 m3 distribution tanks with two 1 HP centrifugal pumps, through pipes, ball valves and adapters (pin, threaded joints, elbows, T joints) of 1” PVC hydraulic type. The tanks had the function of distributing the residual water to the HA, being filled three times a day (8, 14 and 24 hours). The pipe was verified and corrected for a passage of water in laminar flow, verifying that the feed corresponded to 0.14 L min-1, where the Reynolds number was ≤ 2300 [26].
2.3. Capacity and sampling
The gauging of the treated wastewater was carried out after one year of operation for four weeks from Monday to Friday as they are days where there is an organic load from the source (students) (from August 20 to September 14, 2018), measuring four times a day, at 8, 12, 16 and 20 hours., the method used was direct Volume-Time gauging [26] The water quality sampling was carried out from Monday to Friday at 11:00 a.m., under the guidelines of NMX-AA-003 Wastewater-Sampling. A simple sample was taken from each sampling point, that is, in the distribution tanks (RT1 and RT2), in the effluent of three FFCW-SL with Sagittaria latifolia , in the effluent of three SSFCW-SL with Sagittaria latifolia and its respective targets (three FFCW-W without species and three SSFCW-W without species), collecting a total of 14 daily simple samples for four weeks, with a total of 280 samples that were analyzed at the DACBiol-UJAT Water Technology Laboratory, preserving them according to the rules.
2.4. Analytical determination
Determination of control parameters such as temperature (°C), turbidity (NTU), color (UC), dissolved oxygen (DO, mg L -1), potential hydrogen (pH), total dissolved solids (TDS, mg L -1) and chemical oxygen demand (COD; mg L -1), was by methods SM 2550, SM 2130 B, APHA 2120, SM 4500 OG, SM 9040 B, SM 2540 and USEPA 410.4 respectively. To obtain the temperature, TDS and pH, the multiparameter HANNA Waterproof was used. Tester model HI 98129, a HANNA HI 98193 was used for DO, a LaMotte SMART3 photometer for color, a HANNA HI 98703 turbidimeter for turbidity and a HI 83099 photometer for COD.
2.5. Experimental design and statistical analysis
In this research, we only sought to see the performance of the plant in the wetland and an experimental design of one factor and two levels was proposed to analyze the treatment systems (type of flow with species) and their controls (type of flow without species), evaluating the variables temperature, turbidity, color, pH, TDS, DO and COD, of which all the response variables turned out to be non-parametric data because they did not comply with the postulates of normality and homoscedasticity , since the standardized bias and/or the standardized kurtosis was outside the range of -2 to +2 for the Treatments in the analyzed variables. This indicates some significant non-normality in the data, which violates the assumption that the data come from normal distributions, so nonparametric analysis was used to find statistical differences using Kruskal-Wallis and Mann-Whitney test of medians. The data was analyzed with the STATGRAPHICS version 16.1 software. Three repetitions were performed for each treatment (Fig. 1).
2.6. Removal efficiency
The removal efficiency of basic contaminants in wastewater treatment was evaluated by measuring the response parameters at the inlet and outlet of each experimental unit, according to the following eq. (1):
Where: ɳ = removal in percentage, Ce = average concentration of wastewater inlet, Cs = average concentration of treated wastewater outlet.
2.7. Estimation of the kinetic degradation constant “k”
The behavior of residual water obeys a first order kinetic reaction [23]. A piston flow will be considered for both types of managed wetlands, since according to Rodríguez-Miranda et. al., [27] FFCW can behave better as a plug flow reactor in terms of organic matter removal according to the HRT that is used, similar to the one that is to be used in this research; eq. (2):
Where:
t: retention time for COD removal (d),
C e: COD concentration in the reactor effluent (mg/L),
C 0: Concentration in the influent
k: Degradation constant (days -1).
3. Results
In the FFCW, the operating cost during business days was 199.5±2.1 L day-1 (mean ± standard deviation), with a minimum value of 195.8 L day -1 and a maximum of 203.8 L day -1. For the SSFCW, the operating cost was 201.1±2.1 L day-1, presenting a minimum value of 197.3 L day -1 and a maximum of 204.5 L day -1, so the HRT for the FFCW (0.1 m of support) was 6.7 days and for the SSFCW (0.5 m of support) it was 3.5 days. For the weekend (Saturdays and Sundays) spending tends to zero. Next, Fig. 2 shows the behavior of spending during the week for both types of CW.
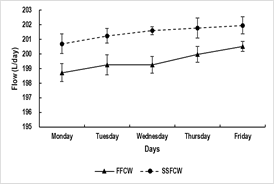
Source: Self-made
Figure 2 Average operating expense (X) ± standard error (SE) in FFCW free-flow constructed wetlands and CWFS flow-constructed wetlands (N=20).
Table 1 Average values ± SD of the experimental units: raw wastewater, CW with species and without species.
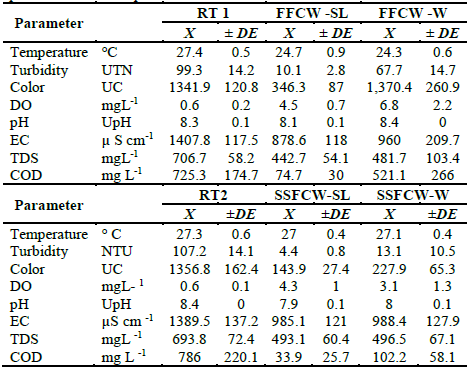
Source: Self-made
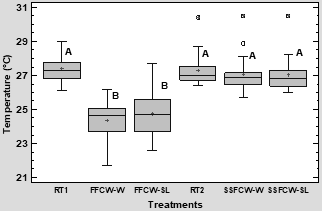
Source: Self-made
Figure 3 Median values ± interquartile range for temperature (°C). Different letters indicate significant differences (Kruskal-Wallis; p<0.05) with a 95% confidence statistically significant between treatments.
The physicochemical characterization obtained in the evaluated treatments and their controls (RT1: control tank 1 raw residual water, FFCW-W: free-flow wetland with witness; FFCW-SL: free-flow artificial wetland with Sagittaria latifolia ; RT2: control 2 raw wastewater, SSFCW-W: subsurface flow wetland with white; SSFCW-SL: subsurface artificial wetland with Sagittaria latifolia ), was compared among all in order to verify the concentrations of influent-effluent and which treatments presented greater removal of contaminants.
The general characteristic of the residual water that supplied the experiments presented a temperature of 27.3±0.8 °C, turbidity of 103.3±27.1 NTU, color of 1,349.3± 225.2 UC, DO of 0.6±0.3 mg L -1, pH of 8.3±0.2, TDS of 699.5±85.9 mg L -1 and COD of 755.7±198.5 mg L -1. Table 1 shows the values per control tank and effluents of the SSFCW and FFCW with their respective blanks.
The results of the statistical analysis of the experiment show us that in temperature a significant statistical difference was found between the medians (p<0.05). FFCW-W treatment presented the lowest median value with 24.65 °C, followed by FFCW-SL with 24.7 °C, while the highest value was RT2 with 27.0 °C (Fig. 3).
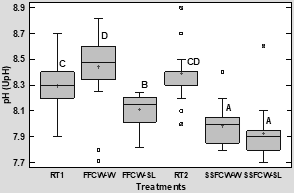
Source: Self-made
Figure 4 Median values ± interquartile range for pH (UpH). Different letters indicate significant differences (Kruskal-Wallis; p<0.05) with a 95% confidence statistically significant between treatments.
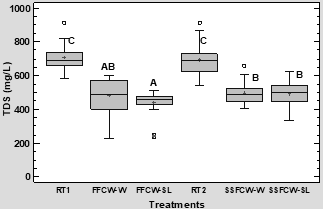
Source: Self-made
Figure 5 Median values ± interquartile range for total dissolved solids (mg L -1). Different letters indicate significant differences (Kruskal-Wallis; p<0.05) with a 95% confidence statistically significant between treatments.
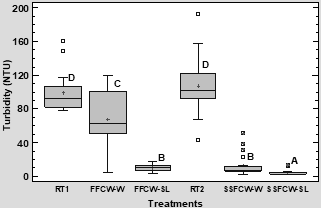
Source: Self-made
Figure 6 Median values ± interquartile range for turbidity (UNT). Different letters indicate significant differences (Kruskal-Wallis; p<0.05) with a 95% confidence statistically significant between treatments.
In pH (p<0.05), the SSFCW-SL treatment presented the lowest median value with 7.9, followed by SSFCW-W with 8, while the highest value is the FFCW-W treatment with a pH of 8.48 (Fig. 4).
In the TDS (p<0.05), the FFCW-SL treatment presented the lowest median value with 460.0 mg L -1, followed by the SSFCW-W with a median value of 486.5 mg L -1, while the highest value is RT1 with 690.5 mg L -1 (Fig. 5).
Turbidity and color also showed a statistical difference (p<0.05). The SSFCW-SL treatment presented the lowest median value of turbidity with 3.15 NTU, followed by SSFCW-W with 7.35 NTU, while the highest value is the RT2 treatment with 102.0 NTU (Fig. 6).
The CWFS-SL treatment presented the lowest median value of color with 116.0 UC, followed by CWFS-B with 196.0 UC, while the highest value is the RT2 treatment with 1345.5 UC (Fig. 7).
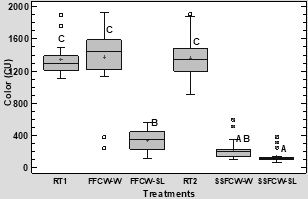
Source: Self-made
Figure 7 Median values ± interquartile range for color (UC). Different letters indicate significant differences (Kruskal-Wallis; p<0.05) with a 95% confidence statistically significant between treatments.
The OD (p<0.05), revealed that the FFCW-W treatment presented the highest median value with 6.55 mg L -1, followed by the SSFCW-SL with 4.2 mg L -1, while the lowest value is RT2 and RT1 with 0.6 mg L -1 (Fig. 8).
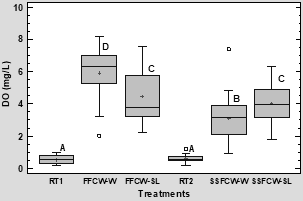
Source: Self-made
Figure 8 Median values ± interquartile range for dissolved oxygen (mg L -1). Different letters indicate significant differences (Kruskal-Wallis; p<0.05) with a 95% confidence statistically significant between treatments.
Finally, for the COD parameter (p<0.05) it was found that the lowest median value was in the SSFCW-SL treatment with 24.5 mg L -1 , followed by the FFCW-SL treatment with 72.5 mg L -1 , with the SSFCW treatment -W with 82.0 mg L -1 . The treatment with the highest value was RT2 with 769 mg L -1 (Fig. 9).
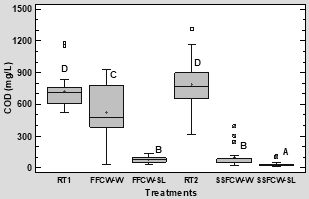
Source: Self-made
Figure 9 Median values ± interquartile range for chemical oxygen demand (mg L -1). Different letters indicate significant differences (Kruskal-Wallis; p<0.05) with a 95% confidence statistically significant between treatments.
Table 2 Removal efficiency in free-flow constructed wetlands (FFCW) and subsurface flow constructed wetlands (SSFCW) with and without Sagittaria latifolia. Average values (N=20).
Parameter | RE (%) FFCW-SL | RE (%) FFCW-W | RE (%) SSFCW-SL | RE (%) SSFCW-W |
---|---|---|---|---|
T. Water | 9.6 | 11.1 | 1.1 | 1 |
Turbidity | 89.8 | 31.8 | 95.9 | 87.8 |
Color | 74.2 | -2.1 | 89.4 | 83.2 |
OD | -704.1 | -1123.4 | -595.2 | -403.5 |
pH | 2.2 | -1.8 | 5.3 | 4.7 |
SDT | 37.4 | 31.8 | 28.9 | 28.4 |
COD | 89.7 | 28.1 | 95.7 | 87 |
Source: Self-made
Table 3 Removal kinetic constants in free-flowing constructed wetlands (FFCW) and subsurface flow constructed wetlands (SSFCW) with and without Sagittaria latifolia.
Wetland | HRT | Color | Turbidity | COD |
---|---|---|---|---|
days | k, days -1 | k, days -1 | k, days -1 | |
FFCW-SL | 6.7 | 0.2 | 0.3 | 0.3 |
SSFCW-SL | 3.5 | 0.6 | 0.9 | 0.8 |
FFCW-W | 6.7 | -0.0 | 0.0 | 0.0 |
SSFCW-W | 3.5 | 0.5 | 1.4 | 1.4 |
Source: Self-made
The turbidity and color removal efficiency in FFCWs is high in wetlands with species (FFCW-SL) being 89.8 and 74.4% respectively. The FFCW-W reached 31% in turbidity and the color of the effluent increased due to the presence of algae, with a negative efficiency of -2.1%. The removal of turbidity and color in the SSFCW was higher in the wetlands with species (SSFCW-SL), being 95.9 and 89.4%, respectively. The SSFCW-W reached 87.8% in turbidity and 83.2% in color. Regarding COD, higher removal is reported in SSFCW-SL with 95.7%, followed by FFCW-SL with 89.7% and in the case of whites, SSFCW-W reached 87% and FFCW-W only 28.1%. The other parameters evaluated in the CW are presented in Table 2, observing that the removal of contaminants is greatening the SSFCW, except for the OD parameter, which is greater in the FFCW-W, since it was favored by the presence of algae.
3.1. kinetic constants
The removal kinetic constants are found in Table 3, where it can be seen that the best kinetic behavior for the variables color, turbidity and COD occurs in the subsurface flow wetlands, which observe a plug flow behavior
4. Discussion
To evaluate the performance in wastewater treatment systems, the removal efficiency is estimated [6], if the efficiency is reported negative (-), it means that the concentration of the pollutant is higher in the effluent (output ) than in the influent (entrance), which is favorable in the DO, since it means that the macrophytes have supplied oxygen favoring degradation, increasing the concentration of DO in the wetland [28], but in other parameters it represents problems and the phenomenon is known as "short circuit" and involves a lack of control of the process (increasing flow rate, entrainment speed or concentration) and must be corrected so that the concentration in the effluent is lower than the input concentration as foreseen in its design [29]. Wang et al assure that these problems are caused by factors such as the organic load and the porosity of the substrate [45]. The temperature of the residual water of the influent was 27.3±0.8 °C, presenting in the FFCW-SL and FFCW-W 24.7±0.9 and 24.3±0.6 °C, while the SSFCW-SL and FFCW-W presented 27.0±0.4 and 27.1±0.4 °C respectively. These values are ideal for removing soluble and suspended organic matter [23] Similar to the temperature found by Romellon-Cerino et al., in a treatment train worked with the same species (Sagittaria latifolia) [41], since the temperature favorably influences the processes favored by microorganisms such as artificial wetlands [30], the growth of mesophilic organisms is favored, the which have adequate metabolic development at that temperature, performing many biogeochemical functions such as nitrification, denitrification and P assimilation [31,32]. In this way, the temperature complied with the maximum permissible limit (LMP) in its discharge to receiving bodies, which is 40 °C as established in NOM-001-SEMARNAT-2021 [33]
The SSFCW with Sagittaria latifolia presented the lowest values of color and turbidity effluents of up to 143.9±27.4 CU and 4.4±0.8 NTU, while the FFCW with species presented 346±87 CU and 10.1±2.8 NTU. The SSFCW-W by the microorganisms fixed to the support medium reached a high removal with 232±65 CU and 13.1±10.5 NTU in their effluent. The color and turbidity are related to solids in suspension in the wastewater, in this sense, the support medium fulfills a filtering function of these solids, retaining them by adhesion due to the formation of biofilm [34] while the same flow due to its low speed allows sedimentation, which favors the SSFCW to have a yield of 95.9% for turbidity and 89.4% for color, in the case of the FFCW the color and turbidity increased as a consequence of the growth of algae in the system [35]. Turbidity and color are parameters not regulated by NOM-001-SEMSARNAT-2021 [33], however, they are necessary in the control of a treatment plant [23].
Macrophytes are adapted to grow in water-saturated media or in aquifers because they have developed a system of large internal air spaces, these supply air from the atmosphere to the roots and rhizomes, provide good conditions for physical filtration and a surface large for attached microbial growth, favoring oxygen transfer to the rhizosphere, although estimates of the amount of this oxygen transfer vary over a wide range by species [28]. Macrophytes favor the water purification process, participating in the cycle of nutrient assimilation, gas production and disease control [42]. Niha et al. suggest that these effects are due to the influence of macrophytes on microbial communities [46]. The DO establishes the microbial environment, being anaerobic at low concentrations (0 and 2.5 mg L -1) as in the CW inlet water (RT1 0.6±0.2 mg L -1 and TR2 0.6±0.2 mg L -1) (Saeed and Sun, 2011), FFCW-SL and FFCW-W presented 4.5±0.7 mg L-1 and 6.8±2.2 mg L-1 respectively, while SSFCW-SL and SSFCW-W presented concentrations of 4.3±1.0 mg L -1 and 3.1±1.3 mg L -1. The DO results obtained are higher than those reported by Saeed et al., [10], who treated municipal wastewater in FVCW, horizontal flow Constructed wetlands (SSFCW) and surface floating flow wetlands (FSF) with mixed vegetation (Phragmites australis, Cyperus difformis, Dracaena sanderiana, Cyperus papyrus, Echinodorus cordifolius and Ludwigia adscendes) and HRT of 4 d, 8 d and 6.8 d, respectively, obtaining 0.1, 0.13 and 0.17 mg L -1 of DO in the effluents of the respective HA.
The pH is responsible for the development of microorganisms, biota and associated with these the degradation of contaminants and can be affected by acid or alkaline changes. In this study, the HA effluents presented values from 7.9 to 8.4, complying with the LMP of NOM-001-SEMARNAT-2021, which establishes an interval of 6.5 to 10 for discharge in national assets [33].The AH presented alkaline influents (RT1 with a pH of 8.3 and RT2 with a pH of 8.4) and the effluents of the treatments tend to be neutral and are considered slightly alkaline (FFCW-SL 8.1±0.1 and SSFCW-SL 7.9±0.1), these values did not show a negative effect on the adaptability of the CW, since the plants did not present mortality and continued their reproduction [36]. The results of this study compared with those of Winanti et al. [37], are very similar, since they evaluated a SSFCW with Canna Sp., obtaining neutral values of 7.0 with initial water of 8.15 and propose this standard value in wastewater treatment in universities or colleges in Indonesia.
Regarding the SDTs, the influent presented higher values than the FFCWs, these being the ones that reached the greatest removal, since the FFCW-SL removed 37.4% respectively, the FFCW-B removed 31.8%. Regarding the SSFCW-SL, they presented lower removal with 28.9% for SDT, being the lowest removals in the SSFCW-W with 28.4% for SDT. These values that managed to decrease are dissolved inorganic salts [37] and the removal cannot be higher because the CW do not have prolonged TRH to favor the elimination of ions or nutrients combined with the temperature effect [38]. The TDS values in the effluents of our study are within what is allowed for irrigation discharge (440 to 500 mg L -1), since the concentration of TDS that does not have harmful effects on any crop is 500 mg L -1 [39].
It has been reported that in SSFCW the removal of COD is high, reaching up to 90%, mainly due to sedimentation and degradation processes of degradable and biodegradable organic matter both in water and on the surface of the substrate [28]. The COD is proposed in the Official Mexican Standard NOM-001-SEMARNAT-2021 [33], where the strictest value for this parameter is 60 mg L -1 for irrigation of green areas, a value that is satisfactorily met in the SSFCW-SL treatment with 33.9±25.7 mg L -1, while the FFCW-SL reached 74.7±30.0 mg L -1 , complying with the permissible limit in the other receiving bodies. [40] treated domestic wastewater from a high school in Colombia, in two SSFCW with Typha latifolia and Cyperus papyrus, operating with 9 d of HRT, a flow rate of 15 L d -1 and an initial organic load of 305.4 mg L -1 of COD in the case of the wetland with T. latifolia and 293.2 mg L -1 for the wetland with C. papirus, obtaining a COD removal of 53.9% with T. latifolia and 47.9% with C. papyrus. If we compare other species used in CW in southeastern Mexico, [18]) evaluated the performance of Thypa latifolia in FFCW, Paspalum paniculatum in SSFCW, E. crassipes in FFCW and C. articulatus in SSFCW, whose TRH for FFCW were 7.5 d and 5.5 d for SSFCW. The removal efficiencies and median values (±SD) in the effluent for turbidity were 97.1% (4.1±1.7 NTU), 95.8% (6.0±1.7 NTU), 94.7% (7.6±1.0 NTU) and 85.4% (20.9±2.7 NTU) respectively; In the case of removal efficiency and median values (±SD) for color, the values were 83.4% (236.0±29.4 CU), 84.1% (226.0±69.9 CU), 79.6% (291.0±62.2 CU) and 71.6% (404.5±71.21 CU) respectively. For COD it presented 97.5% (9.9±48 mg L -1), 95.3% (18.9±5.3 mg L -1), 93.1% (27.3±8.5 mg L -1) and 86% (55.9±9.7 mg L -1) respectively. In this sense, Sagittaria latifolia was the best treatment, which is SSFCW-SL, presented similar yields to Thypa latifolia, since it reaches 95.9% (3.15 NTU) of turbidity removal, 89.4% (116.0 CU), for COD 95.7% (33.9±25.7 mgL -1), and a kinetic constant k:0.89 days -1. This is attributable to all the components, since the success of artificial wetlands depends on the whole (flow, vegetation, type, etc.) [43].
5. Conclusions
We can conclude that the species Sagittaria latifolia is feasible to implement in CW for wastewater treatment in decentralized communities such as those present in the RBPC and southeast Mexico. It is a species with good abundance in the area that can reduce operation and maintenance costs due to its availability, size and management. The process control parameters allow the treatment to be monitored at a lower cost and savings in analysis time, allowing the performance and quality of the treated water to be known on the same day of monitoring. The best performance was in the SSFCW-SL with Sagittaria latifolia, with turbidity, color and COD removals of 95.9, 89.4 and 95.7% respectively, the FFCW-SL reached removals of 98.8, 74.2 and 89.7% respectively. Sagittaria latifolia favors the oxygenation of the medium in the CW, since the DO concentrations were up to 4.3 mg L -1 in the treated water and, therefore, it presented good performance in the removal of basic contaminants. Finally, S. latifolia presents better management due to its size, which favors its operation and maintenance, unlike the species T. latifolia, which is the most used in the region.