Introduction
Maize, Zea mays (Poaceae) is an important grain crop globally as it is a food with high energy value and great consumption flexibility (Ranum et al., 2014). Brazil recorded the world's second-largest land area for transgenic crops in 2018, with 51.3 million hectares, representing 27 % of the global area for transgenic crops. Among these, the most harvested transgenic crops in the country include soybeans, maize (summer and winter), and cotton at 34.86, 15.38, and 1.0 million hectares, respectively, totalizing an adoption rate of 93 % (ISAAA, 2018).
The most common primary cause of postharvest losses in maize production is insect physical damage to the stored grains, such as the attack of maize weevil, Sitophilus zeamais (Motschulsky, 1955) (Coleoptera: Curculionidae), which causes qualitative and quantitative losses to grains (Abass et al., 2014). Its ability to initiate infestation in the field and attack intact grains until the storage phase justifies its importance in the maize production system (Caneppele et al., 2003).
The effect of herbicides on insect populations in maize fields should be considered in integrated pest management (IPM), since the molecules of these chemicals can have deleterious effects on arthropod species, including beneficial insects (de Menezes & Soares, 2016; Zanuncio et al., 2018). However, the interactions of different herbicides with different crop genotypes and insect species are particular (De Souza et al., 2020), making it difficult to obtain accurate information on the subject. The present study observed the emergence of adults of S. zeamais in genotypes of transgenic and non-transgenic maize sprayed with different applications of herbicides.
Material and methods
The study was first conducted on the field at the experimental station of the Institute of Agricultural Sciences of the Universidade Federal dos Vales do Jequitinhonha e Mucuri - UFVJM, in the municipality of Unaí, Minas Gerais State, Brazil. The medium-textured soil was fertilized according to recommendations for maize (Ribeiro et al., 1999).
The assay was a 3 × 5 factorial experiment in a randomized complete block design with four replications. The first factor comprises three maize genotypes, Herculex® and PowerCore®, both genetically modified and an Isohybrid (non-transgenic). The second factor includes five herbicide treatments: Atrazine, Atrazine + Nicosulfuron, Ammonium Glufosinate, Nicosulfuron, and control treatment without the application of herbicides. Each plot was 4.8 × 5.0 m, with 8 rows per treatment. The maize genotypes were sown at 0.6 m spacing between rows with eight seeds per linear meter. A drip irrigation system was used, when necessary, with a watering shift at 24-hour intervals. The border rows were planted with bell peppers and passion fruit.
The Herculex® maize genotype, event TC1507, features the Cry1F gene that confers resistance to attack by lepidopteran insects and the pat gene, originated from Streptomyces viridochromogenes, which confers tolerance to the activity of the Ammonium Glufosinate herbicide. The PowerCore® genotype, registered by the event MON89034 × TC1507 × NK603, has the genes Cry1F, Cry2Ab2, and Cry1A.105 that provide resistance to attack by lepidopteran insects and the genes pat and cp4-epsps (aroA: CP4) that confer tolerance to herbicides Ammonium Glufosinate and Glyphosate, respectively. A non-Bt isogenic maize hybrid (Isohybrid) of the same genetic background was used as a control. The herbicides were applied on the thirtieth day after sowing, when maize plants had four fully expanded leaves, with a pneumatically operated single nozzle sprayer equipped with a TT11002 flat jet nozzle calibrated to provide a spray volume of 150 L ha-1. Manual weeding was not performed on the control plots. The applied doses of the chemicals were 2400 g ha-1 of Atrazine, 2400 g ha-1 of Atrazine + 60 g ha-1 of Nicosulfuron, 400 g ha-1 of Ammonium Glufosinate and 60 g ha-1 of Nicosulfuron.
The harvested ears of maize were taken to UFVJM Campus JK, in Diamantina, Minas Gerais State, Brazil for drying to 13 % moisture content.
The second part of the study was carried out under controlled temperature (25 ± 1 °C), relative humidity (RH: 70 ± 10 %), and a 12-hour photoperiod in the Biological Insect Control laboratory. After threshing, 300g of grains in each plot were put in plastic pots in triplicates. The experiment was set up for 100 days. Six assessments of the natural emergence of S. zeamais were taken. Adults of S. zeamais were collected by sieving (mesh nº 60), counted, and transferred to the laboratory collection so that the subsequent evaluation did not have the same insects.
The S. zeamais emergence in the treatments was subjected to analysis of variance using the statistical program SISVAR (version 5.6). Means were compared using regression analysis. The degree of which the independent variable (genotype and genotype-herbicide interaction) determined the dependent variables (S. zeamais emergence) was estimated using nonlinear regression analysis according to Carvalho et al. (2014).
Results
The emergence of S. zeamais was affected differently by interactions between genotypes and herbicide treatments, as shown in Figures 1, 2, and 3, and Table 1.
The Isohybrid genotype, without herbicide exposure, showed a significant S. zeamais emergence throughout the period covered by the evaluation (Fig. 1, Table 1). Additionally, there was no emergence of S. zeamais for the Isohybrid with Atrazine application (Fig. 1).
However, with Nicosulfuron exposure, an emergence peak followed a Gaussian model in the non-transgenic Isohybrid genotype (Fig. 1). Treatments with the application of herbicides followed a similar response on S. zeamais emergence in the Isohybrid (Fig. 1, Table 1).
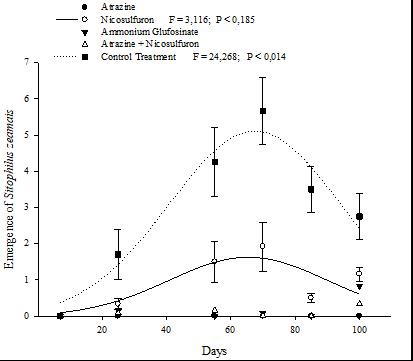
Figure 1 Emergence of Sitophilus zeamais in the Isohybrid genotype with different herbicide treatments exposure for 100 days.
In the Herculex® genotype, an emergence peak induced by Ammonium Glufosinate exposure followed a Gaussian model (Fig. 2). Additionally, with the application of Atrazine, Atrazine + Nicosulfuron mixture, and Nicosulfuron, a three-parameter Gaussian model was significantly fitted to describe the emergence behavior during the 100 days of evaluation (Fig. 2).
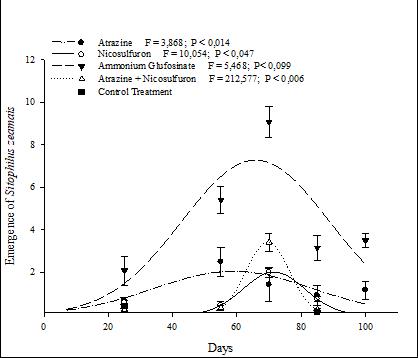
Figure 2 Emergence of Sitophilus zeamais in the Herculex® genotype with different herbicide treatments, for 100 days.
The PowerCore® genotype treated with Nicosulfuron or with Ammonium Glufosinate showed an asymptotic behavior growth of the S. zeamais emergence with upper asymptote after 55 days. On the other hand, there was no observed effect of applying Atrazine and Atrazine + Nicosulfuron at the S. zeamais emergence during the evaluated period (Fig. 3).
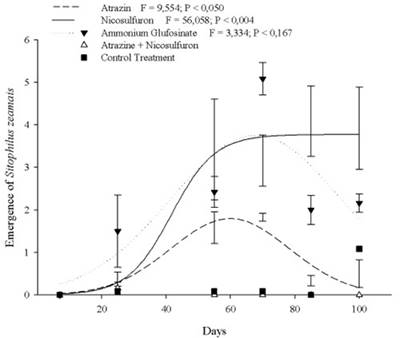
Figure 3 Emergence of Sitophilus zeamais in the PowerCore® genotype with different herbicides treatments, for 100 days.
The total mean of S. zeamais emergence showed no statistically significant difference between genotypes with Atrazine and Atrazine + Nicosulfuron application (Fig. 2, Table 1). The mean emergence of S. zeamais in the treatment without herbicide exposure differed between the Isohybrid and the other genotypes (Figs. 1 e 3, Table 1). Herculex® and PowerCore® genotypes were significantly affected by Ammonium Glufosinate (Table 1). PowerCore® was significantly affected in the emergence of S. zeamais with Nicosulfuron, especially between 55 and 90 days (Fig. 3, Table 1).
Table 1 The total means of the emergence of Sitophilus zeamais in grains of different maize genotypes with and without herbicide application
Genotype | Control | Atrazine | Atrazine + Nicosulfuron | Ammonium Glufosinate | Nicosulfuron |
---|---|---|---|---|---|
Isohybrid | 2.31 ± 4.43 b | 0.00 ± 0.00 a | 0.10 ± 0.30 a | 0.06 ± 0.16 a | 0.90 ± 1.59 a |
Herculex® | 0.03 ± 0.14 a | 1.28 ± 2.13 a | 0.71 ± 2.72 a | 3.88 ± 5.96 c | 0.60 ± 1.72 a |
PowerCore® | 0.89 ± 3.80 a | 0.78 ± 1.64 a | 0.22 ± 0.88 a | 2.19 ± 3.46 b | 2.47 ± 4.03 b |
Values followed by the same lowercase letter in the column do not differ by the Scott Knott test at 5% probability.
Discussion
Agrochemicals have been increasingly used, given their benefits in controlling pests, pathogens, and weeds (Pereira et al., 2009; Smith et al., 2008). Close to 300 commercially available herbicides target 27 different mechanisms within plants, including enzymes involved in the biosynthesis of carotenoids, chlorophyll, amino acids, fatty acids, lipids, and cellulose. Low applications rate of some herbicides to specific crops can increase biomass, growth, protein content, and disease resistance (Cutulle et al., 2018; Xin et al., 2012). On the other hand, the insertion of a foreign gene in plants can cause unintended phenotypic alterations (Jiang et al., 2017; Ladics et al., 2015; Li et al., 2015). Additionally, chemical stress induced by herbicide application can affect plant composition and insect attractiveness, mainly due to the complex mechanisms involved in stress response caused by herbicide non-target sites on both GMOs and non-transgenic plants (Ghanizadeh & Harrington, 2017; Herman & Price, 2013). For Isohybrid without herbicide application, there were significant emergence peaks of S. zeamais during the evaluated period when compared with the Herculex® and PowerCore® genotypes. Plant genotypes can show differences in metabolic phenotype (Ren et al., 2009), affecting S. zeamais preference (Frazão et al., 2018). Herbicides can interact with genotypes, altering metabolic pathways or plant development and attractiveness to insects (De Souza et al., 2020). The insect attractiveness is based on olfactory orientation and variations in the ratio of volatile components produced by plants (Bruce et al., 2005; Toshova et al., 2010). The presence of phenolic compounds in maize grains is one of the factors related to resistance to attack by S. zeamais (Demissie et al., 2015). When modifications by these interactions are carried out, unintended changes in the quality of plant resources due to the pleiotropic effects can occur, affecting the insect-plant interaction (Ladics et al., 2015; Schuler et al., 1999). In this sense, the Isohybrid evaluated in this study may have favorable chemical stimuli involved in the attraction of S. zeamais for oviposition and development.
The S. zeamais emergence in the Herculex® and PowerCore® genotypes, when Ammonium Glufosinate was applied, was high during the evaluated period. The pat gene, isolated from Streptomyces viridochromo genes Tii494 (Strauch et al., 1988), encodes the phophinothricin-N-acetyl transferase enzyme, which catalyzes an NH2 acetylation reaction of ammonium glufosinate, inactivating it in a non-toxic form (Krenchinski et al., 2019). Therefore, herbicide exposures can induce plant stress that affects the general biochemical pathway, including herbivore defensive mechanisms (Dyer, 2018; Xin et al., 2012). These interactions may vary according to the studied genotype. It is known that the Herculex® and PowerCore® genotypes have the pat gene that confers tolerance to Ammonium Glufosinate, indicating the possible effects of these interactions.
The selectivity of Nicosulfuron in maize is related to the rapid detoxification of this herbicide, transforming it into non-phytotoxic compounds (Fonne-Pfister et al., 1990). Sweet maize (Zea mays L. var. rugosa), when treated with Nicosulfuron, showed a lower number of amino acids and an increase in the total amount of sugars (Cutulle et al., 2018), which may have reflected in the greater preference for the S. zeamais in the PowerCore® genotype. Besides, the tolerance of maize hybrids to post-emergent herbicides in the sulfonylurea group is quite variable and may be high for some and reduced for others (Cavalieri et al., 2008), which may explain the low emergence of S. zeamais in the other genotypes.
The lowest means of S. zeamais emergence exhibited in genotypes with Atrazine could be related to the absence of chemical stimuli involved in host plant selection for oviposition with this chemical. Interactions mechanisms between S. zeamais and maize plants in agroecosystems remain poorly understood and require more attention when compared with other maize insect pests (Brown & Lee, 2002; Ni et al., 2012). For example, the attractiveness of S. zeamais in maize grain is influenced by aspects such as hardness, size, and quantity of protein and starch (De Groote et al., 2017; Dobie, 1974). Differences in these parameters may have occurred in the genotypes used in this study.
Interactions between maize plant genotypes and S. zeamais still need to be better understood, particularly how attractiveness parameters are affected by foreign gene insertion and herbicide stress in the field and storage. In this work, evidence of herbicide interference in both transgenic and non-transgenic maize for S. zeamais colonization success was observed. Efforts to better understand the factors that influence the S. zeamais and maize plant relationships are necessary to develop management strategies to reduce S. zeamais infestation in storage maize.
Conclusions
The insertion of an exogenous gene and herbicides applied in the field in maize plants can change components of the insect-plant interaction, with the Isohybrid being more attractive to S. zeamais when herbicides are not applied. The applications of Atrazine and Atrazine + Nicosulfuron herbicides can potentially reduce plants' attractiveness to S. zeamais. Ammonium Glufosinate increased S. zeamais preference for the Herculex® and Nicosulfuron for PowerCore®.