Introduction
The Cocoa Agroforestry System (CAS) is one of the most important, which are distributed in the states of Tabasco and Chiapas (South of Mexico). However, in recent times, this system is facing phytosanitary problems, coupled with low yields due to inadequate crop management (Bautista-Mora et al., 2016), which is causing that currently is cutting plantations in favor to other crops. In accordance with Don et al. (2011), the soil use change is the second most important source of variation that man has caused to induce CO2 emissions. In particular, deforestation in the tropics and subtropics causes loss of biomass and soil organic carbon (SOC). The soil use change can affect SOC levels due to the variation of production, which can reach to the soil and by acceleration in the decomposition rate of organic wastes (OW). Therefore, factors such as crop management, some species are being incorporated, as well as the OW characteristics, affect the SOC storage capacity (Jandl et al., 2007). According to Schroth et al. (2015), CAS can accumulate a significant amount of tree biomass (which means: storage of soil organic carbon in high amounts, sometimes even higher than some natural forests).
Other important aspects which are modified by the reduction of the soil organic matter (SOM) content, are the contents of soil total nitrogen (STN) and assimilable forms of soil nutrients.
After the humification process, in which a microbial activity intervenes (after the crushing caused by soil mesofauna), being responsible for the release of nutrients, which can be absorbed by plant roots, aided by the hyphae of mycorrhizal fungi. In Tabasco-México, the largest surface area of CAS is distributed in the region of the Chontalpa, an area where the grassland system has recently registered a significant increase in its surface area (Ramos, Sánchez & Gama, 2016). However, modifications in physical, physicochemical and biochemical properties have not been sufficiently evaluated (in particular, SOC storage), which are being triggered by this change in soil use. Given these concerns, the aim of this research was to evaluate the modification of SOC levels and other edaphic variables (edaphic density, penetration resistance, cation exchange capacity (CEC), STN and C/N ratio), which are indicative of edaphic fertility in a chronosequence of established pastures after cacao plantations felling.
Material and methods
Study area
The research was carried out in the communities Iquinuapa, Hermenegildo Galeana and Soyataco belonging to the municipality of Jalpa de Méndez, Tabasco-Mexico, places that are located within the geographical coordinates 10º 33´ N and 93º 45´ W (Figure 1).
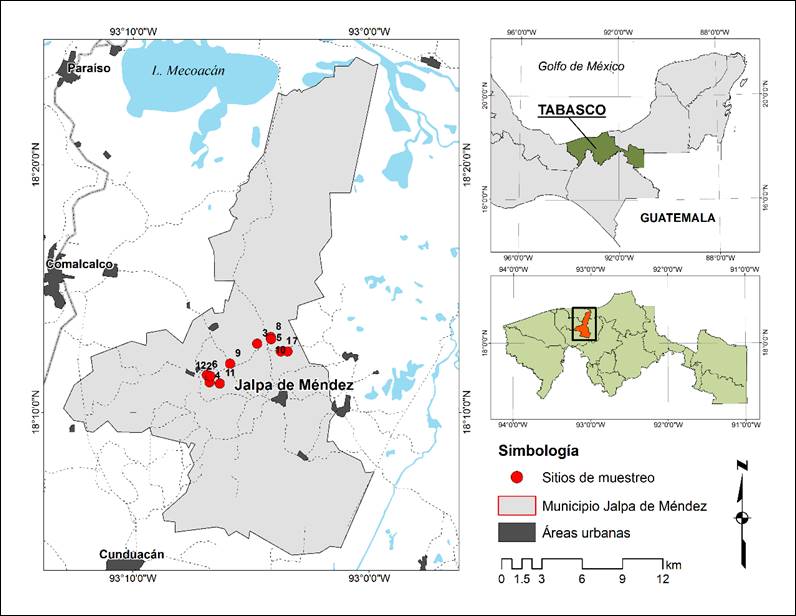
Source: INEGI (2006)
Figure 1 Location of sampling sites in communities of Jalpa de Méndez, Tabasco-México.
Weather conditions
The climate corresponds to a warm-wet, with abundant rains in summer. The average annual temperature ranges between 24 and 28°C and the average annual rainfall between 1500 and 3000 mm.year-1 (INEGI, 2006).
Geological components
The dominant geological materials are deep sediments, which were carried by numerous rivers and streams that cross the zone from the weathering of the Sierra rocks and the soil hills erosion, mainly during the recent Quaternary period. These materials, which are composed of different mineral mixtures, were finally deposited by a continuous avenues, constituting currently a great land extensions (Palma et al., 2007).
Soil types
The soils were formed from such Quaternary sediments, they are young soils, in any case from Holocene period (Palma et al., 2007). According to the soil map provided by: Jiménez et al. (2013), predominate the Gleysols soils.
Plant cover
According to Palma et al. (2007), the native vegetation of this area corresponds to a medium-evergreen forest. However, this type of vegetation has been replaced by a native and improved grasslands, where are still conserved the Cocoa Agroforestry System (CAS), which have a great diversity of flora, highlighting approximately 28 families, 58 genera and 67 species (Sánchez-Gutiérrez et al., 2016), among them being the most important: Erythrina americana Mill., Erythrina poeppigiana (Walp.) O.F.Cook, Gliricidia sepium (Jacq.) Walp. (Fabaceae), Cedrela odorata L. (Meliaceae) and Colubrina arborescens (Mill.) Sarg. (Rhamnaceae).
Soil samples
Sites which were formerly CAS were selected, due they underwent a change in soil use. In order to select the sampling sites in a first stage, a survey was carried out with the producers in order to know their crop management record, in particular the number of years since the change of soil use. From the survey results, was determined the major change of soil use, which was from CAS to grassland (GL). The years of soil use change, which were reported by farmers, were organized into a database, establishing soil use ranges as follows: 1-5 years (GL5), 6-10 years (GL10) and 11-20 years (GL20). Each time interval was considered as treatment, with four plots (replicates) for each treatment. As reference treatment (control), CAS plots of 20-35 years of establishment (CAS35) were sampled, where each plot was considered as an experimental unit.
Determination of soil physical properties
A first soil sample set was taken with a cylindrical sampler of known volume for the edaphic density determination. In each experimental unit, 10 sampling sites were located. Alternatively, at each soil sampling site, soil samples were taken at three depths as follows: 0-10, 10-20 and 20-30 cm, respectively. Soil samples were dried in a forced air oven at 105°C until constant weight. The dry weight of soil sample was related to the volume of the cylinder for to calculate the edaphic density (ρ b). In addition, the penetration resistance was measured in situ with a Bush SP1000 ELE penetrometer fitted with a force sensor and a displacement sensor. Conversely, a standard cone was used (ASAE, 30°) with a diameter at the base of 1.28 cm. The approximate insertion velocity was 5.0 cm. s-1 and the penetration resistance was expressed in MPa.
Determination of soil biochemical properties
Another research stage, consisted in obtaining composite soil samples (from 10 subsamples per experimental unit) taken at -30 cm soil depth. These samples were dried in the shade, crushed in plastic trays and then sieved to obtain a particle size of less than 2.0 mm. In addition, these samples were determined SOM by the Walkley & Black method, STN by Kjeldahl semi-micro method and CEC by the ammonium acetate extraction method, processes that allows to generate a subsequent quantification by atomic absorption, respectively. The SOM percentage was calculated using 1.72 factor from SOC value. In fact, ρ b was used to transform the SOC results obtained as Mg of C. g-1 to values of Mg of C. ha-1.
Data analysis
The collected data were analysed using the GLM procedure from Statistical Analysis Systems (SAS, 2002) for Windows, version 6.12 (r). To identify significant difference among treatments and statistical significance for all comparisons was made at p<0.05. Tukey's multiple range test was used to compare the mean values of treatments.
Results
Soil physical properties
In the edaphic density (ρ b) variable, statistical differences were observed for each treatment factor and soil depth factor (Table 1). In the soil surface horizon (0-10cm), the treatment GL20 performed the highest significantly value ρ b, being of 1.3 Mg. m-3 (Table 1). In fact, the lowest value with GL5 and GL10, which were statistically equal to CAS35 (1.00 Mg. m-3).
It was observed that in Table 1, in general, as the soil depth sampling increases, the ρ b value increases in each treatment. It is known that, is due to the fact in the first soil surface horizon (0-10 cm) a higher SOM content accumulates, which decreases with respect to soil depth, the effect on soil structure (and, therefore, the soil porosity), decreases significantly. Therefore, as the soil depth sampling increases, a significant increase in ρ b in each treatment can be observed. (Table 1), which were inversely correlative to the SOM concentration.
Table 1 Edaphic density (ρ b) values and soil compaction (0-30 cm) with different soil uses (grassland (GL) and Cocoa Agroforestry System (CAS)) in the Southeast of Mexico
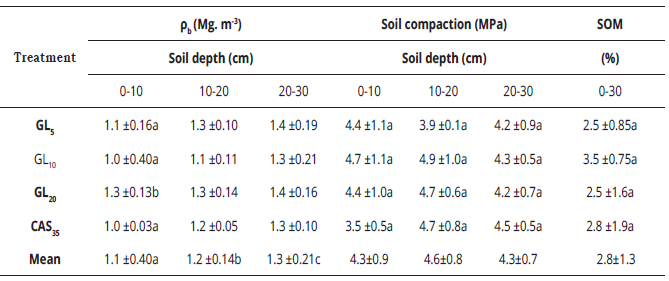
§ Different letters indicate statistically significant differences (P <0.05).GL: Grassland; CAS: Cacao agroforestry system; SOM: Soil organic matter.
There were no statistically significant differences in relation to soil compaction values in the soil surface horizon, both for treatment factor and soil depth factor (Table 1).
Soil physical-chemistry properties
In relation to soil CEC, it was significantly higher in CAS35, GL5 and GL10, which were statistically equal to each other (27, 27 y 23.7 Cmol(+).kg-1, respectively). A significantly lower value of CEC, was obtained in the longest grassland (GL20; Table 2) being between 15 and 20 Mg. g-1 for SOC and 1.5 to 1.6 Mg. g-1 for STN.
Soil biochemistry properties
There were no statistical differences in the contents of SOC and STN among different treatments (Table 2).
Table 2 SOC contents and some edaphic chemical properties (0-30 cm) of a chronosequence from grassland (GL) to CAS in the Southeast of Mexico
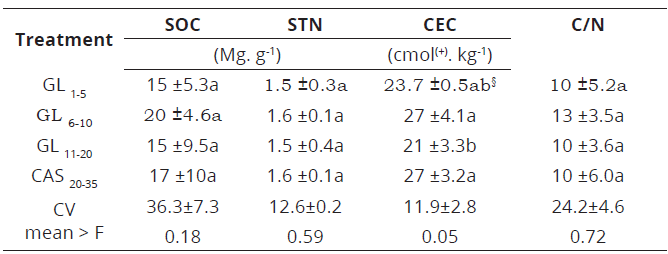
§ Different letters indicate statistically significant differences (P <0.05).GL: Grassland; CAS: Cacao agroforestry system; SOC: Soil organic carbon.
The C/N ratio in CAS is also significantly similar to the grassland systems (GL) (Table 2), a consequence of high soil spatial variation. Regarding the vertical distribution by treatment of SOC, in fact, was significantly higher in soil depth of 0-10 cm for the following treatments: GL10, CAS35 and GL5 (3.3 mg of C. g-1), respectively, among which there was no statistical difference (Table 3). The GL20 treatment performed the lowest SOC percentages (being 21 mg of C. g-1). For soil depth of 10-20 cm and 20-30 cm, the SOC did not present significant difference for each treatment (Table 3), being in any case, significantly lowest to those for edaphic surface horizon.
Discussion
Soil physical Properties
It is important to note that a ρ b increase by the continuous cattle overgrazing is common in the evaluated grasslands, in fact is correlated to soil compression in the surface soil horizon, reducing the porous space and a ρ b increased, which is what is observed in GL20. In addition, SOM content is significantly the same, likely a result which cannot be attributed to its quantity, which promotes less soil aggregation. In this sense, a change in soil humic quality is not detectable since no significant variation in the soil C/N ratio is observed (Table 2). Nascente, Yuncong & Costa (2015), found that SOM concentration was negatively correlated with ρb in the surface soil horizon (0-10 cm), which are thought to contribute to a higher concentration of SOM with a lower ρ b.
As a result, it has long been thought that a high soil compaction and high ρ b affects negatively the agroforestry system productivity, since both conditions have allowed an adequate root plant penetration. It was observed that plant nutrition becomes difficult, due to internal air flows, water and heat, which are limited before the porous space decreasing.
Soil physicochemical properties
A significantly lower value of CEC seems to indicate a loss of edaphic quality after 20 years of soil use change (from the grassland (GL) to cacao agroforestry system (CAS)), and provides more accurate and reliable estimates of the C/N ratio values, which were not modified with soil use change.
Liang et al. (2005), indicated that regardless of the SOC amount, the organic waste decomposition rate regulates many biogeochemical processes, specifically the nutrient cycles and retention. In addition, these organic wastes are mostly degraded, increasing the aromatic compounds (humification), which can be adsorbed on the clay minerals surface, which causes a greater specific soil surface area, being reflected in a greatest CEC, as it should have happened in the CAS of the present research. In fact, this clay-humic complex goodness of the agroforestry system is evident a deterioration with cattle overgrazing, since both the edaphic apparent density (ρ b) and CEC, deteriorate in a medium term (GL20).
For instance, the regression coefficient between SOM and CEC variables was 0.65 (r = 0.02), indicating no significant correlation (Table 1), which indicates the C/N ratio have not allowed to detect the soil use change in terms of the SOM content, is believed to be an outcome of enzymatic activities.
Soil biochemistry properties
Physical and chemical properties evolve favorably on the edaphic surface (e.g. 0-20 cm). CAS may maintain significant amounts of SOC in a reasonable time (e.g. six years) of establishment. Thereafter, the edaphic properties tend to stabilize at similar levels to secondary forests in their natural state (Arévalo-Gardini et al., 2015).
It is known that high coefficient of variation (CV) of the results prevents significant differences in these properties from being observed in the present study, indicating more sensitive parameters, such as enzymatic activities, since the soil use change after CAS deforestation, which implies a bare soil. Therefore, there must be an obligatory temperature and humidity regime modification in CAS, which modifies the mineralization speed of the organic wastes (litter or mulch) provided by the CAS tree vegetation for several years.
Aceñolaza & Gallardo (1999), found that thinning of the montane alder forest of the Argentine Yunga, increased the soil dryness, which moderated the organic wastes decomposition, while the canopy development was accelerated. During the first years of the soil use change, there may be a greater nutrient release as a result of an increased mineralization.
In fact, in a previous work, a chronosequence of grassland from 3 to 56 years of age, was evaluated. Their results indicated that 15 year old grasslands had a higher amount of C and N due to the age, the grassland system accumulated more SOM, coming specifically from plant and root organic wastes, which generated a higher SOC contribution and other nutrient uptake. After that age, there was a decrease in the release of chemical elements in such systems (Cheng & Shao (2015); Jie, Cheng, Li & Liu (2012); Tadakatsu, Ohkura & Matsumoto (2015)). Alternatively, Huang et al. (2010), mentioned that over the years, grasslands lose their vocation to accumulate SOC due to a decrease in the system productivity, mainly due to an increasing degradation of its properties, motivated by cattle overgrazing and the type of grassland established. Accordingly to Goodrick et al. (2015), some tropical agroforestry systems slowly reduce the SOC content due to the higher N contents that legumes procure (which speeds up the mineralization processes). In addition, a higher STN concentration and higher mineralization, reduces the C/N ratio, resulting in a greater SOM decomposition. Other research has documented that under certain conditions, by switching from crop to grassland, an increase in SOC levels, which can be achieved by 26% (Don et al., 2011). On the other hand, Johnson et al. (2007), conducted a study in which they revealed the SOC amount, which can be stored in the soil as a function of the organic wastes amount produced and entering into the soil, for instance, soil composition and soil decomposition rate, noting that roots of the grasses decomposition have allowed a great amount of recalcitrant compounds.
The results found for the three first edaphic layers studied (Table 3). These results are comparable in variability to the report by Céspedes et al. (2012), who indicated the highest SOC content in prairies and grassland is concentrated in the first -10 cm, concentrating from 41 to 62% of the SOC (referred to SOC found at -1.0 m soil depth) in the first -20 cm. Alternatively, the results indicate that grassland after 20 years of establishment can accumulate more than twice the amount of SOC than soils with secondary vegetation over 50 years of age. In addition, they indicated in the grasslands more than 50% of SOM, which was located in the first -20 cm, whereas in the secondary succession it was only 23-31% of the SOM (for that same soil depth). However, in the present study (under conditions of the humid tropics) after 20 years of soil use change (GL20) of CAS, the SOC in the 0-10 cm soil layer decreased, indicating the humification of the organic wastes did not compensate for the loss of SOM mineralization, which negatively affects the soil structure (increase of ρb) and porosity.
Conclusion
The soil use change from CAS to grassland does not significantly reduce the SOC stores (referred to 0-30 cm soil depth) in a significant way, have achieved the soil C/N ratio. In fact, a high soil spatial variation obliges us to look for other more sensitive indexes to measure the possible impact. Given these concerns, it is possible to observe a negative modification which affects the edaphic density at medium term CEC (20 years) in the first -10 cm soil depth, parallel to a significant fall of the SOC to that same soil depth, which ends up negatively affecting the soil fertility.