The world production of cheese for the year 2020 amounted to approximately 21.69 million metric tons, being the European Union the main producer of cheese in the world with a production volume of around 10.35 million metric tons (FAO, 2020). Whey constitutes approximately 90% of the total volume of milk after cheese making. It contains more than half of the soluble elements such as sugars, salts, proteins, and fats, among others (Asas et al., 2021). The nutritional value of whey is given by the amount of proteins, minerals, and amino acids present in it, among the most abundant proteins found in this co-product, are β-lactoglobulin and α-lactalbumin, which correspond to 10 and 4% of all milk protein, respectively; among the most notable amino acids are tryptophan, leucine, and lysine, which are essential amino acids; and in terms of minerals, potassium, calcium, phosphorus, sodium, and magnesium stand out, in addition to the B vitamins, being pantothenic acid and ascorbic acid found in greater quantity with concentrations of 3.4*10-3 g L-1 and 2.2 *10-3 g L-1, respectively (Maticorena et al., 2018).
The final disposal of whey constitutes an environmental problem because it is usually discarded in water sources, and due to the high load of organic matter it contains, it causes a relatively high oxygen demand to be produced, ranging from 30,000 mg O2 L-1 to 50,000 mg O2 L-1 (Agualongo et al., 2022). Membrane filtration is a technology used to separate one or more compounds from a solution, and in the case of whey, it has been used to separate proteins and sugars, among others, with various applications in the food industry and other sectors (Iltchenco et al., 2018). Whey permeate obtained by ultrafiltration, containing lactose and minerals, can be considered a raw material to be used in food products, particularly for fermented dairy beverages, because lactose is found in significant quantities as a structural carbohydrate, which allows the growth and multiplication of lactic acid bacteria.
The Food and Agriculture Organization (FAO, 2020) defines a fermented dairy beverage as a milk product obtained through the fermentation of milk, which may have been made from products obtained from milk with or without modifications in composition, through the action of suitable microorganisms and resulting in a reduction in pH with or without coagulation (isoelectric precipitation). Montesdeoca et al. (2017) and Santos et al. (2008) have shown that by substituting milk for sweet whey in percentages between 30% and 40%, dairy beverages with high acceptability are produced, both in sensory and physical-chemical tests, showing optimal characteristics for its possible consumption and commercialization. Different authors have also used several types of flour and fruit in whey-based dairy beverages to obtain better viscosity and consistency (Gavilanes et al., 2018; Marulanda et al., 2016; Rodríguez and Hernandez, 2017; Muñoz et al., 2019). Pescuma et al. (2010) used whey, WPC, and lactic acid bacteria for the elaboration of a functional beverage, finding that it had improved characteristics, such as reduced content of β-lactoglobulins and increase in essential branched-chain amino acids.
It is currently estimated that two-thirds of the world's adult population suffer from lactose intolerance (Alcalá et al., 2021). Enzymatic hydrolysis through the enzyme β-galactosidase is commonly used in the dairy industry, causing the breakdown of lactose, and achieving easy digestion of these foods, thus, this population can consume dairy products. In addition, this splitting not only solves this problem but also increases the power of sweetness in the food.
The sugars used in the food industry define the degree of sweetness of the final product and also affect the physicochemical and techno-functional properties of various food matrices. Thereon, tagatose is a monosaccharide sugar remarkably similar in its structure to fructose, which can be an alternative to replace sucrose in various applications, presenting a sweetening power of 92% and 38% fewer calories than sucrose (Alvarez and Molina, 2017). Various entities such as the FAO, the WHO, and the European Union accepted it as a safe food ingredient because it has prebiotic effects and helps in the treatment of diseases related to obesity and diabetes (Manzo et al., 2019).
At present, there is little or no development of fermented dairy beverages using simultaneously milk, sweet whey permeate and ultrafiltration concentrate subjected to hydrolysis process. The objective of this study was to develop a hydrolyzed and non-hydrolyzed fermented dairy beverage from ultrafiltration permeate, whey protein concentrate, and whole milk sweetened with tagatose, evaluating its physicochemical and techno-functional stability over time under controlled refrigeration.
MATERIALS AND METHODS
Raw Materials
Whole milk: it was obtained from the dairy facilities of the Paysandú farm, property of the Universidad Nacional de Colombia (Medellín Campus). Ultrafiltration Permeate (UFP) and Protein concentrate (WPC): These products were obtained from 250 L of whey ultrafiltration using a PERINOX membrane filtration system equipped with a semi-permeable polyethersulfone spiral membrane with a 10 kDa cut-off size and the following operating conditions: pressures of 1 and 3 bars at the outlet and inlet, respectively; a temperature of 48 °C, and a concentration factor of 18 (Vargas, 2017). Both the UFP (240 L) and WPC (10 L) obtained were stored under refrigeration at 4 °C until the dairy beverage was prepared. Starter culture: DRI-SET 432, a lyophilized microbiological culture provided with Lactobacillus delbrueckii subsp. bulgaricus and Streptococcus salivarius subsp. thermophilus lactic acid bacteria, provided by the company VIVOLAC. Tagatose: Tagasweet was acquired from the company BIOFOODS. Starch: Thermflo (waxy corn base) E 1440 was provided by the company INGREDION. Stabilizer: Pectin LM 35 from the company INGREDION and Sodium Citrate from the company TECNAS.
Raw materials characterization
For whole milk, the content of total solids (ICONTEC, 2001), proteins (AOAC, 1996), fat (AOAC, 2000), acidity as a % lactic acid (ICONTEC, 1999), density (AOAC, 1995), cryoscopic point (ICONTEC, 2013), and pH (ICONTEC, 1999).
The UFP was characterized by measuring the pH according to the method (AOAC, 2000), acidity (% lactic acid) (AOAC, 1997), total soluble solids (AOAC, 2000), lactose by liquid chromatography (HPLC), proteins (AOAC, 2009), minerals (ICONTEC, 2003), and ash (AOAC, 1990). The WPC, total soluble solids (AOAC, 2000), proteins (AOAC, 2009), α-lactalbumin and β-lactoglobulin were determined by liquid chromatography (HPLC) using a Shimadzu chromatograph and following the methodology proposed by Elgar et al. (2000), and density (AOAC, 1995). Table 1 presents the corresponding characterization.
Formulation of the dairy beverages
The beverages formulation was carried out through a simplex-centroid mixture design: 40% <milk<47%, 51%<UFP<58% and 2%<WPC<5% (Table 2). This design was conducted for both the hydrolyzed beverage (HFDB) and the non-hydrolyzed beverage (NHFDB) with total soluble solids of 11%, in which sucrose was replaced by TagaSweet Tagatose (Biofoods) to achieve these values.
In each treatment, the whole liquid milk was mixed with the UFP and WPC, in the case of the HFDB, commercial lactase 0.4 g L-1 was added to these mixtures at 4 °C for 24 h. The NHFDB and the HFDB, it was subjected to a heat treatment at 42 °C, adding starch (1.3%), sodium citrate (0.2%), and the stabilizer (0.3%). Subsequently, the mixture was homogenized at 17.23 MPa and pasteurized at 85 °C with 15 min of retention time and cooled to 45 °C when the process of inoculation with biological culture (DRI-SET 432) was realized. The mixture was incubated at 42 °C until reaching 0.52% lactic acid (Imbachi, 2018) and stored at 4 °C to sweetening with Tagasweet under continuous stirring.
Physicochemical analysis in the fermented dairy beverages
Acidity was measured by titration (ICONTEC,1999). The pH was determined with an OHAUS STARTER 3100 potentiometer (ICONTEC, 1999). The syneresis index was performed by taking 20 g of sample and subject to centrifugation at 1250 rpm for 20 min at 4 °C, the supernatant was separated by pouring, weighed, and recorded as syneresis (g 100g-1 of sample) (Amaya et al., 2008). The determination of viscosity was established employing the flow curves at 4 °C using a Brookfield DV-III ultra rheometer with the SC4-27 spindle (Ramírez and Vélez, 2013). Viscoelastic behavior within the linear viscoelasticity range was determined utilizing a frequency sweep (0.01-100 Hz) using an Anton Paar rheometer (MCR-302) coupling a cone/plate geometry with temperature control at 4 °C. The protein was determined by the Kjeldahl method (AOAC, 2009). Soluble solids were expressed as Brix degrees (oBx) and quantified using a digital refractometer (HI 96801) (Baldasso et al., 2011). For the percentage of hydrolysis, glucose, galactose, and lactose carbohydrates were measured by means of HPLC agilent technologies Series 1200 with an aminex HPX-87H ion exchange column (300 x 7.8 mm), and as a mobile phase solution of H2SO4 0.008 N at a constant flow of 0.6 mL min-1 (Beltrán and Acosta, 2012).
Sensory analysis in fermented dairy beverages
The sensory analyzes were carried out in two stages: the first one was performed by 20 expert technicians belonging to the Laboratory of Dairy Products of the Universidad Nacional de Colombia at Medellín campus, which was effected using a 5-point Hedonic scale, where the general acceptability of the 10 hydrolyzed fermented dairy beverages (HFDB) and the 10 non-hydrolyzed fermented dairy beverages (NHFDB) was evaluated, information that was used for choosing the best hydrolyzed and non-hydrolyzed beverage. In the second stage, a sensory profile was implemented by multidimensional approximation for the fermented dairy beverage samples according to the methodology indicated by NTC 3932-1996 (ICONTEC, 1996). This analysis was conducted by a panel of expert judges made up of 5 people between the ages of 25 and 60 years old. The relevant descriptors on the sensory attributes were identified and selected, assessing the intensities on a rating scale from 0 to 5 for all the descriptors except for overall quality, where a scale from 1 to 3 was used, 3 being the highest value and 1 the lowest value.
Stability of the fermented dairy beverage
In this phase, a batch was prepared for the best HFDB and another for the best NHFDB from the optimization of the statistical mixture design. The samples were packed in polystyrene containers -commercial presentations of 200 g - with a screw cap and left in storage at 5±1 oC. Measurements were made on days 0, 7, 14 and 21 for the variables pH, lactic acidity %, and syneresis index.
Statistical analysis
Phase I: A mixture design was carried out, evaluating the results with an ANOVA at 5% using the statistical program Statistica (StatSoft, version 12), with adjustment to a special cubic model (this model was used because presents the best goodness of fit with R2=0.98). The optimization process was performed using the multiple response desirability technique. Phase II (Stability study): the factor was the type of HFDB and NHFDB, performing four readings for a study time of 21 days. The results were analyzed using an ANOVA (α=5%) and mean difference test over time using Fisher’s LSD method (α=5%).
RESULTS AND DISCUSSION
The physicochemical results of the non-hydrolyzed (NHFDB) and hydrolyzed (HFDB) fermented dairy beverages of the mixture design are shown in Table 3 and Table 4, respectively. For the analysis of the mixture design, optimization was performed by minimizing the response variable syneresis and maximizing general acceptability and protein content variables (Figure 1A and B). Optimization analyzes showed values of WPC (5%), UFP (52.2%), and milk (42.8%), for the NHFDB (R2=0.9772). For HFDB, the optimal formulation under the same conditions (R2=0.9798) was WPC (5%), UFP (51%) and milk (44%).
Table 3 Physicochemical characteristics and sensory evaluation of non-hydrolyzed dairy beverages under the different formulations (NHFDB).
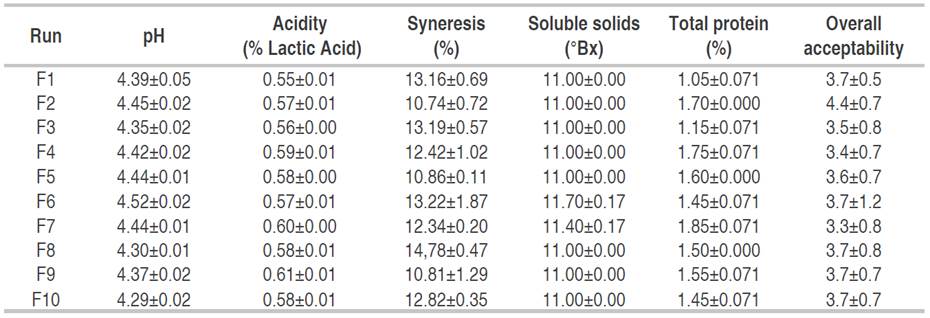
Table 4 Physicochemical characteristics and sensory evaluation of hydrolyzed dairy beverages under the different formulations (HFDB).
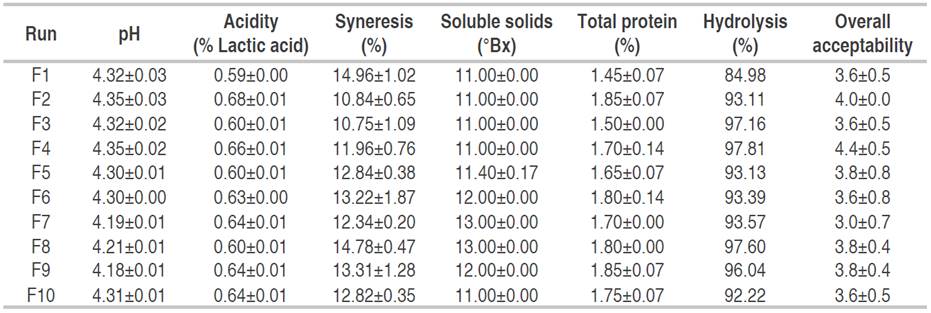
Characterization of selected beverages
The formulations selected through the mixture design were experimentally validated (Table 5). Regarding the value of total soluble solids (11.375 °Bx), they were similar to the sweet whey-based dairy beverages reported by Gavilanes et al. (2018) whose value was 12.87 °Bx. Nogueira et al. (2016) reported a soluble solid value of 10 °Bx for a dairy beverage fermented with Kefir. The pH and acidity of the dairy beverages developed in this study presented values similar to those reported for fermented dairy beverages developed by Gavilanes et al. (2018): 4.42<pH<4.68, 0.63<acidity<0.68% expressed as lactic acid. Miranda et al. (2014) reported acidity values of 0.63% (expressed as lactic acid) and a pH value of 4.36. The protein (1.74% for both beverages) coincides with the values reported by Almeida et al. (2001) of 1.94% for a fermented dairy beverage made with 50% sweet whey. Also, the syneresis values of 10.69% for NHFDB and 10.72 for the HFDB are similar to those reported by Zambrano and Zambrano (2013), who found a value between 9.90-10.10% using 30% whey in the beverage formulation.
The rheological results showed a pseudoplastic behavior with the best fit using the power law model (R2>0.99) where the apparent viscosity decreases with the increase in shear rate (Figure 2A). This behavior (non-Newtonian) was also observed by Pacheco et al. (2017) in beverages with whey, skim, and whole milk, indicating a continuous reorganization of the molecular weight structure, resulting in less resistance to flow due to the presence of a high molecular weight substance such as corn starch. This rheological result has also been found by Colominas et al. (2019), on a fermented beverage of whey, mango pulp, and rice flour.
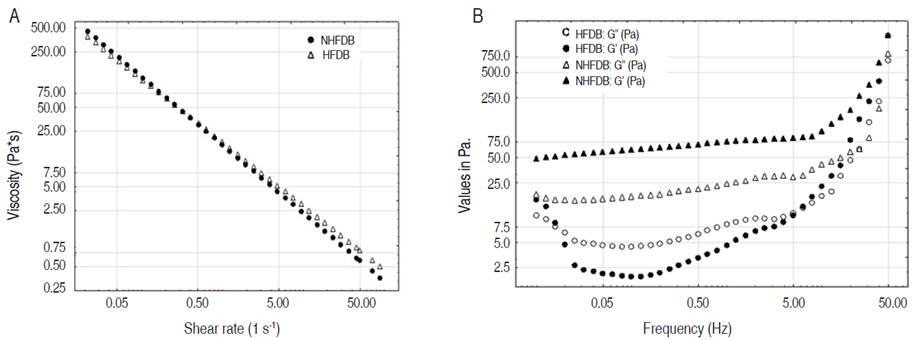
Figure 2 Rheology of fermented dairy beverages: A. Rheogram; B. Viscoelastic behavior (frequency sweep at 4 °C).
For the optimal dairy beverages (Table 5) there were no statistically significant differences (P>0.05) in the variables evaluated. The consistency index (K) and the fluidity index (n) found in this study are similar to those reported by de Castro et al. (2009) who evaluated oligofructose in probiotic dairy beverages, reporting values of 17-20 Pa.sn for the consistency index and between 0.11-0.22 for the fluidity index expressing the pseudoplastic behavior of the material. Additionally, evaluating the apparent viscosity at a shear rate of 50 s-1, since this value would represent the approximate viscosity felt in the mouth (Díaz, 2018), viscosity values of 0.587 Pa.s were obtained in the HFDB and 0.7919 Pa.s for the NHFDB, qualitative values that coincide with the sensory evaluation of the product (Figure 3). Moreira et al. (2014) found values from 0.5338 to 0.6609 Pa.s, under 50 s-1 conditions, with pseudoplastic behavior in a dairy drink with soy milk and lecithin. Pachekrepapol et al. (2021) for a non-hydrolyzed and hydrolyzed beverage, presented a higher apparent viscosity for the non-hydrolyzed beverage because of the larger particle size of galactooligosaccharides tends to destabilize the protein matrix and lower the viscosity, which agrees with the results obtained.
Figure 2B shows the viscoelastic behavior of the beverages, indicating the destruction of the gel network of the product due to the effect of hydrolysis, which means less stability (higher degree of syneresis) for the HFDB beverage. Additionally, the gel behavior (G'>G") for the NHFDB beverage is observed, which gives greater stability to the product.
Sensory analysis
Figure 3 shows the multidimensional sensory analysis for HFDB and NHFDB. The results indicated a slight variation in the odor and acid taste, this is related to the results obtained in the physicochemical characterization where the NHFDB presented a higher acidity, although not significant. Additionally, no significant difference in viscosity was shown, although NHFDB had a higher viscosity because galacto-oligosaccharides are formed due to its hydrolysis (Gómez and Sánchez, 2019). According to Pachekrepapol et al. (2021), these oligosaccharides weaken the gel network by interfering with the protein network, which can cause a decrease in viscosity. Both drinks were rated by the expert judges as high-quality drinks.
Stability Beverage
Fermentation is one of the key operations in yogurt-type fermented dairy beverage technology. When the mixture is inoculated with S. thermophilus and L. bulgaricus bacteria, it begins a lactic acid fermentation where enzymatic hydrolysis of lactose occurs, reaching glucose and galactose monosaccharides as a result and hence the glucose is finally decomposed to lactic acid (Cabrera and Villa, 2020). This production of lactic acid causes a decrease in pH and an increase in acidity, which takes place not only during incubation but also during storage of the fermented dairy beverage because the microorganisms remain viable, although the decrease is less marked due to the effect of low temperature (Figure 4 A and B). Such behavior may be due to the production of galactooligosaccharides formed during enzymatic hydrolysis and cause instability in the gel network formed during fermentation, leading to a higher percentage of syneresis at the end of the storage time.
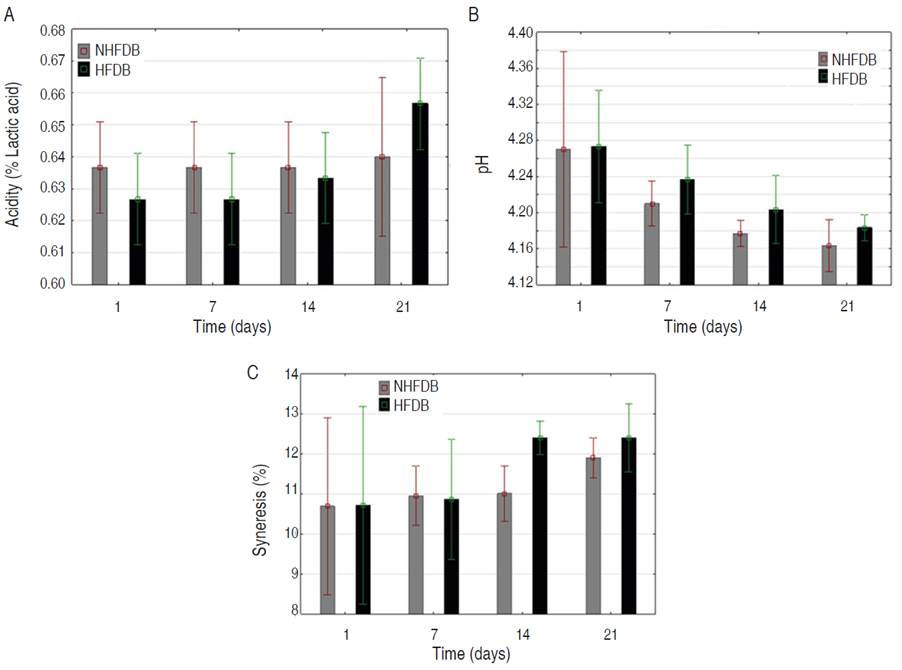
Figure 4 Stability study of fermented dairy beverages (95% confidence intervals for the mean: Fisher LSD Method): A. Acidity; B. pH; C. Syneresis (%).
Figure 4A shows that total acidity tends to increase for both beverages. There are no statistically significant differences between NHFDB and HFDB, but over time there is a significant difference for HFDB between day 1 and day 21 since lactic acid bacteria have the characteristic of producing acids that increase the concentration of H+ in the medium. Different authors comment on the proteolytic activity of Lactobacillus spp, in which amino acids and small acid peptides that induce a reduction in pH are produced, additionally, S. thermophilus generates metabolites such as formic acid and carbon dioxide that also reduce the pH value (Zapata et al., 2015). Other authors have reported this same effect, reaching an acidity of up to 1.44 (% lactic acid) at the end of storage (Hussain et al., 2009; Parra, 2013). According to the NTC 805-2005 standard and the Codex Alimentarius STAN 243-2003, corresponding to fermented milk-yogurt, the acidity value must be at least 0.6% expressed in lactic acid, thus, the titratable acidity values of the different acidity treatments comply with both norms (ICONTEC, 2005; FAO, 2003).
Figure 4B shows the decrease in pH during storage for both beverages. Between the NHFDB and the HFDB, there are no statistically significant differences, but over time there is a statistically significant difference for the HFDB between day 1 and day 21 of storage. This behavior can be attributed to the fact that microbial activity by the lactic acid bacteria present in the fermented dairy beverage occurred during storage under refrigeration conditions (Simijaca et al., 2018; Zhi et al., 2018). Gomes et al. (2017) sweetened yogurt with honey and stored it for 28 days at 4 °C, where the pH decreased over time, relating this behavior to the degradation of lactose into lactic acid. In addition, in both beverages where sucrose was replaced by tagatose, and the pH was found within the acceptable ranges, thus maintaining the microbiological quality. This agrees with works reported by Torrico et al. (2019), who carried out different replacements of tagatose in yogurt, they found that Physicochemical quality associated with titratable acidity, pH, viscosity, and soluble solids was not affected by tagatose replacements in yogurts.
Figure 4C shows a tendency to increase the percentage of syneresis with storage time, which is due to the loss of stability and elimination of water from the components of the fermented dairy beverage due to possible structural modifications in the gel network (Simanca et al., 2013). The above behavior may be due to the production of galactooligosaccharides formed during enzymatic hydrolysis and cause instability in the gel network formed during fermentation, leading to a higher percentage of syneresis at the end of the storage time. Although the maximum level reached in this study was 13%, close to those reported by Lobato et al. (2014) between 9.7 and 23.6, it can be concluded that the gel-like structure formed for HFDB tends to be lower concerning to the NHFDB beverage, which coincides with the viscoelasticity analysis performed. Tamime (2006) reports syneresis values greater than 42%, which according to the author is indicative of loss of structure and high phase separation that deteriorates the physical and techno-functional quality of the product.
CONCLUSION
The use of conjugated systems based on ultrafiltration permeate and protein concentrate of sweet whey and milk makes it possible to obtain fermented dairy beverages with adequate sensory characteristics and physicochemical quality. However, the effect of hydrolysis is notorious in the stability of the emulsions, since the stability of the product is reduced, presenting a higher degree of syneresis for hydrolyzed beverages. Both beverages presented sensory characteristics of a high-quality fermented beverage, with a fresh and milky taste and smell; therefore, it can be stated that tagatose as a sweetener does not affect the sensory perception of these beverages. In relation to stability over time, there is a tendency towards a decrease in the pH value and an increase in acidity and syneresis, the latter being the most noticeable characteristic for HFDB, showing a marked weakness in the gel structure.