1. Introduction
Clays are interesting materials which have been extensively used for a number of industrial applications, for example, they are applicable in the preparation of nanocomposites [1], controlled-release pharmaceutical systems [2,3], catalytic supports [4,5], and other purposes. A category of clays, layered double hydroxides (LDH), are useful materials because it is possible to modulate several of their physical-chemical properties by proper modification of the preparation method and their chemical composition [6-10]. LDH consist of positively charged layers of metallic hydroxides containing water molecules and anions in the interlayer spaces that compensate the charge of the laminae. The positive charge on the layers comes from the substitution of divalent cations by trivalent ones on some octahedral positions, giving rise to structures that are represented according to equation (1):
Where M may be a divalent or trivalent metal, x corresponds to the molar ratio M(II)/(M(II)+M(III)), and An- is the interlayer chemical species with charge n, while m is the hydration extent [11,12]. These materials have successfully been used for immobilization of metallic nanoparticles based on Au, Pt, Ni or Pd [13-15], useful as heterogeneous catalysts for organic reactions such as alcohol oxidations, hydrogenations, carbon-carbon couplings and others [16-18].
Another kind of clays is constituted by metallic hydroxysalts, which are represented by equation (2):
Unlike in LDH, the M in hydroxysalts corresponds to only one kind of metal, but similarly to the LDH structure, metals in hydroxysalts occupy not only octahedral positions (oct) inside the layers but also tetrahedral positions (tet) which are differentiated. About one-fourth of octahedral sites are empty (vac) oct and they are compensated by tetrahedral arrangements up and down in that particular point of the layer. As in LDH, this structural feature gives rise to a charge excess that promotes the intercalation of hosted anionic species in the interlayer space [19,20].
Conventionally, laminar hydroxysalts are solid structures resulting from the reaction of a metal oxide and a salt, promoting in one side the metal species that form the laminar inorganic structure and the interspace anion which confers its stability [19, 21-22]. A particularly useful hydroxysalt is zinc hydroxychloride (Zn5(OH)8Cl2.H2O: simonkolleite: ZHC) [22] which has advantages as a supporting material for catalytic systems in comparison to other frequently used materials, especially regarding their easy preparation and modification. Some authors have reported the synthesis of zinc hydroxychloride by adjusting pH around 6 units by the addition of NaOH [22], and others have prepared hidroxysalts hosting nitrate anion in the interspace, through a two-day reaction of zinc oxide and zinc nitrate at temperatures above 60 ºC, but a shorter methodology to prepare ZHC would be welcome, so we prepared a highly crystalline material in a shorter reaction time.
On the other hand, there are reports in the literature [6-10] about chemical modification of clays by inserting hosting molecules in the interlayer space resulting in materials with modulated properties, especially for catalysts. Taking into account the above, we decided to insert a surfactant into the interlayer space of ZHC to generate lipophilicity inside the material, and then it was exchanged with a palladate complex anion and reduced to metal palladium. It would be expected for this new material to be a promising heterogeneous catalysts for C-C coupling reactions [1,2].
This work illustrates the arrangement of palladium nanoparticles on zinc hydroxychloride which was previously exchanged with a surfactant aiming to take advantage of the wide interspace distances which might positively affect the distribution of palladium nanoparticles. The physical-chemical characterization of the intermediate and target materials and their properties such as morphology, textural analysis, crystallinity and particle size are being presented and discussed as well.
2. Materials and methods
2.1. Starting materials
Analytical grade reagents were used: ZnO (Sigma-Aldrich, 99+%), ZnCl2 (Merck, 98%), CH3(CH2)11OSO3Na (DS) (Carlo Erba, 92%), Na2PdCl4 (Aldrich, 99,99%). Distilled water was deionized previously in our laboratories. All materials were dried at room temperature and atmospheric pressure without the use of drying solvents.
2.2. Physical-chemical characterization of the materials
All materials (ZHC, ZHC-DS, ZHC-DS-PdCl, and ZHC-DS-Pd0) were characterized by X-Ray diffraction (PXRD), Scanning Electronic Microscopy (SEM), Transmission Electronic Microscopy (TEM), surface area and pore distribution were measured at the temperature of liquid nitrogen (-196 ºC).
PXRD analyses were carried out on a Rigaku Miniflex II instrument (30 kV and 15 mA) that uses copper as the radiation source (Cu K() with a scan rate of 2° per minute in normal environmental conditions. The crystal size of Pd in the ZHC- Pd0 was estimated from its diffraction pattern by the Debye-Scherrer´s method [25].
Micrograph images were run on a Scanning Electron Microscope SEM, model QUANTA 250 of FEI, while Transmission Electronic Microscopy TEM analyses were run in a JEOL 1200 EX instrument.
Nitrogen adsorption-desorption isothermals and the textural properties of the materials were determined by nitrogen adsorption on a Micromeritics instrument ASAP 2020, using samples of about 0.100 g. Specific surface areas were estimated from the adsorption isothermals by applying the BET method [26].
2.3. Experimental methods
Precursor preparation (ZHC). Zinc hydroxychloride was obtained in an aqueous phase using the reported procedure [27], but in our case, we did not use an autoclave. ZnO (3.5 g) was added to 100 mL of an aqueous solution containing 136 g of ZnCl2. The resulting suspension was shaken at room temperature under a nitrogen atmosphere for 6 hours, and a white precipitate was obtained by centrifugation, and then it was washed several times with distilled deionized water (DDW). The material was dried at room temperature.
Surfactant-modified, ZHC-DS . To a 100 mL of a 0.05M solution of sodium dodecyl sulfate, 1.3 g of ZHC was added. The entire suspension was shaken at room temperature for 22 hours. A white precipitate was obtained by filtration, and then it was washed several times with distilled deionized water (DDW) and dried at room temperature.
Tetrachloropalladate-exchanged, ZHC-DS-PdCl . The surfactant in ZHC-DS was exchanged with the tetrachloropalladate anion. 1.5 g of Na2PdCl4 was added to 50 mL of an aqueous suspension containing 0.3 g of ZHC-DS. The resulting suspension was shaken at room temperature for 5 hours, and a brown precipitate was obtained by filtration. Then, it was washed several times with distilled deionized water (DDW) and dried at room temperature for 12 hours.
Nanoparticles of Pd in surfactant-modified, ZHC-DS-Pd0 . The target material was obtained by reduction of ZHC-DS-PdCl. 72 mg of ZHC-DS-PdCl were suspended in 5.0 mL of CH3CH2OH and shaken for 2 hours at room temperature. A black precipitate was obtained by centrifugation and it was washed seven times with 3.0 mL of CH3CH2OH each.
3. Results and discussion
Zinc oxide and zinc chloride were combined to form the respective ZHC, a white material that was used as the starting precursor to assemble the palladium nanoparticles inside the ZHC. The target material was obtained through a step-by-step sequence that involved: (1) synthesis of the hydroxysalt (ZHC) according to the standard procedure [19]; (2) intercalation of dodecyl sulfate anion in the interlayer space of the ZHC precursor (ZHC-DS); (3) exchange of dodecyl sulfate by tetrachloropalladate anion (ZHC-DS-PdCl); (4) reduction of the palladium species retained inside the ZHC to be converted into metallic palladium nanoparticles ZHC-DS-Pd 0 . Visible qualitative changes in the appearance of the materials upon going successively from precursors to intermediates and to the final product are strong suggestions that modifications were occurring properly, and such transformations were confirmed by instrumental analysis as discussed ahead. Our first piece of evidence comes from X-ray diffraction (PXRD patterns) of the materials (Fig. 1), exhibiting the typical lines corresponding to laminar structures.
For the ZHC precursor, Fig. 1a suggests the presence of the expected typical pattern of this kind of materials [28] and shows the crystallographic planes (003), (006), (110) and (0012) corresponding to 2Ө values of 11.2, 22.5, 28.1 and 46.0° respectively, and a basal spacing of 7.8 Å. Fig. 1b-1d exhibits substantial differences of the surfactant-modified hydroxysalt (ZHC-DS, Fig. 1b) and the subsequent materials (ZHC-DS-PdCl, Fig. 1c, and ZHC-DS-Pd0, Fig. 1d) with respect to the precursor ZHC (Fig. 1a). The disappearing of the characteristic zinc hydroxychloride peaks evidences the transformation of the laminar phase into a new one, with the surfactant molecules being intercalated in the interlayer space, giving rise to an ordered bilayer structure, where the longitudinal axis of the surfactant molecules turns out to be approximately perpendicular to the precursor laminae [29]. In Fig. 1b, two peaks at low 2Ө values are observed, which correspond to two phases with d-spacing of 32.3 Å and 26.2 Å for the main phase. The first value closely resembles the reported data of 31.5 Å for a nitrate-based hydroxysalt that was exchanged with DS in methanol in a theoretical and experimental study [29], which concluded that external variables such as solvent and temperature of the system exert some influence on the structural arrangement of surfactant in the interlayer space. According to it, we believe that in our case the presence of the second phase which is absent in the materials studied by Demel et al. [29], may be the result of the use of water instead of methanol as the solvent. In turn, as observed in Fig. 1c for ZHC-DS-PdCl, as a consequence of the intercalation of a PdCl4 -2 anion in the interlayer space, a decreased basal spacing is observed. As such, the surfactant-containing material (ZHC-DS) with original basal spacing’s of 32.3 Å and 26.2 Å is changed for a material (ZHC-DS-PdCl) with a lowered basal spacing of 25.6 Å and 22.7 Å for the primary phase (Fig. 1c). These data are consistent with an expected diminution in the content of surfactant that induces a bilayer structural change in such way that a decrease in the basal spacing takes place, as reported [29].
Finally, in this sequence, Fig. 1d corresponds to the PXRD pattern of the material resulting from the reduction of the PdCl4 -2 anion exchanged ZHC to form palladium nanoparticles (this final material was labeled ZHC-DS-Pd0). Comparing Fig. 1c and 1d, there are substantial changes in the PXRD pattern and morphology (as shown later in Fig. 3d) of the laminar material upon reduction. As expected, palladium is reduced to metallic palladium with a consequent loss of the structural ordering which is due to the size and distribution of the resulting nanoparticles and the subsequent delamination of the ZHC-DS-PdCl material, so that the characteristic peaks of the clay are not visible anymore.
According to a previous report [21,30], palladium nanoparticles formation is suggested by the diffraction peak at about 40º in 2Ө, which is consistent with our result, and it was also confirmed by TEM images (see ahead in Fig. 3e and 3f). Based on this PXRD peak, palladium nanoparticles size was estimated in about 4 nm by using the Debye-Scherrer´s method [25].
Our second piece of evidence of structural changes from precursors to intermediates and to the final material comes from adsorption-desorption isothermals at liquid N2 temperature (Fig. 2) while the respective analysis of the physical chemical features is summarized in Table 1.
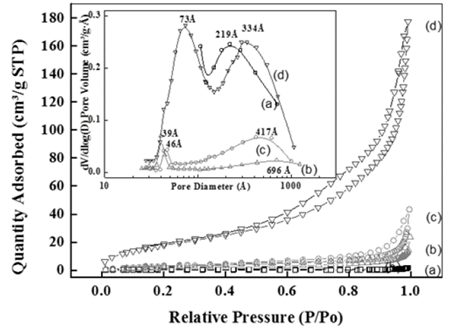
Source: The authors
Figure 2 N2 adsorption-desorption isotermals for (a) ZHC, (b) ZHC-DS, (c) ZHC-DS-PdCl, (d) ZHC-DS -Pd0. Inset. Mesopore distribution by BJH method for (a) ZHC, (b) ZHC-DS (c) ZHC-DS-PdCl, (d) ZHC-DS-Pd0.
According to IUPAC classification, these are isothermals type II (Fig. 2), typical of materials with big pores, characterized by hysteresis type H2 and H3 of materials with slit-shaped pores. The surfactant-modified hydroxysalt (ZHC-DS) register a slight adsorption enhancement, likely because of the electrostatic attraction between the sulfate groups of surfactant molecules and the positive charges of the ZHC, and also because of the hydrophobic interactions of the surfactant, as other authors have reported [31].
It is important to note that ZHC pore diameter distribution is characterized by a heterogeneous distribution with diameters centered mainly at 219 Å (Fig. 2a), while the surfactant-modified ZHC (ZHC-DS) (Fig. 2b) exhibits a significant diameter decrease (39 Å), probably because the insertion of surfactant forms mesopores. Upon exchange with the PdCl4 -2 anions, pore diameters of the resulting material (ZHC-DS-PdCl, Fig. 2c) increase again due to a possible reconfiguration of the interlayer space as explained before. After the reduction to form a palladium-containing surfactant-modified ZHC (ZHC-DS-Pd0, Fig. 2d), a heterogeneous pore diameter distribution is observed. In Fig. 2d, the first peak suggests the presence of 73 Å-mesopores, and the second peak indicates the occurrence of pores diameters centered at around 334 Å. The first maximum might be the result of palladium nanoparticles being incorporated possibly in the interlayer space, while the second one indicates an increase of the mesopore diameters that are formed in the material due to the incorporation of bigger palladium nanoparticles and mesopores that could be formed between the particles of the material.
According to the trends observed in Table 1, the precursor material ZHC is characterized by a low BET surface area (1.07 m2g-1) which is significantly increased to 11.6 m2g-1 when DS is intercalated into the material, and is in turn diminished back to 6.79 m2g-1 when the PdCl4 -2 anion entered into the material. This latter effect might be the result of a reconfiguration of the interlayer surfactant molecules because of the insertion of the PdCl4 -2 in some of the pores, in agreement with a previous literature report [32]. Furthermore, by comparison of BET surface areas for these materials, a drastic increase from 6.79 m2g-1 (in ZHC-DS-PdCl) to 70.11 m2g-1 (in ZHC-DS-Pd0) was observed, and this should be the result of the formation of palladium nanoparticles that generate mesopores with the consequent substantial increase of the pore volume, which finally results in the loss of the ordered structure in the material (consistent with Fig. 1d).
To study the morphology of the materials, SEM analyses were run for each of them, and the respective micrographies are shown in Fig. 3.
The first image corresponds to the initial hydroxysalt (ZHC), where a crystalline material is observed, and some octahedral crystals with sizes of around 2-3 μm can be distinguished (Fig. 3a). In contrast, the morphology of the surfactant-modified hydroxysalt (ZHC-DS) is characterized by platelets of irregular thickness and irregular aggregates of flakes (Fig. 3b), which suggest the presence of two phases. This drastic change in the morphology of the materials illustrates that a DS intercalation effectively took place.
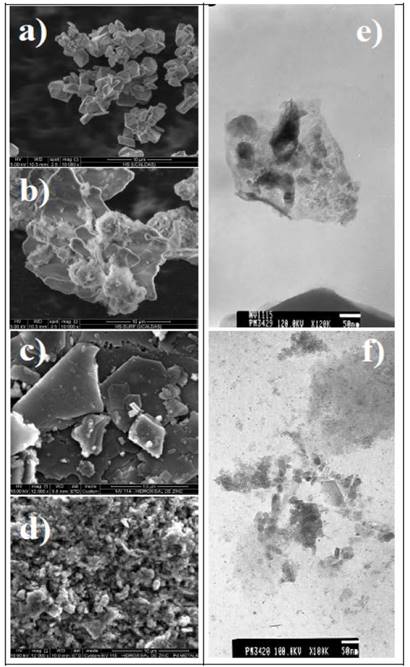
Source: The authors
Figure 3 SEM micrographies: (a) ZHC (10.000x), (b) ZHC-DS (10.000x), (c) ZHC-DS-PdCl (12.000x), (d) ZHC-DS-Pd0 (12.000x). (e) and (f) TEM micrographies of the reduced material ZHC-DS-Pd0.
On the other hand, and according to Fig. 3c, after intercalation of the PdCl4 -2 anions, irregularly-shaped laminae are visualized in the resulting material ZHC-DS-PdCl, which upon treatment with refluxing ethanol, changes its morphology to clusters of sub-micrometric particles (see Fig. 3d for ZHC-DS-Pd0). Additionally, TEM images were run for the material containing reduced palladium (ZHC-DS-Pd0), Fig. 3e and 3f, where it is possible to differentiate particles with sizes varying approximately from 4-7 nm up to bigger aggregates and even particles of 100 nm in size. Both, TEM images (Fig. 3e and 3f) and PXRD patterns (Fig. 1d) confirm the successful generation of metal palladium particles with nanometric sizes. These particles are characterized by irregular shapes and are heterogeneously dispersed throughout the material.
4. Conclusions
The physical chemical characterization of materials proved that changes were occurring from the structural, textural and morphological point of view, upon going step by step from the ZHC precursor to intermediates and to the final material. PXRD patterns confirm that an intercalation of surfactant into the interlayer space of the hydroxysalt took place, giving rise to an interstratified material (32.3 Å and 26.3 Å). Then, the exchange reaction with a PdCl4 -2 anion promotes a decrease in the content of surfactant, which was evidenced by the PXRD analysis (basal spacing changes to 25.7 Å and 22.7 Å). Subsequently, the respective reduced material is constituted by palladium nanoparticles into the surfactant-containing material, and this fact was confirmed by both their PXRD patterns and TEM images.
The possibility of a surfactant-guided preparation of palladium nanoparticles into a hydroxysalt has been demonstrated. The significant increase of BET surface area of the reduced material is closely related to the palladium nanoparticles formation and the laminar material exfoliation. The adsorption of surfactant into a hydroxysalt makes possible to introduce a slight lipophilic character into this highly hydrophilic material, which may be taken advantage of in organic reactions, as suggested by Liu et al [33] who reported that a catalyzed Suzuki C-C coupling reaction with palladium nanoparticles supported on a LDH was favored by intercalation of dodecyl sulfate anion.
In order to have some idea about the likely catalytic potential of the new material, a preliminary catalytic test with our reduced material in a Suzuki C-C coupling reaction was performed, starting from iodobenzene and phenylboronic acid, which gave rise to about 90% yield of a white solid, which was not spectroscopically characterized but exhibited identical physical properties than an authentic biphenyl sample.