1. Introduction
Brazil is the largest sugarcane producer in the world. It annually produces more than 700 million tons, representing around 40% of world production, followed only by India and China with 18 and 7%, respectively [1]. In Brazil, a large part of the sugarcane is destined for ethanol production, which generates sales of around US$ 10 billion a year and places this product among the 10 most important for the Brazilian economy. Only in 2015, Brazil produced 27.5 billion liters of ethanol, with special remark on the state of São Paulo, for producing 50% of this amount [2].
It is estimated that each liter of ethanol production generates between 9 and 14 liters of vinasse, the main wastewater from sugarcane processing. Because of the large volumes generated, their disposal and destination is one of the biggest challenges involved in ethanol production. Nowadays, the main use of vinasse is in the fertirrigation of the sugarcane crop, as a substitution of part of the chemical fertilization. However, its application in high doses can lead to soil salinization [3] and surface and/or groundwater pollution [4].
Several researches have focused on obtaining options for the vinasse remediation. Among them, microalgae cultivation is a promising alternative, since the produced biomass during the treatment can be feedstock for biofuel and bio-based chemical production. Among the species of microalgae studied for this purpose, the Chlorophyceae Desmodesmus subspicatus stands out for its high productivity observed in the distillery effluent [5]. Its cultivation could be potentiated by reducing the vinasse characteristic turbidity, allowing suitable incidence of luminosity to the photosynthetic cells, therefore promoting mixotrophic growth [6].
A promising option for vinasse clarification may be electrocoagulation (EC), due to its efficiency in suspended material removal from wastewaters with high content of dissolved salts, such as vinasse, over a wide pH range, without prior dilution or addition of chemical reagents [7]. Electrocoagulation is based on the simultaneous in situ generation of coagulant chemical species, gaseous hydrogen and hydroxyl ions by the electrolytic oxidation of a sacrificial anode and the decomposition of water at the cathode surface, triggered by the application of an electrical potential difference between the electrodes [8].
In this context, the present research evaluated the cultivation of the green microalgae D. subspicatus in sugarcane vinasse previously clarified by electrocoagulation with aluminum or iron electrodes, aiming at effluent remediation and microalgae biomass production.
2. Materials and methods
2.1. Distillery effluent
The vinasse used in the study was collected in September 2017 directly from the distillation columns of sugarcane processing plant in Araras city, São Paulo state, Brazil, in 20 L plastic containers and immediately taken to the Laboratory of Applied Microbiology and Control (LABMAC) of Federal University of São Carlos (UFSCar), campus Araras. At the site, the samples were fractionated in 2 L plastic containers and frozen (-20 °C) until use.
2.2. Electrocoagulation
It was used a batch-type electrolytic reactor (Fig. 1) with the following parts: (1) Direct current power supply (0-30 V, 0-15 A); (2) 3 L plastic electrolytic cell containing 2.8 L of vinasse; (3) metal electrodes in parallel arrangement, 3 cathodes and 3 anodes, with a total anode area of 300 cm2 and cathode-anode distance of 2 cm; (4) magnetic stirrer. The electrocoagulation was done in triplicate with aluminum or iron electrodes for 5 hours, constant current of 6 A (current density = 20 mA cm-2, ratio anode area: volume = 0.1 cm -1), automatic electrode polarity inversion in intervals of 15 min and magnetic stirring of 450 rpm, as used in similar studies [9,10].
EC samples were taken in one-hour intervals. Then, they were left to settle for 24 hours. The supernatants were collected for turbidity, pH, total organic carbon (TOC) and total nitrogen (TN) determination. Removal and remaining percentage of each parameter were calculated from the raw vinasse turbidity, TOC and TN. The theoretical electrode consumption (Celectrode), equivalent to the dosage of Al+3 or Fe+2, was calculated with eq. (1) and expressed as g L-1. The electric energy consumption (Cenergy) expressed as kWh m-3 was calculated with eq. (2). The approximate EC operating cost expressed as US$ m-3 was calculated with eq. (3), considering just electrodes and electric energy consumption.
Where: i = current passing through the electrodes expressed in A; t = electrolysis time expressed in h; M = molar mass of the chemical species (27 g mol-1 for aluminum and 55.8 g mol-1 for iron); n = electric charge of the chemical species (3 for aluminum and 2 for iron); F = Faraday constant (96500 C mol-1); V(t) = voltage in the electrodes at a time t; Vol = effluent volume, expressed in L; a = cost of the metal, expressed as US$ kg-1; b = cost of electric energy, expressed as US$ kWh-1.
After selection of an optimum vinasse electrocoagulation time using turbidity removal criterion, the supernatants of EC pretreatment with aluminum and iron electrodes were used as culture mediums for D. subspicatus microalgae, without any dilution or sterilization.
The results of the pretreatment by electrocoagulation were compared with the following control pretreatment: raw vinasse pH adjustment with 10 M NaOH until pH 7.5 and subsequent centrifugation for 5 minutes at 1844xg and 25 °C. The supernatant was characterized (control pretreatment) and used without dilution or sterilization as culture medium for D. subspicatus.
2.3. Microalgae cultivation
D. subspicatus strain was obtained from LABMAC-UFSCar microalgae bank and propagated in modified BG11 medium [11] until approximately 0.5 g L-1 dry biomass concentration. Subsequently, a sufficient volume of inoculum was centrifuged at 1844xg for 20 minutes, the supernatant discarded and the sediment resuspended in vinasse pretreated by electrocoagulation with iron or aluminum electrodes, or in centrifuged vinasse (control pretreatment) to begin the cultivation with 0.3 g L-1 of microalgal dry biomass. Cultures in each medium were performed in triplicate in an orbital shaker (100 rpm, 25 °C) for 48 hours, with photoperiod of 12 hours and light intensity of approximately 2 klux. Samples were taken at intervals of 3, 6, 12, 18, 14 and 48 hours for TOC, TN, pH and dry biomass determination.
The microalgae maximum specific growth rate (μmax) expressed as h-1 was calculated by linear regression in the exponential growth phase (eq. 4). The biomass productivity (Pbiomass) expressed as g L-1day-1 was calculated according to eq. (5), considering the cultivation total time.
Where: X1 and X2 correspond to dry biomass at the beginning and at the end of the exponential phase, respectively, expressed in g L-1; t1 and t2 correspond to the initial and final time of the exponential phase, respectively, expressed in h; Xo and Xf correspond to dry biomass at the beginning and at the end of the cultivation, respectively, expressed in g L-1; tf = cultivation total time, expressed in h.
2.4. Analytical procedure
The physical-chemical characterization of the raw and treated vinasse was done according to the methodology of the Standard Methods [12]. Table 1 shows the analytical techniques used to determine each parameter. The results were presented as the mean value ± standard deviation. Statistical analyses (ANOVA and Tukey test p < 0.05) were performed using software R version 3.4.0. The removal percentages of each physical-chemical parameter were calculated considering the raw vinasse initial parameters.
Table 1 Physical-chemical parameters and analytical methods
Parameter | Analytical method | Units |
Turbidity | Nephelometry | NTU |
Suspended solids | Gravimetry after 0.45 μm membrane filtration | mg L-1 |
pH | Potentiometry | - |
Total organic carbon (TOC) | High temperature combustion | mg L-1 |
Total nitrogen (TN) | Combustion and chemiluminescence | mg L-1 |
Dry biomass | Gravimetry after 0.45 μm membrane filtration | g L-1 |
Source: The Authors.
3. Results and discussion
3.1. Global process
Table 2 presents the physical-chemical characterization of the raw vinasse and after each stage of the different treatments. EC process with aluminum electrodes and subsequent cultivation of D. subspicatus removes greater amount of TOC and TN from the distillery effluent (66% and 75%, respectively) than the iron electrode EC process and cultivation (removal of 55% of TOC and 63% of TN). Both processes reach higher removals of TOC and TN than the control process of centrifugation and subsequent cultivation (removal of 49% and 56%, respectively).
Table 2 Physical-chemical characterization of the raw vinasse and after each stage of the different treatments
Treatment | Parameter | ||||
---|---|---|---|---|---|
Turbidity (NTU) | Suspended solids (mg L-1) | pH | TOC (mg L-1) | TN (mg L-1) | |
Raw vinasse | 1314 | 5451 | 4.3 | 10205 | 584 |
Centrifugation1 | 142 | 1120 | 3 7.3 | 9763 | 533 |
Centrifugation and cultivation2 | - | - | 8.3 | 5172 | 255 |
Aluminum EC | 27 | 247 | 6.4 | 5676 | 251 |
Aluminum EC and cultivation | - | - | 8.1 | 3459 | 141 |
Iron EC | 25 | 253 | 6.2 | 6848 | 305 |
Iron EC and cultivation | - | - | 5.0 | 4648 | 214 |
1 Control pretreatment
2 Control process.
3 Adjusted by NaOH addition.
Source: The Authors.
According to Fig. 2, the D. subspicatus cultivation in the control pretreatment and in the aluminum EC pretreatment obtained similar microalgal biomass productivities (around of 1.5 g L-1day-1). These results are interesting mainly in terms of process scale-up, taking into account that vinasse clarification by centrifugation is generally limited at laboratory scale, while electrocoagulation is a scaling-up technology to treat larger effluent volumes [8].
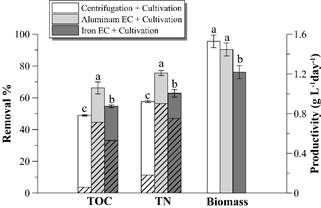
Source: The Authors.
Figure 2 TOC and TN removal percentages and biomass productivity achieved by the different treatments. The hatched bar portion represents the TOC and TN removal percentage by each pretreatment. Different letters above bars represent statistically different values (p < 0.05). Error bars represent the standard deviation (n = 3).
The results of EC processes with subsequent cultivation of D. subspicatus are similar to those obtained by [13], in the study integrating the cultivation of Chlorella vulgaris with electrocoagulation pretreatment with iron electrodes. The authors reported the global removal of 77% of the chemical oxygen demand (COD) and 88% of TN from an anaerobic digestion liquid effluent from a mixture of dairy manure and food wastes. The results of the present study are promising since show the possibility of replicating the EC method and microalgae culture in the sugarcane effluent.
3.2. Electrocoagulation
Fig. 3 shows the turbidity, suspended solids, TOC and TN removal percentage from raw vinasse according to the different treatments. Electrocoagulation with both electrode materials removes larger percentages of the mentioned parameters than centrifugation.
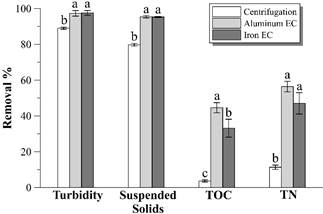
Source: The Authors.
Figure 3 Turbidity, suspended solids, TOC and TN removal percentages achieved by the different treatments. Different letters above bars represent statistically different values (p < 0.05). Error bars represent the standard deviation (n = 3).
Fig. 4 shows the remaining turbidity profiles in the supernatant after each hour of EC. There is a turbidity removal of more than 98% with both electrode materials, reaching values less than 30 NTU after 4 hours of EC with aluminum electrodes and after 5 hours with iron electrodes. Such result is more promising than the presented by [14], but similar to that observed by [9], both studies in distillery effluent using aluminum electrodes. High levels of clarification can also be observed with both materials in electrocoagulation of baker’s yeast wastewater [15] or winery effluent [16].
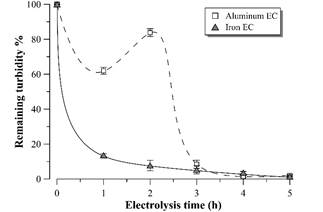
Source: The Authors.
Figure 4 Remaining turbidity percentage profile during vinasse electrocoagulation. Error bars represent the standard deviation (n = 3).
In the case of aluminum EC, a turbidity increase is observed in the second hour of electrolysis, reaching the minimum value after 4 hours. This behavior was analyzed by [17] and could be ascribed to the aggregates particle size during the EC according to the pH and concentration of the coagulant species. However, this behavior was not observed in EC with iron electrodes in the time intervals analyzed. In this case, the turbidity gradually decreased until reaching a value similar to EC with aluminum electrodes after 5 hours.
In Fig. 5 is shown the appearance of supernatants every hour of EC. It is observed an intense coloration in the first two hours of EC with iron electrodes, probably due to the presence of the Fe (II) compounds generated by the EC, favored by the initial acidic pH of the vinasse [10].
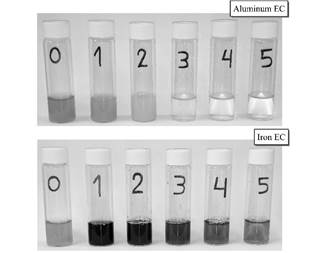
Source: The Authors.
Figure 5 Appearance of supernatants during vinasse electrocoagulation. Each number represent the time (h) of electrolysis.
Figs. 6, 7 show the remaining percentage of TOC and TN in the supernatant and accumulated electric consumption after each hour of EC with aluminum and iron electrodes, respectively. There is a stability tendency in the remaining percentage of TOC and TN in the supernatant after 3 hours of EC with both materials. The EC with aluminum electrodes is more efficient in TOC removal from the distillery effluent than EC with iron electrodes. Similar results were observed by [10] with the same effluent and by [18] with biodigested molasses process wastewater. However, different results were observed in other effluents, such as in baker’s yeast wastewater [15]. The difference can be attributed to the different physical-chemical nature of the aforementioned effluents [19].
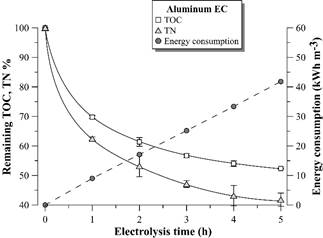
Source: The Authors.
Figure 6 Remaining TOC and TN percentage and electric energy consumption during vinasse EC with aluminum electrodes. Error bars represent the standard deviation (n = 3).
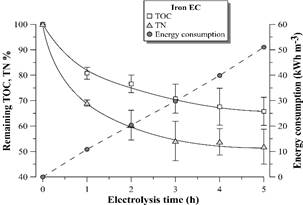
Source: The Authors.
Figure 7 Remaining TOC and TN percentage and electric energy consumption during vinasse EC with iron electrodes. Error bars represent the standard deviation (n = 3).
The electrical energy consumption profile according to time was linear in the 5 hours of EC with both materials, since there was no considerable voltage variation in the electrodes during the experiment (4.0 ± 0.3 V for EC with aluminum electrodes and 4.9 ± 0.5 V for EC with iron electrodes). These results suggest negligible electrode passivation during electrolysis.
In the study, the maximum TOC removal from vinasse was around 45 and 30% by EC with aluminum and iron electrodes, respectively. Despite these results are lower than the reported in the literature for the same effluent [9,10], they are promising considering that after 4-5 hours of EC treatment, there is a highly clarified effluent containing around 5500-6500 mg L-1 of TOC for reclaiming via microalgae cultivation. It is still observed 40-50% of TN remaining in the vinasse, which maintains carbon: nitrogen C/N ratio around 20, the original value of the distillery wastewater.
The supernatant pH value tends to stabilize around 6.0-6.5 for both materials (Fig. 8) during EC process time. In general, electrocoagulation tends to neutralize the pH of acidic effluents due to the production of OH-1 ions at the cathode [20], which is an advantage of the EC process compared to chemical coagulation [21]. It should be noted that the neutralization of the vinasse after EC allows the cultivation of D. subspicatus without the need of previous pH correction of the medium, once the pH after EC with both electrode materials approaches the pH of the BG11 standard medium [11].
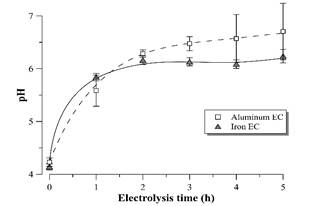
Source: The Authors.
Figure 8 pH profile during vinasse EC with aluminum and iron electrodes. Error bars represent the standard deviation (n = 3).
Considering the EC results showed before, the cultivation of D. subspicatus was done in the supernatants of the EC after 4 hours with aluminum electrodes and after 5 hours with iron electrodes. Table 3 presents an estimate operating cost of the EC treatments in this study and the theoretical electrode consumption, equivalent to the dosage of Al+3 or Fe+2 according to electrode material.
Table 3 Vinasse electrocoagulation operating cost.
Aluminum EC | Iron EC | |
Electrolysis time (h) | 4 | 5 |
Electrode consumption per volume (g L-1) | 2.9 | 11.2 |
Electric energy consumption (kWh m-3) | 33.4 | 51.0 |
Operating cost 1,2 (US$ m-3) | 9.95 | 17.60 |
1 Electric energy cost in Brazil: 0.15 US$ kWh-1 [23].
2 Aluminum metal cost: 1.70 US$ kg-1; Iron metal cost: 0.89 US$ kg-1 [24].
Source: The Authors.
To the authors' knowledge, there are no reported values of estimated cost, electrode nor energy consumption for the raw vinasse electrocoagulation treatment. According to [22], the estimated cost of the chemical coagulation of biodigested vinasse is 1.55 US$ m-3, considering only the coagulant cost (0.55 US$ kg-1 of FeCl3 38% w/w). Thus, electrocoagulation could be a lower-cost alternative for raw vinasse treatment than Fenton advanced oxidative process (23.50 US$ m-3 reported by [22]), considering similar TOC efficiency removal from the biodigested vinasse.
3.3. Microalgae cultivation
Figs. 9-11 show the growth curves of green microalgae D. subspicatus in vinasse pretreated by centrifugation (control) and by EC with aluminum and iron electrodes, respectively. The results indicate similar growth profiles, with maximum specific growth rate of around 0.09 h-1 for both materials and the control (Table 4). However, the biomass productivity was lower in vinasse pretreated by iron EC (1.22 g L-1day-1) than in other pretreatments (around 1.5 g L-1day-1), which indicates a possible interference of the remaining iron compounds in the medium, limiting the development of the microalga, at least in the first hours of cultivation.
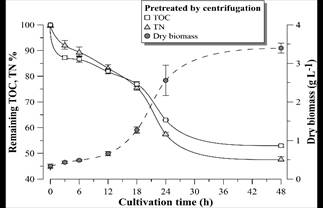
Source: The Authors.
Figure 9 Remaining TOC and TN percentage and D. subspicatus growth curve during cultivation in vinasse pretreated by centrifugation. Error bars represent the standard deviation (n = 3).
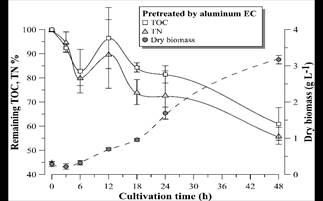
Source: The Authors.
Figure 10 Remaining TOC and TN percentage and D. subspicatus growth curve during cultivation in vinasse pretreated by EC with aluminum electrodes. Error bars represent the standard deviation (n = 3).
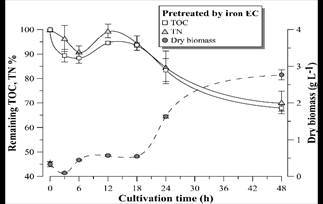
Source: The Authors.
Figure 11 Remaining TOC and TN percentage and D. subspicatus growth curve during cultivation in vinasse pretreated by EC with iron electrodes. Error bars represent the standard deviation (n = 3).
Table 4 D. subspicatus growth kinetic parameters
Pretreatment | |||
Centrifugation (Control) | Aluminum EC | Iron EC | |
µmax (h-1) | 0.085 (R2 = 0.96) | 0.095 (R2 = 0.98) | 0.103 (R2 = 0.76) |
Biomass productivity (g L-1day-1) | 1.53 ± 0.06 a | 1.45 ± 0.07 a | 1.22 ± 0.07 b |
Different letters represent statistically different values (p < 0.05).
Source: The Authors
The kinetic data for both pretreatments and the control are higher than those obtained by [13] with C. vulgaris cultivation in dairy manure and food wastes biodigested effluent pretreated by EC with iron electrodes (0.04 h-1 and 0.22 g L-1day-1). These results indicate the potential of vinasse as microalgae culture medium, probably ascribed to the balanced C/N ratio of the sugarcane effluent (around 20), even after pretreatment, which is suitable for microalgae development [5].
Several studies of microalgae cultivation in sugarcane vinasse can be found in the literature. Maximum specific growth rate of 0.04 h-1 was recorded for Spirulina maxima heterotrophic culture in filtered vinasse 1% v/v [25]. Biomass productivity of 0.16 and 0.22 g L-1day-1 in mixotrophic culture of Micractinium sp. and Chlamydomonas biconvexa, respectively, were obtained by [26] in clarified vinasse with Ca(OH)2 without dilution.
Maximum specific growth rates of 0.06 h-1 were obtained in the mixotrophic culture of C. vulgaris in 60% v/v filtered vinasse and in 80% v/v biodigested vinasse [27]. Maximum specific growth rate of 0.04 h-1 and biomass productivity of 0.13 g L-1day-1 were reported by [28] in the mixotrophic culture of Micractinium sp. in 10% v/v diluted distillery effluent. Those results suggest D. subspicatus growth potential, since the kinetic parameters of its cultivation in clarified vinasse by the methods studied in this work were higher.
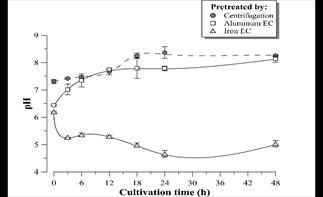
Source: The Authors.
Figure 12 pH profile during D. subspicatus cultivation in vinasse pretreated by centrifugation (control) and EC with aluminum and iron electrodes. Error bars represent the standard deviation (n = 3).
D. subspicatus cultivation achieved maximum removal of respectively 39 and 44% of the TOC and TN of the vinasse pretreated by EC with aluminum electrodes and 32 and 30% of the TOC and TN of the vinasse pretreated by EC with iron electrodes. These results are less than the TOC and TN removal observed in the control (cultivation in vinasse pretreated by centrifugation), where the microalgae consumed 47 and 52% of the medium TOC and TN, respectively. Maximum removal of 36% of COD and 52% of TN was reported by [5] in heterotrophic culture of D. subspicatus in the same effluent, also centrifuged. It could be concluded that the clarification of the sugarcane vinasse promoted the microalgae mixotrophic metabolism, since less consumption of organic carbon, maintaining the same growth rate, suggest CO2 fixation as complementary carbon font for growth support.
Fig. 12 shows an alkalization trend of the medium during the D. subspicatus batch-time cultivation in centrifuged vinasse (control) and in vinasse pretreated by EC with aluminum electrodes. This tendency began after 24 hours in the case of vinasse pretreated by iron EC. It is known that the alkalization of the liquid medium is related to microalgae photosynthesis [29], therefore could be concluded autotrophic metabolism and simultaneous consumption of organic carbon sources (mixotrophic metabolism). The pH decrease in the first hours of microalgae cultivation in vinasse pretreated by iron EC might be related to the oxidation of Fe (II) remaining in the medium to Fe (III) species catalyzed by the presence of light or dissolved oxygen during the culture. The study of such phenomenon will be deepened in following works.
4. Conclusions
The results indicate the viability of the integrated electrocoagulation and green microalgae D. subspicatus cultivation, obtaining simultaneously microalgal biomass production and high degree of raw vinasse remediation. Electrocoagulation with aluminum and iron electrodes removed more than 98% of the initial raw vinasse turbidity, without dilution or previous pH correction. EC pretreatment allowed the subsequent culture of microalgae, since electrocoagulation raised the acidic pH of the distillery wastewater to values close to the pH of standard BG11 medium. Aluminum EC and subsequent microalgae cultivation removed 66 and 75% of initial total organic carbon and total nitrogen, respectively, with biomass productivity of 1.45 g L-1day-1 and maximum specific growth rate of 0.095 h-1. Microalgae productivity was inferior in vinasse pretreated by iron EC, suggesting possible interference of ferric compounds in the microalgae development. Further studies should aim to minimize the treatment energy consumption and to maximize effluent remediation and biomass productivity, in order to offer a new sustainable alternative for sugarcane vinasse treatment.