1. Introduction
Base isolation (BI) is a technique of seismic protection of structures to reduce their seismic risk. In this technique, the structural base is uncoupled from the superstructure through devices called isolators, which are significantly flexible in the horizontal direction but highly rigid in the vertical one. The primary objective is to reduce the earthquake energy input to the superstructure, increasing two essential structural parameters: flexibility and damping. Traditional isolation systems are composed of either elastomeric bearings or pendulum friction devices. The selection of one type or the other depends on technical and economic criteria.
BI is widely accepted due to its excellent behavior in isolated buildings and bridges exposed to several earthquakes [1-4]. As a result, they suffered minimum damage regarding the damage of fixed base infrastructure, reducing its seismic risk. Around the world, more than 7000 buildings [5] have been equipped with seismic isolation. Colombia’s implementation of base isolation is recent, with approximately 30 buildings and 20 bridges [6]. In the last decades, the topic has been paid attention to in the country, as evidenced in the considerable number of research presented during the X Colombian Conference on Earthquake engineering in July of 2022 [7]. However, it is essential to note that the technique has some limitations where, theoretically, its efficiency is reduced, such as in tall buildings and soft soil conditions.
Although the technique’s benefits for buildings and bridges are known, the use of seismic isolation for other structural types has been little explored or unexplored in Colombia. In different countries, it is possible to find other types of isolated structures such as Liquefied Natural Gas (LNG) tanks [8], water tanks, fuel tanks [5,9], cultural heritage structures [10], monumental structures [11,12], communication centers [13], nuclear power plants [14], offshore platforms [15], boilers, racks, roofs, stadiums [16] and some structures that need to reduce vibration transfer from the subsoil [17]. The mentioned cases show the ability of BI to improve seismic performance for a range of structural systems. However, the decision to use the technology depends on the structural engineer.
Sports stadiums in Colombia are non-conventional structure that could be equipped with isolator devices to improve their seismic performance. Examples of isolated stadiums around the world include the Sofi stadium in Los Angeles (USA), Ariake Arena in Tokyo (Japan), and the Philippine Area in Bulacan (Philippines). To the authors’ knowledge, no Colombia stadium has been seismically isolated. By applying BI in stadiums, it would be possible to mitigate the seismic risk due to the following aspects:
The decrement in the acceleration of the structure will allow evacuating people from the stadium more safely in the stadium during an earthquake.
The stadium could be destined for a humanitarian shelter if kept structurally safe after the earthquake.
Less damage in the stadium implies the protection of the significant investments made by the public or private sectors for the construction and operation of these specific structures.
Fixed-base structural configurations in stadiums present unfavorable vertical configurations (irregularities) and sloping beams that affect their seismic behavior [18].
This research deals with the design of the seismic isolation system for a soccer stadium grandstand with the aims of a) showing the possible structural benefits of this type of infrastructure located in Colombia, taking as reference the behavior of the fixed base grandstand, b) evaluating the approximate cost of implementing the technique (related to materials), and c) verifying the hypothesis of limitations in BI’s application on typical soft soil in the country. First, in Section 2, the paper presents the methodology for the structural design of the stadium grandstand with and without seismic isolation. Then, the results of the obtained design are reported in Section 3. Finally, the main conclusions of this research are reported in Section 4.
2. Methodology
2.1. General considerations
In this research, the stadium grandstand is considered for design purposes with two types of foundations: a) a fixed base and b) a seismic-isolated base. The type of BI used for the isolation system corresponds to a high-damping rubber bearing (HDRB). HDRBs have high damping values due mainly to their lead core and present high flexibility and recentering capacity. It is assumed that the structure is located in Bogota (Colombia), a city with an intermediate seismic hazard level but a high population and a notable presence of soft soils. In Bogota, a building with BI has been recently built.
The seismic zonation for Bogota considers five types of soils. Piedemonte B and Lacustre 200 soil types are used in the research to define the impact of hard and soft soil on implementing the technique. Piedemonte B is a small microzone located east of the city, characterized by colluvial and alluvial soil with a thickness higher than 12m; its
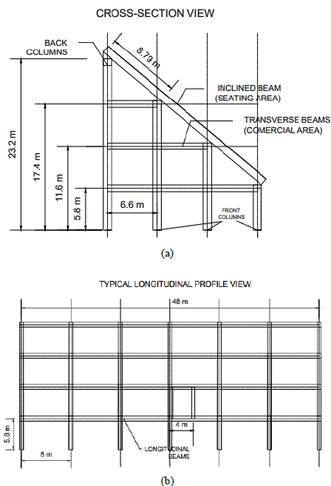
Source: the authors.
Figure 1. Daniel Villa Zapata stadium grandstand geometry: a) cross-section view and b) longitudinal view.
fundamental period ranges between 0.3s and 0.6s, and its shear wave velocity (Vs) ranges between 300 m/s and 750 m/s. Lacustre 200 is an important microzone in the city due to its high extension; it is principally located in the northeast zone of the city, characterized by soft lake soil with a fundamental period range between 2.5s and 3.5s and a shear wave velocity (Vs) less than 175m.
2.2. Geometry of stadium grandstand
The Daniel Villa Zapata stadium grandstand geometry (Fig. 1) is used in the research due to its geometrical simplicity. The maximum height and width are 23.2m and 48m, respectively. Columns are uniformly distributed along the grandstand. The original structure was built in Barrancabermeja city (Colombia) and presents a capacity of 10.000 spectators [19].
The structure does not have any plan irregularities and only has the vertical irregularity named 3A in NSR-10, with a reduction of dissipation energy coefficient (a=0.9. There is no redundancy absence.
2.3. Vertical loads definition
Two types of areas for the grandstand are considered due to their function in the stadium. The first is the seating area, and the other is the commercial area (Fig. 1. Cross-section view). An inclined beam forms the seating area and connects the horizontal and vertical elements. Each zone has two vertical load cases: live (L) and dead (D) loads.
2.3.1. Seating area
The seating area corresponds to where people are located during an event in the stadium; therefore, this zone directly supports the dynamic loads that come from spectators’ movements. The loads are applied in the model on the slope beams.
D loads are estimated according to GN-80/50 grandstand seats (seat width 80cm, backrest 50cm), which have a self-weight of 2.4kN/m.
L loads are defined according to the Colombian earthquake-resistant building code (NSR-10)[20], where for stadium and coliseum stands, a value of 5kN/m2 is recommended. An impact factor of 3 is used to amplify (multiply) live loads to consider the impact of live loads on the structure. That value is taken from experimental research on jumps in soccer matches [19].
By using afferent longitude of 8m, a dead load of 18.8 kN/m and a live load of 120 kN/m are located on the internal inclined beams, while half of the mentioned values are used for external beams.
2.3.2. Commercial area
This type of zone corresponds to the inter-story areas below the grandstand seats, where bathrooms, restaurants, and stores are located.
For stories 1 and 2, D loads of 16.75 kN/m2 are estimated considering an inter-story slab, masonry walls, and other non-structural components. For story 3, which does not include some non-structural components, the D load is 14.85 kN/m2. L loads for all the stories are 6.0 kN/m2, according to NSR-10, for commercial use.
The afferent longitude to estimate loads on beams is 8m, obtained from the separation of columns. For stories 1 and 2, a dead load of 134 kN/m and a live load of 48 kN/m are located on the transversal internal beams (Fig. 1. Cross section view); the middle of those values are used for transversal external beams. Transversal internal beams on story 3 have a dead load of 118.84 kN/m and a live load of 48kN/m; therefore, on external beams, the middle of that values are used.
2.3.3. Other vertical loads
The stairs, with a total width of 4m (Fig. 1. Longitudinal view), were represented through a distributed load on 2m of a specific longitudinal beam of the first level (Fig. 1. Longitudinal view) with a total value of 45.83 kN/m. The roof of the stadium grandstand has a dead load of 2.5 kN/m2, considering its self-weight and wind load according to NSR-10. A load of 0.5 kN/m2 is considered for live load. The roof load is represented as forces and bending moments loads on the back columns. By using afferent areas of 79.2 m2, forces of 198kN and 39.6 kN with bending moments of 1960kN-m and 392.04 kN-m are used for dead and live loads, respectively.
2.4 Seismic loads definition
Seismic loads are defined using spectral accelerations estimated according to the design spectra of NSR-10, which are prescribed for 5% of damping about critical and a return period of 475 years. Regarding the parameters required to build the spectra, the acceleration (Aa, Av) and site effects coefficients (Fa, Fv) for Piedemonte B and Lacustre 200 are shown in Table 1.
A design under the NSR-10 requires the structure to be classified depending on its use. The grandstand was assigned to group III due to the possibility of being designated as a humanitarian shelter after a large earthquake. Therefore, a coefficient of importance of 1.25 was defined for the structure.
Fig. 2 shows spectra calculated for both zones. Again, there is evident amplification in the spectral acceleration’s ordinates for high structural periods in Lacustre 200 soil type.
2.5 Structural analysis and design
The spectral modal analysis was applied to the fixed-base and isolated structures, considering a mass source corresponding to 100% of the dead load and 40% of the live load. The last percentage is due to the possibility of the high presence of people during an earthquake event, which can take the numerical model to have a different behavior during the earthquake simulation. SAP2000 software was the tool for developing the computational 3D model of the grandstand.
2.5.1. Analysis and design of the fixed base structure
The NSR-10 regulation [20] was used to design the fixed base structure. It is worth noting that such code is intended for buildings structures. Although a stadium grandstand is not a building, its structural system is composed of moment-resisting concrete frames similar to those used in buildings.
The analysis used six vibration modes to satisfy the minimum mass participation factor required (90%) for NSR-10. The energy dissipation coefficient R=4.5 used in the structural design was estimated at Ro=5 (moment resisting frames with a moderate capacity of energy dissipation) x (a=0.9 (reduction of dissipation energy coefficient, section 2.2).
An iterative process was carried out to define the properties of materials and the sections assigned to structural elements, satisfying the characteristics, geometry, and loads mentioned in 2.1 to 2.3 and using the load combinations of NSR-10 defined in chapter B.2. It is worth noting that all requirements of design by capacity (column/beam capacity, shear joint capacity, etc.) and drift limit (1% story height) were checked in this stage. In addition, some of the requirements for an intermediate seismic hazard zone, which means moderate dissipation characteristics incorporated in the finite element model, defining the framing type as Sway Intermediate in SAP2000. Orthogonal effects were considered as well in the model.
Fig. 3 shows the numerical model implemented in SAP2000.
2.5.2. Analysis and design of the isolated structure
The guidelines of ASCE 7-16 [21] Standard were used in the research to size the seismic isolation systems because Colombia does not have a local regulation for that type of seismic protection device. Therefore, the NSR-10 code was employed only to design the concrete structure.
As for the structure with a fixed base, information corresponding to six vibration modes was used in the structural analysis. Although, only three modes would be necessary to achieve more than 90% of the participative mass. Modes 4, 5, and 6 correspond to the superstructure in isolated structures; therefore, those modes will be used to compare them with the three fixed base modes.
Table 2 shows the damping ratio β and the period objective (T) for the two types of soils. Such values reduce to low levels the drift and accelerations in the structure, which implies control of the damage in structural and non-structural elements. A higher damping value was required for the structure built on the Lacustre 200 because this is a soft soil that presents acceleration amplification for the zone of objective periods.
However, the acceleration spectra presented in Fig. 2 was modified to account for the reduction in the spectral ordinate due to the higher damping values of the isolated structure, as shown in Table 2. The spectral reduction was carried out using factor B of eq. (1), developed specifically for Colombia by Piscal and Almansa [22].
Where
The B factors obtained for damping and period values of Table 2 are shown in Table 3.
Additionally, the design spectra for isolated structures according to ASCE 7-16 corresponds to the Maximum Considered Earthquake (MCER), meaning a spectrum with a return period of 2475 years. In this research, the MCE spectrum was obtained as 1.5 times the design spectrum (return period of 475 years and no importance factor). This indication is stipulated in ASCE 7-16, 11.4.7 because the NSR-10 does not report how to obtain such spectrum.
The spectra obtained for the isolated structure are shown in Fig. 4 and Fig. 5, for Piedemonte B and Lacustre200, respectively. The spectra for the same soil types and base fixed conditions are included additionally in Fig. 4 and Fig. 5 with the aim of comparison. It is pointed out that spectra for isolated structures were modified due to higher
damping for periods higher than 1s. Lower periods correspond mainly to the superstructure, with damping values lower than 5% of critical. As a recommendation seismic isolation on soft soil must consider the development of a site-soil spectrum.
General considerations (such as location, geometry, and loads) of the structure with a fixed base are employed in the design of the isolated structure. Fig. 6 shows the distribution of HDRB isolators for the grandstand. In this paper, the inclusion of sliders was not considered.
The energy dissipation coefficient R=1.7 used in the structural design was estimated according to the ASCE 7-16, where the R factor of fixed base (4.5) is multiplied by 3/8.
After an iterative process, the materials’ properties and sections were assigned to structural elements to satisfy stiffness (Drift limit 0.5% story height) [22,23] and design requirements. It is relevant to say that all prerequisites to design by capacity (column/beam capacity, shear joint capacity, etc.) were checked in this stage. As for the fixed-base structure, some of the requirements for an intermediate seismic hazard zone, meaning moderate dissipation characteristics, were incorporated into the model, defining the framing type as Sway Intermediate in SAP2000. Finally, orthogonal effects were also considered in the model.
Fig. 7 shows the numerical model for the structure with the isolated base. A link-type element represented by the effective stiffness (K e f f ) of the isolation system is given by:
Where m is the seismic mass and T is the objective period.
3. Results
The following results are presented for the two types of structures in each type of soil. The comparison criteria on the obtained structural designs correspond to: i) the effective stiffness of the isolation system for each one of the twenty-eight isolators (K e f f ) ii) material (concrete, steel) quantities, and iii) the design base shears for each grandstand configuration.
3.1. Fixed base, Piedemonte B soil type
The structural period is 0.54 s and 0.35 s, for X (longitudinal view. Fig. 1) and Y (cross view. Fig. 1) directions, respectively. As expected, the concrete-reinforced four-level grandstand is a suitable structure to be isolated because it presents low periods. The X and Y axis design base shears are 13813 kN and 13746 kN, respectively. The approximate concrete volumes and steel weights necessary for this case are shown in Table 4.
3.2. Fixed base, Lacustre 200 soil type
The change in the soil type to soft soil produced a more flexible design where the structural period is 0.70 s and 0.43 s, for x and y directions, respectively.
On the contrary, the design base shears for the x and y directions are 7602 kN and 7629 kN, respectively. This decrement with respect to the values obtained for Piedemonte B results from the lower spectral ordinate in the acceleration spectra (see Fig. 2). In addition, those design base shears reduce the required concrete volume and the weight of reinforced steel, as shown in Table 5. Finally, it is worth mentioning that material quantities concerning structural foundations were not considered in this research.
3.3. 3.3. Base isolated, Piedemonte B soil type
First, considering the seismic mass (m) of the grandstand (5888 Mg), and the period (T) defined in Table 3, in this case, the effective stiffness (K_eff) of the isolation system has been estimated according to eq. (2). Therefore, for each one of the eighteen isolators the K_eff=922 kN/m.
The design base shears for x and y directions are 3041 kN and 2865 KN, respectively.
The approximate concrete volumes and steel weights necessary for this case are shown in Table 6.
Table 4 Amount of materials: Fixed base Piedemonte B soil type.
Material | Amount | Unit |
---|---|---|
Concrete | 1064 | m3 |
Steel | 189 | t |
Source: The authors.
Table 5 Amount of materials: Fixed base Lacustre 200 soil type.
Material | Amount | Unit |
---|---|---|
Concrete | 901 | m3 |
Steel | 170 | t |
Source: The authors.
Table 6 Amount of materials: Base isolated Piedemonte B soil type.
Material | Amount | Unit |
---|---|---|
Concrete | 775 | m3 |
Steel | 180 | t |
Source: The authors.
Table 7 Amount of materials: Base isolated Lacustre 200 soil type.
Material | Amount | Unit |
---|---|---|
Concrete | 849 | m3 |
Steel | 185 | t |
Source: The authors.
Table 8 Amount of materials: Fixed and isolated structure.
Structure | Decrement in concrete | Decrement of steel |
---|---|---|
Fixed base structure on Piedemonte B | 1 | 1 |
Fixed base structure on Lacustre 200 | 0.85 | 0.90 |
Isolated Base Structure on Piedemonte B | 0.73 | 0.95 |
Isolated Base Structure on Lacustre 200 | 0.80 | 0.98 |
Source: The authors.
3.4. Base isolated, Lacustre 200 soil type
With the seismic mass (m) of the grandstand (5929 Mg), and the period (T) defined in Table 3, in this case the effective stiffness (K_eff) of the isolation system for each one of the eighteen isolators is K_eff=929 kN/m.
The design base shears for x and y directions, are 5562 kN and 5187 kN, respectively.
The approximate concrete volumes and steel weights necessary for this case are shown in Table 7.
Table 8 compares concrete and steel quantities for the different soil types considered and for the fixed base and isolated base structures. These results evidence the impact of seismic isolation on the amounts of materials for structural elements and the differences for each soil type.
It is important to note that using BI is not related to saving money on the structural project but to enhancing the structure's seismic performance. However, it is essential to account for the contribution of the isolation devices in the initial cost to have a feasible project. One impact that can be measured is associated with environmental factors obtained from the reduction in conventional materials.
4. Conclusions
This research has permitted to conclude, regarding the type of structure analyzed, the following:
The fixed base structures' analysis and design show the quantities of materials according to the seismic hazard generated by each soil type study. For example, for short structural periods, higher values of spectral accelerations correspond to Piedemonte B soil type; therefore, the highest amount of materials is obtained for this case.
Generally, there is a reduction in the amount of materials, regarding the fixed base structures, when the grandstand is isolated in its base. The aforementioned is due to the decreased forces generated by flexibility and damping provided by the isolation system.
In the isolated structures, when there is a hard/soft soil type, the percentages of reduction in concrete volumes, regarding the fixed base, for the same damping and period considered, are respectively 27% and 6%. Regarding the amount of steel, there is a reduction in hard soil and an increase in soft soil. The increase in steel indicates that the cross-sections considered in Lacustre 200 isolated base could be closer to those considered in Lacustre 200 fixed base, to keep the same steel amount in both cases. Therefore, the reduction in concrete volume in the structure Lacustre isolated could be lower than 6%.
Although a higher damping value was selected in the case of soft soil, the spectral design acceleration for the considered period (0.19 g) is nearly twice the value in the case of hard soil. (0.1g). To obtain a spectral acceleration near 0.1 g in the soft soil, it is necessary to increase the period of values close to 6s, practically twice the considered period.
It is important, but not mandatory, to obtain a notable reduction in spectral ordinates acceleration in isolated structures, to therefore generate concrete volumes reduction. Otherwise, the total cost of the isolated structures could be very high, due to the additional cost of the seismic isolation system.
The technique of isolated base is more efficient on hard soils; however, it has benefits in seismic risk reduction for both soil types. When a structure on soft soil is considered to apply the technique, it is recommended to use periods higher than conventional ones for low structures, which means periods higher than the range between 2.5-3.5 s.