Introduction
Lauraceae is one of the most important families of angiosperms and includes 55 genera and over 2000 species (Chanderbali et al., 2001; Schmid, 2005; van der Werff and Richter, 1996). Seed oil from the Lauraceae family is characterized by its high lauric acid content, whose concentration can vary depending on the species (Badami and Patil, 1980; Hopkins et al., 1966). This fatty acid is unusual in plants and is highly valued for its health and nutritional benefits. In traditional medicine, lauric acid is used for the treatment of viral infections, such as the common cold, the flu, genital herpes, and many other conditions (WebMed, 2023). Recent studies show that the consumption of lauric acid may be related to a decrease in cognitive impairment (Currenti et al., 2023). The therapeutic properties attributed to lauric acid are its anticancer, antibacterial (against Streptococcus aureus), antiviral (against herpes simplex, vesicular stomatitis and visna), and antifungal (against Candida albicans) activity (Sandhya et al., 2016; Nitbani et al., 2022). Some studies propose that lauric acid helps lower cholesterol levels, combat cardiovascular diseases, and decrease body weight (de Roos et al., 2001; Sandhya et al., 2016).
To date, lauric acid has been found in most Lindera, Cinnamomum, Laurus, Umbellularia and Litsea species. In contrast, capric acid has been mainly described in Sassafras albidum (Lauraceae) seed fat (Badami and Patil, 1980; Gottlieb, 1972; Hopkins et al., 1966; Litchfield, 1971). Lastly, in Persea americana (Lauraceae), oleic, palmitic, and linoleic acids have been observed (Ozdemir and Topuz, 2004).
Ocotea is one of the largest pantropical genera in the Lauraceae family, which comprises approximately 350 species distributed primarily in the Neotropics of America, and some species have also been found in Micronesia, Madagascar and African countries (Rakotondraibe et al., 2015; van der Werff, 2002). Phytochemical studies on the genus Ocotea have reported the presence of aporphine and benzylisoquinoline alkaloids, alkylphenols, arylpropenes, benzopyrans, butanolides, coumarins, essential oils, esters, flavonoids, lignans, neolignanes, sesquiterpenes, steroids, and saponins. Among the biological activities reported are antiherpetic, antioxidant, antimicrobial, antiplasmodial, antimalarial, antifungal, anti-inflammatory, antiproliferative, antithrombotic, antinociceptive, antiprotozoal, antileishmanial, and molluscicidal. Furthermore, it can be employed in the treatment of cardiovascular diseases. It is also known for its cytotoxicity, its larvicidal and cruzain inhibitory activity, and as a mutagenic and recombinogenic agent leading to lethality and toxicity (Garcez et al., 2011; Monte Neto et al., 2008; Montrucchio et al., 2012; Salleh and Ahmad, 2017; da Silva et al., 2017; Zhou et al., 2000). Moreover, in some regions of Amazonia, this oil is used as biofuel to replace kerosene (Marques et al., 2012).
Ocotea caudata (Nees) Mez. is known by the basionyms Ocotea cernua (Nees) Mez. and Oreodaphne cernua Nees (van der Werff, 2002). In Colombia, it is known as jigua negro, jigua baboso, amarillo and laurel. In Peru, it has several common names such as moena, moena blanca, roble, roble blanco and puchuguero, and in Brazil as lauro-preto. The wood is semi-hard, which makes it workable and durable, and highly prized for carpentry and joinery (Reynel and Rodriguez, 2006). In these South American countries, the wood of this plant is greatly valued for its quality and its use as a living fence. To the best of our knowledge, there are few phytochemical and pharmacognostic studies on this plant species; therefore, the present study is the first contribution on fatty acid content in Ocotea caudata (Nees) Mez fruits from Colombia. In addition, considering the large number of new sources of lauric acid with interesting nutritional, cosmetic and pharmaceutical uses, this research aims to contribute to exploring the potential of non- traditional oleaginous plants available in Colombia and to support the chemotaxonomy of the Lauraceae family.
Materials and methods
Plant material sampling
Ocotea caudata (Ness) Mez mature fruits were randomly collected from five to eight plants in the municipality of Puerto López, Meta, Colombia [4°5’28” N, 73°04’21” W]. Fruit samples were separated, cleaned, freeze-dried (-80 °C), grounded and stored in the dark at -20 °C until analysis. The collected specimens were identified by Professor Adolfo Jara Muñoz at the Universidad Nacional de Colombia. The voucher specimen (COL544562) was deposited in the Herbario Nacional Colombiano, in Colombia.
Oil extraction and preparation of fatty acid methyl esters (FAME)
The powdered material (27.3 g) was extracted in a Soxhlet equipment using heptane (500 mL) as solvent. The extraction was carried out according to the AOAC method (AOAC, 2012), with modifications. Briefly, the sample was processed for 4 h at the heptane’s boiling point for 20 runs. The solvent was removed using a rotary vacuum evaporator at 40 °C (Heidolph Instruments, Hei-Vap Precision). The oil fraction was then transferred to sealed amber glass bottles, which were capped and stored at -20 °C until analysis.
O. caudata lipid fraction derivatization was carried out according to Metcalfe et al. (1966) as follows: 50 mg of the lipid fraction was placed in a test tube and 5 mL of BF3-MeOH complex at 14 % were added. Then, the mixture was heated at 85 °C for one hour.
Finally, FAME were extracted with heptane (1 mL x 3), dried with anhydrous Na2SO4, and the resulting dried solution was injected into the gas chromatograph for analysis. All experiments were carried out in triplicate (Metcalfe et al., 1966; Parimelazhagan, 2016).
Gas chromatography with flame ionization detection (GC-FID) analysis
The FAME content was quantified using a Shimadzu GC-2014 gas chromatograph-FID equipped with a RT- 2560 (100 m x 250 μm x 0.2 μm) column and an AOC- 20i+s autosampler under the following conditions: 450 mL min-1 air pressure and 45 mL min-1 H2 pressure.
Helium was used as the carrier gas at 1.2 mL min-1 with a split of 1:200, and a 1.0 mL sample injection. The oven temperature was maintained at 100 °C for 3 min, then increased to 3 °C min-1 and held at a final temperature of 240 °C for 10 min. Injector and flame ionization detector (FID) temperatures were 225 °C and 240 °C, respectively. Identification and quantification of FAME by FID response integration were accomplished by comparing the relative retention times of the sample peaks with those of authentic standards (Food Industry FAME Mix # 35077, Restek, 30 mg mL-1 CH2Cl2).
Fatty acid methyl ester quantification was carried out by the external calibration method. Calibration curves were prepared by diluting a known weight of a standard FAME in CH2Cl2 and analyzing it in the concentration range (Dodds et al., 2005). For quantitative purposes, they were prepared in triplicate. For each FAME, the integrated peak area versus the amount of standard methyl ester were plotted.
Gas chromatography-mass spectrometry (GC-MS) analysis
FAME content identification was confirmed using a gas chromatograph Agilent 7890A GC with split/ splitless injector coupled to a Hewlett Packard 5973 mass selective detector, equipped with a HP- FFAP (50 m x 200 μm x 0.33 μm) column. Operating conditions were as follows: it was used as the carrier gas helium with a 1.0 mL min-1 flow. The oven temperature program was set at 100 °C (0 min) to 240 °C (10 min) at 5 °C min-1 with an injection temperature of 250 °C, and a detector temperature of 285 °C. Ionization voltage was set at 70 eV with a 60 μA ionization current; the range acquisition mass was 40-450 m z-1 with a split of 1:30. The compounds were identified by the comparison of their mass spectra with those of NIST08, Wiley9L libraries spectra. The integration of the total chromatogram, expressed as percent area, has been used as a rough parameter to ascertain the relative composition of the lipid fraction (Dodds et al., 2005).
Results and discussion
O. caudata heptane oil extraction from the fruit yielded a 15 g lipid fraction after the solvent was evaporated under reduced pressure, which represents 54.9 % of the total lipids. Studies on the extraction of fatty acids from Lauraceae species’ seeds using petroleum ether as the extraction solvent results in 20 % and 60 % oil (Hopkins et al., 1966; Kotoky et al., 2007; Wang et al., 1985). Fatty acid extraction yields using petroleum ether and hexane are comparable; however, better yields have been reported with hexane (Taha et al., 1988). Furthermore, heptane is an alternative to hexane for fatty acid extraction; the yield and quality of some seed oils obtained with heptane are similar to extraction processes with hexane (McConnell and Farag, 2013; Taha et al., 1988). In the present work, heptane was selected as the extraction solvent since oil extraction with heptane is faster in comparison with hexane. Moreover, it is more environmentally friendly and less toxic than hexane (McConnell and Farag, 2013; Taha et al., 1988).
Linearity response and sensitivity
Table 1 presents the calibration curves for the different FAME selected including their retention time (Rt), and equation and correlation coefficients (r). Fatty acid standards were detected by GC-FID as FAME in approximately 60 min of analysis. According to the linear regression equations obtained from the calibration curves, the detector response to the tested concentrations was linear with the coefficient of correlation values higher than 0.99 for all fatty acids studied. These values demonstrate that the calibration model was efficient for all standards assayed. For all fatty acids, the slope values of the regression line were similar (mean value of 6.68e-3), which indicates a comparable sensitivity for each fatty acid (Salimon et al., 2017).
GC-FID and GC-MS analysis
Analysis of the O. caudata seed oil obtained from the GC-FID heptane extract presented five prominent peaks (Figure 1). O. caudata concentration and prevalence of saturated, monounsaturated, and poly-unsaturated fatty acids is described in Table 2. Four saturated, one monounsaturated, and one polyunsaturated fatty acids were observed in this study. Lauric acid (C12:0, 51.7 %), oleic acid (C18:1, 23.6 %) and palmitic acid (C16:0, 16.6 %) were the main fatty acids identified. A discrete quantity of myristic acid (C14:0, 2.3 %), stearic acid (C18:0, 2.0 %) and linoleic acid (C18:2, 3.8 %) was also present in the species. The oil was rich in saturated fatty acids, particularly lauric acid, which agrees with the report for other Lauraceae seeds. Hopkins et al. (1966), studied the seed oils of six Lauraceae species (Lindera umbellata, Lindera praecox, Lindera benzoin, Laurus nobilis, Cinnamomum camphora, Umbellularia californica) and classified them into three types: The first grouped species contain saturated fatty acids (C10 and C12), to this group belong Lindera benzoin, Lindera Praecox, Cinnamomum camphora and Umbellularia californica. The second group encompasses principally saturated medium-chain acids (C12) and unsaturated long-chain acids (C18), as observed in Laurus nobilis. Lastly, the third group contains medium-chain saturated and unsaturated fatty acids (C10 - C14) found mainly in Lauraceae such as Lindera umbellata. Due to its contents of lauric acid (51.7 %, C12:0) and oleic acid (23.6 %, C18: 2), O. caudata was classified in the second group.
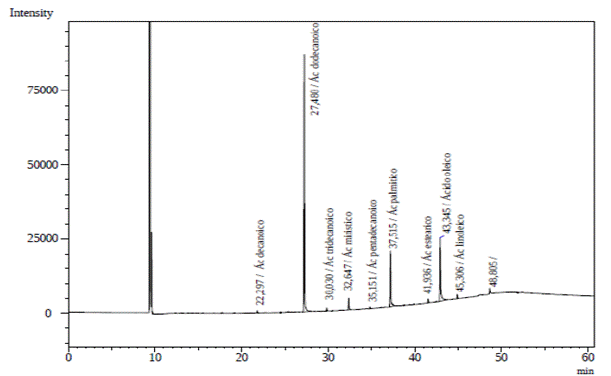
Figure 1 O. caudata fruit lipid fraction from GC-FID chromatogram: Capric acid (22.94 min), lauric acid (27.48 min), tridecanoic acid (30.03 min), myristic acid 32.65 min), pentadecanoic acid (35.15 min), palmitic acid (37.52 min), stearic acid (41.94 min), oleic acid (43.35 min), linoleic acid (45.31 min), and heneicosanoic acid (48.81 min).
Table 2 Fatty acid composition percentage in O. caudata fruits
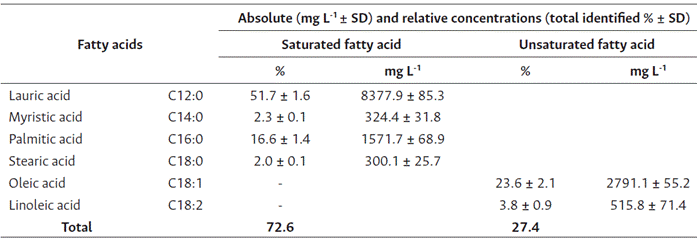
Note: Values are presented as means of total fatty acid methyl ester percentage (n = 3). SD = standard deviation.
The amount of lauric acid found in the species is similar to that of coconut oil (50 %) (Nitbani et al., 2022), suggesting its suitability for soap, detergent and cosmetic production, and all products based on lauric acid, as well as their use as an edible oil (Liebert, 1987). Furthermore, lauric acid inhibits bacteria growth, such as Salmonella sp., Escherichia coli, Staphylococcus aureus, Staphylococcus epidermis, Bacillus stearothermophilus, and others of the Candida, Micrococcus, Pneumococcus, and Pseudomonas genera (Barlina et al., 2022).
Lauric acid has clinical significance, it is the main antiviral and antibacterial substance found in human breast milk, esterified into the monoglyceride form, known as monolaurin (Lieberman et al., 2006; Sandhya et al., 2016). Lauric acid antiviral and antibacterial activity is greater than other medium- chain glycerides, such as caprylic acid (C8:0), capric acid (C10:0) or myristic acid (C14:0) glycerides (Walters et al., 2003; Sandhya et al., 2016). However, it is still unclear whether monolaurin can be synthesized from lauric acid in humans. Nevertheless, there is evidence that some is formed (Lieberman et al., 2006). Monolaurin is biologically many more times active than lauric acid against viruses, coronaviruses and bacteria, posing the interesting question on its conversion rate in humans (Lieberman et al., 2006; Subroto et al., 2020; Nitbani et al., 2022). Hence, it is necessary to study these aspects in O. caudata for economic and commercial use.
Conclusions
The present work, though preliminary in nature, revealed that fruits of Ocotea caudata might be explored for the extraction of lauric acid, which is used in the manufacture of industrial and pharmaceutical products. The study of fatty acids in fruits of Lauraceae contributes to the chemotaxonomy of the family according to their composition of fatty acids, classifying Ocotea caudata within the species that accumulate medium-chain saturated fatty acids (C12) and long-chain unsaturated fatty acids (C18) in their seeds.