I. INTRODUCTION
The contamination of water bodies with heavy metals is one of the most important problems in society and has been addressed by different control entities and researchers [1-2]. Among these pollutants, chromium is one of the most toxic; it is normally found in rocks, animals, plants and soil. It can exist in different forms: Cr (0), Cr (III) and Cr (VI) are the most common forms in which this element is found; Cr (0), called metallic chrome, is used in the manufacture of steel, Cr (III) and Cr (VI) in chrome plating, dyes and pigments, leather tanning, wood preservation, in small quantities, in drills for oil extraction, corrosion inhibitors and the textile industry [3]; being Cr (III) a necessary element to maintain a good state of health at trace levels, because it helps the body to metabolize sugar, fat and proteins [4 - 6].
The adverse health effects of chromium depend on the valence of this element at the time of exposure, the concentration and the solubility of the compound. Short term exposure to Cr (VI) and Cr (III) may cause mechanical irritation to the eyes and respiratory tract, allergic symptoms and nasal sores [7 - 9]. Breathing Cr (VI) over a long period increases the risk of lung and nasal cancer. Studies show that the presence of these in drinking water can cause an increased risk of stomach cancer and reproductive harm [10-11].
Diverse methods have been applied to reduce its presence in water, like chemical precipitation, ion exchange, solvent extraction, membrane processes, electrodialysis, phytoextraction, ultrafiltration, reverse osmosis and adsorption [12 - 14]. These methods present limitations when working at trace levels, involving the use of expensive reagents, energy requirements, sludge generation, and operating expenses; thus the adsorption technique emerges as a viable alternative because it is an efficient and low-cost method [12, 15]. Therefore, this study proposes the possibility of taking advantage of the residual biomass from the process of obtaining plantain starch, as a Cr (VI) adsorbent from synthetic waters that simulates the concentration of the metal ions understudied, present in the wastewater; which has become important as a food alternative, among other applications, compared to other starches; keeping in mind that starch is used in different sectors of the industry, especially in food; the textile, paper, bioplastics, dextrin and glues manufacturing industries, among others. There is currently a trend towards the search for new alternatives to native starches or to physically or chemically modified starches [16-17]. At the same time, the characteristics of the biomass that facilitate the process of Cr (VI) adsorption are evaluated by assessing the effect that the bed height in the packed column has and the effect that the temperature variation in the aqueous solutions generates on this adsorption.
II. METHODS
This section describes the experimental procedure to carry out Cr (VI) adsorption tests on a fixed bed column packed with the residues of the plantain starch process, for which a factorial design in response surface of type 22 compound was followed star central.
A. Preparation of the Adsorbent and Cr (VI) Solution
The plantain (Dominico harton) was collected from farms in the department of Bolivar (Colombia). The plantain was peeled, sliced and washed several times with distilled water to remove different impurities. After that, it was immersed in a 0.25% NaOH solution and cooled to 5 ºC for 20 h; the pH was adjusted using HCl and NaOH 2M to recover the residual biomass of interest by filtration [18]. The residual biomass was dried for 8 h in the sun, subjected to size reduction and classified by choosing particle sizes between 2 mm and 1 mm. A 1000 ppm Cr (VI) stock solution was prepared using K2Cr2O7 (Merck Millipore), analytical grade, in deionized water; from this stock solution, it was diluted to obtain the different concentrations used in the adsorption tests [19].
B. Adsorption Tests in Continuous System
The experiments were performed on an acrylic column, with an internal diameter of 41 mm and a height of 150 mm, the solution which contained the Cr (VI) ions flowed into the column by gravity, with a flow rate of 0.75 mL/s adjusted through a balloon valve. The column was packed with particle sizes between 1 and 2 mm, determining the effect of bed height and temperature over the adsorption efficiency. Temperature variation and control was performed employing a REX C-100 temperature controller. The samples to measure the final concentration of the ions present in the solution were taken at the bottom exit of the column and the final concentration data were determined with UV-VIS spectrophotometry using the colorimetric method of 1.5-diphenylcarbazide at 540 nm applying the standard method ASTM D1687-17 [20]. The statistical analysis was done with the software Statgraphics Centurion 18.1.02, determining the best experimental condition at which the rupture curve was made. The efficiency and adsorption capacity were calculated as equations (1) and (2).
Where Ci and Cf are the concentrations at the entrance and exit of the column in mg/L, q is the adsorption capacity in mg/g, V is the volume in L and m the amount of adsorbent in g.
C. Breakage Curve and Adsorption Models in a Continuous System
The breakage curve was made at the optimal conditions found when applying the Response Surface Methodology (RSM). The models used to predict the performance of the rupture curve, calculating the process constants and evaluating the capacity are shown in Table 1.
D. Characterization of Biomass as Bioadsorbent Material
The adsorbent (before and after the modification) was characterized by Fourier Transform Infrared Spectroscopy (FTIR) and by scanning electron microscopy (SEM). The morphology of the surface was studied in a JEOL Scanning Electron Microscope, model JSM 6490-LV with excitation energies from 0.1 kV to 30 kV, to know elemental composition of the material and its structure; Gold was used to coat the samples using a Desk IV Metallizer that provides a fine grain coating (100Å) in a single coating cycle. The evaluation of the functional groups was carried out using a Thermo Scientific Nicolet 6700 optical FTIR spectrometer through 64 scans in Transmittance mode from 4000 to 500 cm-1 of 4 cm-1 [21].
III. RESULTS AND ANALYSIS
This section present the characterization of the biomass used, as well as the effect of the variation in temperature and bed height on the efficiency of removal of Cr (VI) in a continuous system.
A. Characterization of Biomass
From the FTIR analysis shown in figure 1 before adsorption, records between 3000 and 3500 cm-1 are highlighted where unsaturated =CH- (alkenes and aromatics) may exist, also between 3200 and 3500 cm-1 amines and small carbonyl overtone signals may appear due to stretching vibrations of the O-H bond. The peak of 2927.94 cm-1 is attributed to possible vibrations of C-H methyl, methylene and methoxy groups, between 2000 and 2500 cm-1 there are signs of alkenes and carboxylic acids that may be due to the stretching of O-H. Peaks close to 1650 cm-1 are also recorded which indicate the presence of functional groups of cellulose, hemicellulose and lignin due to stretching of C=O and C-O by the vibrations of carboxyl groups of pectin, hemicellulose and lignin [1, 19, 21, 22], and also may indicate the presence of aromatic rings due to hydrogen vibrations representing the stretching of C=C [23].
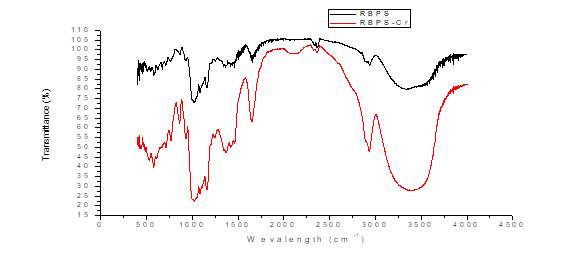
Fig. 1. FTIR spectrum for residual plantain starch biomass (SBPS) before and after Cr (VI) (SBPgS-Cr) adsorption.
On the other hand, between 1000 and 1200 cm-1 evidence the presence of primary, secondary and tertiary alcohols, a product of the stretching vibrations of the C-OH bond and the bending between the plane (1225-950 cm-1) are only complementary signals because the C-C, C-N and C-O stretch fall in the same region and several signals appear depending on the number of hydrogen. It is important to note that the 600 ± 50 bands show torsional vibrations of the C-OH bond and weak but acute signals, which can increase significantly in the presence of polar groups [24 - 26]. After the adsorption process, an increase in the width of the spectral bands and a slight decrease in intensity can be observed. The decrease provided by the variation of the adsorption frequency can be attributed to the binding of Cr (VI) ions to the different functional groups as corroborated in the peak at 2341 cm-1 due to a possible interaction of hydrogen bridges, as well as in the change of intensity of the absorption peak at 2927.94 cm-1 attributed to the vibrations of C-H methyl, methylene and methoxy groups present in the biomass that facilitate the adsorption process and justify the high percentages of Cr (VI) ion retention at high temperatures [26].
Figure 2 shows the SEM images of the residual plantain biomass, observing a rather uniform and cylindrical aspect in shape. From figure 2a it can be seen that the adsorbent has a tubular, uniform and bonded structure [21]. Figure 2b, corresponding to the SEM analysis after the adsorption of Cr (VI), no precipitation of the metal on the surface of the biomaterial is evident and the ions were found to adsorb inside the pores of the adsorbent, which can be attributed to a mechanism of electrostatic attraction and interactions by hydrogen bonds between the pollutant and the active sites of the biomass [27].
The EDS analysis shown in Figure 3 revealed that the elemental composition of the material is mainly carbon and oxygen, these being the main elements in weight % for the residual biomass of plantain starch: C (58.32), O (41.07), K (0.24), Na (0.20), Cl (0.10) and Cu (0.07). The presence of Cr (VI) in the spectrum after the removal process is observed with 1.12 % in weight.
The analysis SEM-EDS was performed to better understand the mechanism of interaction between ions Cr (VI) and the residual biomass of plantain. There are different types of mechanisms for the elimination of Cr (VI) by biosorbents, such as electrostatic interaction, where ions are adsorbed on the surface of the positively charged adsorbent without any reduction of Cr (VI); complete reduction, where the ions are adsorbed and completely reduced on the adsorbent to Cr (III); cationic adsorption, where the ions are completely reduced by the adsorbent to (III) and then adsorbed on the biomaterial surface; adsorption together with reduction, where the ions are partially adsorbed on the adsorbent and the rest is reduced to the Cr (III) form [28]. These mechanisms, responsible for the removal of Cr (VI) by biomasses, are probably attributed to the chemical composition and properties of the bio adsorbent, which largely depend on the characteristics of the source of the raw material. The structure of biomass of lignocellulosic origin is composed of lignin, pectin, cellulose and hemicellulose, which guarantee the presence of functional groups rich in Oxygen, carbon fractions with aromatic structure, unsaturated and amino compounds, and some mineral constituents that function as sites exchange [23, 29].
On the other hand, experimentation in the present study was carried out at pH 2 where the predominant species of Cr (VI) is HCrO4-, which consumes only one adsorption site and is easy to adsorb due to electrostatic interactions between the ion and the centers adsorbent assets [30]; amino groups (-NH2) also play an important role in the adsorption of anions through the complex formation and ion exchange, since at low pH, amino groups are highly protonated and positively charged, which favors the absorption of species Cr (VI) anions present in aqueous solution at acidic pH [28, 31]. Previous studies showed that the protonation of functional groups on the surface of adsorbents increases under acidic rather than alkaline conditions, leading to a significantly strong electrostatic attraction between the active centers of positively charged biomaterials and chromate anions [32-33].
The reduction of Cr (VI) to Cr (III) in acidic conditions, with the concomitant oxidation of the carbon surface, to form new functional groups that contain oxygen, so the possible explanation for greater adsorption in acidic conditions is that Cr (VI) is oxidized to Cr (III) for its subsequent uptake in the active centers [28, 34-35].
The disappearance of some metals present in smaller proportions as Na and K were observed, and a significant decrease in the presence of O and Cu. These results are attributed to the formation of links between the ion and the active sites of the lignocellulosic material. Besides, there is a significant increase in the presence of C for all biomaterials, which is due to the presence of active sites without saturation at the time of sampling. This presents a good indicator concerning the removal capacity of the bio adsorbent [27].
B. Effect of Bed Height and Temperature on Cr (VI) Adsorption
The results of the removal of biomass residues of plantain starch, in the different configurations of operation in the fixed bed column where the Cr (VI) adsorption tests were performed, are shown in Table 2.
Table 2 Adsorption efficiency for Cr (VI)
Bed height (mm) | Temperature (°C) | % of adsorption |
65 | 33 | 65.67 |
100 | 40 | 82.45 |
30 | 40 | 63.51 |
114.5 | 55 | 94.78 |
65 | 55 | 92.37 |
15.5 | 55 | 54.87 |
100 | 70 | 94.78 |
30 | 70 | 89.19 |
65 | 76 | 95.42 |
The real effects of the variables studied were analyzed by the software Statgraphics Centurion employing of the Pareto diagram shown in Figure 4, it is observed that the two variables: bed height and temperature have a significantly positive effect on the removal of Cr (VI) ions, in addition that the adsorption efficiency is favored with an increase of both variables.
Likewise, applying the RSM resulted in the regression equation that adjusts the data obtained, as follows:
Where T is the temperature and A the height of the bed, of the coefficients it is observed again that these two variables are the ones that present a high influence in the process, obtaining a satisfactory second-order predictive regression model [36-37]. The predictions they provide are shown in the function's graph as an estimated area (Figure 5).
From the estimated values on the response surface, in order to have a high efficiency in Cr (VI) ion adsorption was obtained that the optimal values to maximize the removal at 99.23 % corresponding to a bed height of 81.49 mm and a temperature of 68°C.
An upward behavior of the Cr (VI) removal percentage was found concerning temperature increase, suggesting that the process is endothermic [38]; this could be due to the temperature-proportional increase of the collision frequency between the adsorbent and the metal ions, which reach more kinetic energy to diffuse from the solution phase to the solid phase of the adsorbent [39], so at higher temperatures, some of the components on the surface can dissociate leading to the generation of more active sites to which the heavy metal can bond [40-41]. The behavior shown by the adsorption efficiency with regard to the adsorbent dose is due to the increase of the residence time of the solution inside the column, so Cr (VI) has more time to diffuse into the solid phase, dominating over the axial dispersion, which at low bed heights predominates in the mass transfer and reduces the diffusion of metallic ions [42 - 44].
C. Breakage Curve
In order to carry out the determination of the bed life, the values of the factors that gave the highest removal were selected and implemented for the packaged column configuration and operation of the adsorption tests, to carry out the establishment of the bed life and breakpoint through a forward curve over a period of six continuous hours. The forward curve is shown in Figure 6.
The metal ion showed a fast adsorption speed in the first minutes, which can be explained by the high availability of active sites and Cr(VI) [45]. As the solution continued flowing, the output concentration started to increase, however during the 6 hours of the process the saturation of the material was not reached, indicating the high adsorption potential of the material under study, with a maximum adsorption capacity of 29.85 mg/g [8]. The parameters of adjustment to the adsorption models of these experimental results were adjusted to each of the Dose Response, Yoon-Nelson, Thomas and BDST models are shown in Table 3, this in order to describe the behavior of the column.
Table 3 Adjustment parameters to adsorption models in a continuous system
Model | Parameters | Values |
BDST | N BDST (mg/L) | 9.151 |
KBDST (L/mg.h) | 9.151 | |
V (cm/h) | 0.02 | |
R2 | 0.466 | |
Thomas | kTh (mL/min.mg) | 0.04 |
qth (mg/g) | 30.67 | |
R2 | 0.599 | |
Dose-Response | qD-R (mg/g) | 4.08 |
A | 0.203 | |
R2 | 0.139 | |
Yoon-Nelson | k Y-N (1/min) | 0.0048 |
qYN (mg/g) | 30.23 | |
R2 | 0.612 |
One of the most widely reported models for predicting advance curves in fixed-bed columns is the Thomas model. This model assumes the flow of the plug along the fixed bed while neglecting axial dispersion in the column. He also considers Langmuir's adsorption-desorption kinetics and considers that the driving force is governed by reversible second-order kinetics; however, q0 and kT are treated as empirical parameters that are estimated by fitting the model to experimental data [46]. On the other hand, the Yon-Nelson model assumes that the rate of decrease in the probability of adsorption of each adsorbate molecule is proportional to the probability of adsorbate advancement in the adsorbent [47]. According to the correlation coefficient (R2) it was established was found that Thomas and Yoon-Nelson's model moderately describe the experimental data by a similar fit of 0.599 and 0.612 respectively, while the other 2 models were further away, so it is suggested that for the scaling up of this type of processes work should be done on the development of an own model [48]. The calculated values of the maximum bed adsorption capacities (q0) were similar for both models and relatively close to the value obtained experimentally. According to the literature, for the evaluated bed height (81.49 mm), the axial dispersion phenomena increase during the mass transfer process, which increases the diffusivity of the ions in the bed, so that the ions have the time enough to diffuse in the adsorbent due to the high availability of active sites [49-50].
IV. CONCLUSIONS
From the results, it is established that the residual biomass of banana starch is an efficient material for the removal of Cr (VI) in aqueous solution. It is also observed that the hydroxyl and methyl functional groups are the ones that mainly participate in the removal; the SEM and EDS show that the process is controlled by electrostatic interactions between the metal and the active centers of the adsorbent. In addition, the temperature and the bed height have a significant proportional effect on the metal adsorption in a continuous system. When applying the RSM, the optimal operating conditions are 81.49 mm bed height and 68°C.