INTRODUCTION
The cape gooseberry, an exotic tropical fruit, holds significant importance due to its nutritional properties as a rich source of vitamins (ascorbic acid, thiamine, riboflavin, and niacin), minerals (calcium, phosphorus, and iron), and the presence of secondary metabolites such as steroids, flavonoids, and alkaloids (Agudelo-Sánchez et al., 2023; Balaguera-López et al., 2024; Álvarez-Herrera et al., 2024). Colombia stands out as the primary producer and exporter of cape gooseberry, contributing to approximately 90% of the world's production, reflecting a remarkable increase of 22.72% between 2020 and 2022 (Agronet, 2023). In 2022, cape gooseberry exports experienced a 1% growth compared to 2021, with the Netherlands, the United States, and Germany being the major export destinations, accounting for 64.2, 15.7, and 6.7%, respectively (Analdex, 2023).
The conventional marketing approach for cape gooseberry involves fresh produce, rendering it susceptible to manipulation during international processes. Due to its high respiratory activity and sensitivity to ethylene, the fruit undergoes accelerated senescence, resulting in softening and limiting its post-harvest life to only 10 to 12 d, this phenomenon leads to losses amounting to up to 21% of the total production in postharvest, equivalent to 1,813-2,373 t of cape gooseberry (Cárdenas-Barboza et al., 2021). Coupled with the prolonged duration of the journey from Colombian seaports to the European market, which can span up to 18 d, exporting cape gooseberry presents a considerable challenge (Fischer et al., 2014).
To address these challenges, various tools are available to extend the post-harvest life and maintain the quality of fruits. One such tool is refrigeration (Diaz and Avila, 2021), whose efficacy has been enhanced by strategies like precooling. Precooling involves the rapid elimination of field heat in freshly harvested products, lowering the temperature close to that of storage, and reducing the respiratory rate of the fruit. This process occurs before the product is transported to the market or stored in a cold environment (Duan et al., 2020). Among the precooling techniques, hydrocooling (HC) stands out, involving the immersion of fruits in water with low temperatures (Makule et al., 2022).
According to Duan et al. (2020), hydrocooling (HC) has demonstrated excellent results by minimizing gas exchange in small fruits, which, due to their large surface-to-volume ratio, tend to lose water rapidly. In a similar way, in melons, HC application has proven effective in preserving the quality attributes of fruits for an extended duration during refrigerated storage, preventing metabolic activity, and maintaining cell wall integrity (Zainal et al., 2019). Comparable outcomes have also been observed in other fruits such as tomatoes (Mani et al., 2023), peaches (De et al., 2020), cherries (Wang and Long, 2015), and pears (Choi et al., 2015).
The immersion of fruits in water may lead to fruit cracking. One approach to mitigate cracking is through the exogenous application of calcium (Ca2+) (Zhang et al., 2019a). The absence of calcium can result in tissue breakdown, an increase in intercellular space separation, and fruit malformation, as calcium plays a crucial role in both cell division processes and wall structure. Hence, its deficiency can induce cracking (Gao et al., 2019). Cape gooseberries are particularly susceptible to this physiological issue, primarily due to calcium deficiency in the plant, inadequate irrigation management during fruit growth, and the fruit size, with larger fruits being more susceptible to cracking (Álvarez-Herrera et al., 2012; Álvarez-Herrera et al., 2015).
Therefore, the objective of this study was to assess the impact of calcium chloride (CaCl2) and hydrocooling on the postharvest behavior and quality of cape gooseberry fruits.
MATERIALS AND METHODS
Location and plant material
The research was conducted at the plant physiology laboratory of the Universidad Pedagógica y Tecnológica de Colombia, situated at a latitude of 5°33' N and a longitude of 73°24' W, at an elevation of 2,787 m a.s.l. The laboratory has an average annual temperature of 12.5°C. The Colombia ecotype cape gooseberry fruits were sourced from the 'Las Nubes' farm in the municipality of Ventaquemada, located at 5°23'25.7928'' N, 73°29'11.4576'' W, and an elevation of 2,675 m a.s.l. The farm experiences an average temperature of 12°C and an average annual precipitation of 882 mm.
Fifty kilograms of cape gooseberry fruits were harvested at ripening stage 3, following the ICONTEC (1999) NTC 4580 standard. The fruits were transported to the laboratory in 25 kg baskets, where the calyx was removed, and a selection process was conducted on 30 kg, considering fruits with uniform size, color, and the absence of damage.
Experimental design
A completely randomized design was employed, comprising seven treatments (Tab. 1) with four repetitions, totaling 28 experimental units (EU). Each experimental unit consisted of 1 kg of cape gooseberry fruits.
Table 1. Treatments used.
Treatments | Precooling (HC) | CaCl2 (%) | Storage temperature (°C) |
---|---|---|---|
+HC0CaCl24ºC | Hydrocooling | 0 | 4 |
+HC0.5CaCl24ºC | Hydrocooling | 0.5 | 4 |
+HC1.0CaCl24ºC | Hydrocooling | 1.0 | 4 |
+HC1.5CaCl24ºC | Hydrocooling | 1.5 | 4 |
-HC0CaCl24ºC | Without precooling | 0 | 4 |
+HC0CaCl216ºC | Hydrocooling | 0 | 16 |
-HC0CaCl216ºC | Without precooling | 0 | 16 |
Before the application of treatments, the fruits underwent disinfection using 0.1% (v/v) NaClO in distilled water for 10 min. For hydrocooling (HC) treatments, the fruits were immersed in distilled water at a temperature of 4°C for 15 min. In conjunction with HC, calcium chloride (CaCl2) concentrations (Merck KG, Darmstadt, Germany) in distilled water were prepared at 0.5, 1.0, and 1.5 (m/v). These concentrations were chosen as they enhance the Ca2+ concentration in cape gooseberry fruits after immersion in the solution (Pinzón-Sandoval et al., 2016; Reyes-Medina et al., 2017). Following the completion of the HC process, the fruits were placed in T-1 polystyrene trays (Ajover Darnel, S.A.S., Colombia) and stored at either 4 or 16°C (room temperature), depending on the specific treatment.
Response variables
Measurements for the treatments were conducted every 3 or 4 d, concluding at 19 days after harvest (DAH) for treatments stored at room temperature (16°C), while refrigerated fruits were measured up to 33 DAH.
Mass loss (ML) was determined by weighing 100 g of fruits in their initial state, using a VîBRA AJ220E semi-analytical balance (Shinko Denshi Co., Ltd., Japan), with a precision of 0.001 g. ML was calculated using equation (1):
where M1 represents the initial mass, and M2 represents the final mass.
Fruit firmness was quantified utilizing the GY-4 penetrometer (Yueqing Handpi Instruments, China), equipped with a 3.5 mm diameter tip, a pressure depth of 10 mm, and a precision of 0.01 N. The average value from three fruits of uniform size for each experimental unit (EU) was recorded and expressed in Newtons (N).
Total soluble solids (TSS) were quantified in 1 mL of juice using the HANNA HI96801 digital refractometer (Hanna Instruments, Woonsocket, RI) with an accuracy of 0.1°Brix. The total titratable acidity (TTA) was calculated following the methodology outlined by Jaime-Guerrero et al. (2022) and expressed as a percentage of citric acid. The maturity ratio (MI) was determined as the ratio of TSS to TTA.
The pH was obtained by diluting 5 mL of juice in 45 mL of distilled water, resulting in a final volume of 50 mL, using the Hanna HI 8424 portable digital pH potentiometer (Hanna Instruments, Woonsocket, RI). Respiratory intensity (RI) was determined in 100 g of fruits following the methodology by Álvarez-Herrera et al. (2021), and the results were expressed as mg kg-1 h-1 of CO2.
The color of the epidermis was assessed using the CIELab scale with a CHNSpec CS-200 digital colorimeter (Hangzhou CHNSpec Technology, China). Three fruits per experimental unit were analyzed, and the color index (CI) was calculated using equation (2):
where, L* represents the luminosity of the color, where a value of 0 corresponds to black, and 100 indicates white. The values of a* less than 0 signify a tendency toward green, while values greater than 0 indicate a tendency toward red. Similarly, values of b* less than 0 suggest a tendency toward blue, and values greater than 0 suggest a tendency toward yellow.
Data analysis
The obtained values underwent normality tests, followed by an analysis of variance (ANOVA) to discern differences among the treatment means. In addition to the Tukey test (P≤0.05) to classify the treatments, an orthogonal contrast test was performed in which different groups of treatments were compared (Tab. 2), using the SAS v software. 9.2e (SAS Institute Inc., Cary, NC).
Table 2. Orthogonal contrasts used to compare the groups of treatments evaluated in the post-harvest of cape gooseberry.
Contrast | +HC0CaCl24ºC | +HC0.5CaCl24ºC | +HC1.0CaCl24ºC | +HC1.5CaCl24ºC | -HC0CaCl24ºC | +HC0CaCl216ºC | -HC0CaCl216ºC |
---|---|---|---|---|---|---|---|
Refrigeration vs. room temperature | 2 | 2 | 2 | 2 | 2 | -5 | -5 |
HC vs. without HC | 2 | 2 | 2 | 2 | -5 | 2 | -5 |
CaCl2 vs. without CaCl2 | -3 | 4 | 4 | 4 | -3 | -3 | -3 |
CaCl2 0.5% vs. CaCl2 1.0% | 0 | 1 | -1 | 0 | 0 | 0 | 0 |
CaCl2 0.5% vs. CaCl2 1.5% | 0 | 1 | 0 | -1 | 0 | 0 | 0 |
CaCl2 1.0% vs. CaCl2 1.5% | 0 | 0 | 1 | -1 | 0 | 0 | 0 |
HC: hydrocooling; 0, 0.5, 1.0, 1.5% of CaCl2; 4°C and 16ºC.
RESULTS AND DISCUSSION
Mass loss (ML)
ML in cape gooseberry fruits increased over the storage period in all treatments, revealing highly significant differences within the initial 19 DAH. Fruits stored at room temperature, both with and without hydrocooling (+HC0CaCl216ºC and -HC0CaCl216ºC), exhibited the highest ML values at 45.44 and 43.45%, respectively. In contrast, fruits stored in refrigeration at 4°C demonstrated an average ML of 9.9% at 19 DAH (Fig. 1A), a critical period at which fruits preserved at room temperature (16°C of storage) lost their commercial quality. The application of CaCl2 at any dose showed significant differences compared to fruits not treated with CaCl2. Generally, fruits subjected to hydrocooling (HC), refrigeration, and 1% CaCl2 application (+HC1.0CaCl24ºC) displayed the lowest ML values (20.81%) after the postharvest period. Additionally, fruits relying solely on refrigeration without HC or calcium application recorded the highest ML at 33 DAH (28.58%), emphasizing the significance of HC in refrigerated fruits during postharvest.
The ML during fruit postharvest is attributed to water release through transpiration and respiration processes, directly influenced by temperature (Jaime-Guerrero et al., 2022). Refrigeration at low temperatures effectively delays ML by diminishing gas exchange in cape gooseberry fruits (Balaguera-López et al., 2015). Likewise, HC plays a crucial role in removing field heat from agricultural products, enhancing the post-harvest performance of refrigerated fruits. Wang and Long (2015) applied HC (0°C) to cherries immersed in 0.2 and 0.5% CaCl2 for 5 min, successfully reducing ML during refrigeration at 0°C. Moreover, HC is reported as the most recommended precooling technique for small fruits with a high surface-to-volume ratio, such as cape gooseberry, as it minimizes moisture loss and maintains turgor pressure in the cells (Duan et al., 2020).
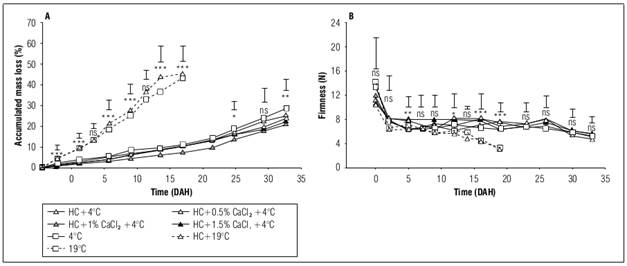
Figure 1. Effect of refrigeration, hydrocooling (HC at 4°C), and CaCl2 on mass loss (A) and firmness (B) in cape gooseberry fruits during storage. ns: not significant. *, ** and *** indicate significant effect according to the Anova P≤0.05, P≤0.01 and P≤0.001, respectively. Vertical bars indicate minimum significant difference according to Tukey (P≤0.05).
Firmness
Firmness values declined with advancing storage duration across all treatments (Fig. 1B). Fruits subjected to hydrocooling (HC), refrigeration, and the application of 1 and 1.5% CaCl2 exhibited greater firmness at 5, 12, and 16 DAH in comparison to other treatments. Similarly, starting from day 12, refrigerated fruits consistently displayed significantly higher firmness (7.32 N) compared to those stored at room temperature (5.90 N), regardless of the hydrocooling. This disparity persisted at 19 DAH, with firmness values of 7.0 and 3.6 N for refrigerated and room temperature-stored fruits, respectively. Treatments with HC showed highly significant differences from 14 DAH onwards compared to fruits that did not receive HC. However, from 23 DAH onward, no significant differences in firmness were observed for refrigerated treatments, which averaged 5.27 N at 33 DAH.
The decline in firmness during fruit ripening initiates with heightened enzyme activity, such as polygalacturonases (PG), pectinases, and cellulases, responsible for hydrolyzing structural and reserve polysaccharides in the cell wall (Saltveit, 2019). Given the enzymatic nature of these processes, storage temperature becomes a crucial factor influencing metabolic activity. Hence, storage at lower temperatures, as observed in this study, decelerates the loss of firmness, consistent with findings by Pinzón et al. (2015) in refrigerated cape gooseberries. Additionally, the application of Ca2+ is known to play a vital role in preserving the cell wall integrity of fruits (Zhang et al., 2019b; Gao et al., 2020), elucidating the reduced loss of firmness in fruits refrigerated and subjected to hydrocooling with 1.0 and 1.5% CaCl2 doses during storage. This underscores the significance of Ca2+ in postharvest maintenance. Similarly, combining hydrocooling with calcium applications has proven effective in sustaining firmness in cherry fruits (Wang and Long, 2015), apples (Ganai et al., 2018), and peaches (Pervitasari et al., 2021).
Total soluble solids (TSS)
Cape gooseberry fruits exhibited significant differences in TSS among treatments at 5, 9, 19, 23, 26, 29, and 33 DAH (Fig. 2A). Throughout the postharvest period, there was a slight upward trend in TSS, increasing from an average of 11.44 to 12.95 °Brix across all treatments. Notably, fruits without refrigeration (+HC0CaCl216ºC and -HC0CaCl216ºC) displayed higher TSS at 14 DAH, decreasing until 19 DAH (13.0 °Brix), while refrigerated fruits consistently showed significantly higher values (13.9 °Brix). There were significant differences between the different doses of ClCa2 at 9 and 19 DAH, where applications of 1.5 and 1.0% presented the highest TSS values, respectively. Beyond 19 DAH, TSS exhibited a slight decline, with refrigerated, calcium-applied, and hydro-cooled fruits maintaining higher values at the end of storage compared to the treatment with only refrigeration (-HC0CaCl24ºC).
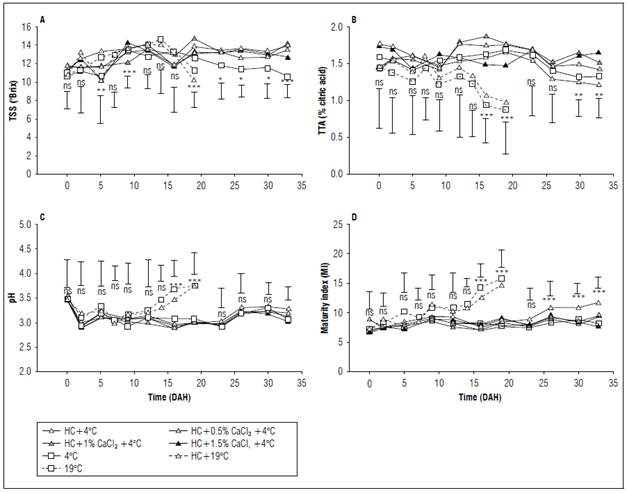
Figure 2. Effect of refrigeration, hydrocooling (HC at 4°C), and CaCl2 on A) total soluble solids (TSS), B) total titratable acidity (TTA), C) maturity index (MI), and D) pH in cape gooseberry fruits during the storage. ns: not significant, *, ** and *** indicate significant differences between treatments according to the Anova P≤0.05, P≤0.01 and P≤0.001, respectively. Vertical bars indicate minimum significant difference according to Tukey (P≤0.05).
The initial rise in TSS during storage is linked to the degradation of reserve polysaccharides into simple sugars (glucose, fructose, and sucrose), facilitated by starch hydrolysis mediated by enzymes such as α-amylase and amyloglucosidase (Yahia et al., 2019). This explains the increase in TSS up to 14 and 19 DAH in fruits stored at room temperature and refrigerated conditions, respectively. Subsequently, the decline in TSS is attributed to the utilization of sugars as a respiration substrate (Saltveit, 2019), coinciding with the climacteric peak reached by cape gooseberry fruits. Similar behavior was observed with the application of hydrocooling in peaches cv. Mibaekdo stored at 10°C (Pervitasari et al., 2021), mangoes (Renu et al., 2022), and Indian gooseberries (Tomar and Pradhan, 2023). In contrast, the application of CaCl2 at 20°C did not impact the TSS of cape gooseberry fruits (Reyes-Medina et al., 2017).
Total titratable acidity (TTA)
The TTA values of cape gooseberry fruits exhibited a slight decrease throughout the storage period and only demonstrated significant differences at 16 and 19 DAH between treatments. At these points, the TTA of fruits stored at room temperature (-HC0CaCl24ºC and -HC0CaCl216ºC) significantly decreased (Fig 2B), showing reductions of 41.56 and 46.75% compared to the average of the refrigerated treatments, respectively. When analyzing the application of HC, it generated significant differences from 16 to 19 DAH, compared to the treatments that did not have HC, likewise, the application of CaCl2 showed significant differences from 12 DAH, in which the 1% dose had the highest TTA.
The reduction in TTA during postharvest is attributed to the utilization of organic acids as a substrate in the respiratory metabolism of fruits and/or in the gluconeogenesis process for sugar generation (Vallarino and Osorio, 2019). This decrease is also associated with the use of refrigeration to delay respiratory metabolic processes in cape gooseberry fruits (Pinzón et al., 2015). Towards the end of postharvest, refrigerated fruits treated with HC and CaCl2 applications (+HC0.5CaCl24ºC, +HC1.0CaCl24ºC and +HC1.5CaCl24ºC), irrespective of the dose, exhibited the highest TTA values compared to fruits with only HC and refrigeration, suggesting that calcium application enhances the performance of cape gooseberry fruits and prolongs the accumulation of organic acids. This aligns with findings in cape gooseberry by Pinzón-Sandoval et al. (2016) and Reyes-Medina et al. (2017) and is similar to the reported effect on cherries refrigerated at 0°C with HC and varying Ca doses after four weeks of storage (Wang and Long, 2015). In this context, it is plausible that calcium application suppresses ethylene-dependent processes at the cellular level, thus retarding fruit ripening (Dorostkar et al., 2022).
Maturity index (MI)
Figure 2C illustrates the behavior of the MI, which increased in all treatments, reflecting the maturation process. During the initial 14 d, the MI did not show significant differences between treatments and had an average value of 8.45±0.68. From this point onward, fruits stored at room temperature (+HC0CaCl216ºC and -HC0CaCl216ºC) exhibited an increase in the MI, reaching values of 11.35, significantly higher than the averages of refrigerated fruits (7.54). The application of HC and CaCl2 separately presented significant differences between 16 and 19 DAH, and between 9 and 19 DAH, respectively. HC delayed fruit ripening since it maintained lower MI values. The latter slightly increased the MI towards the end of postharvest, indicating slower ripening. At 33 DAH, fruits subjected to HC, refrigeration, and CaCl2 displayed the lowest MI value, aligning with the findings of Pinzón et al. (2015), who observed lower MI values in refrigerated fruits due to the maintenance of high TTA values compared to fruits stored at room temperature.
pH
There were no significant differences between treatments in the pH of cape gooseberry fruits during the initial 14 d (Fig. 2D), where it averaged 3.18±0.2. However, at 16 and 19 DAH, fruits stored at room temperature with and without hydrocooling (HC) exhibited significantly higher pH values (3.65) compared to refrigeration treatments (3.03), similar to the pH averages of 3.38 reported by Reyes-Medina et al. (2017) in cape gooseberries subjected to different concentrations of CaCl2. It is noteworthy that refrigeration significantly reduces pH values by slowing down cellular respiratory metabolism. Likewise, when combined with the application of HC and calcium, it helps maintain low pH values.
On the other hand, when analyzing the pH variation in cape gooseberry fruits during the post-harvest phase, a decreasing trend was observed until 9 and 24 DAH in fruits stored in the environment and with refrigeration, respectively, at which point the trend changed and began to increase until the end of the postharvest at 19 and 33 DAH, respectively. This initial decrease in pH is also reported by Reyes-Medina et al. (2017) and can be attributed to the conversion of acids stored in the vacuole into sugars (Saltveit, 2019). Similarly, the subsequent increase in pH may be related to temperature increases that promote higher respiratory rates, leading to the loss of substrates, including acids (Reyes-Medina et al., 2017).
Respiratory rate (RR)
Cape gooseberry fruits exhibited significant differences in RR between treatments in most postharvest measurements except for 23 and 33 DAH (Fig 3). At the initiation of storage, fruits that did not undergo hydrocooling displayed higher RR values (7.16 mg kg-1 h-1 of CO2) compared to other treatments (2.91 mg kg-1 h-1 of CO2), emphasizing one of the main benefits of HC, which is the reduction of field heat, thereby lowering the temperature and metabolic respiration processes of recently harvested fruit (Makule et al., 2022), similar to findings in cherries with HC application (Wang and Long, 2015). It was also observed that fruits stored in room temperature conditions had a higher RR than those refrigerated until 19 DAH, at which point they lost their consumption quality, similar to observations in cape gooseberry by Balaguera-López et al. (2015). Additionally, RR increased from 16 and 14 DAH for treatments with and without refrigeration, respectively, peaking at 26 DAH for refrigerated fruits (7.22 mg kg-1 h-1 of CO2), where the application of HC, refrigeration, and 1% CaCl2 (+HC1.0CaCl24ºC) had the lowest RR values at 5.8 mg kg-1 h-1 of CO2. According to Alvarado et al. (2004), the climacteric peak can occur between 23 and 27 DAH, for fruits without and with calyx, respectively.
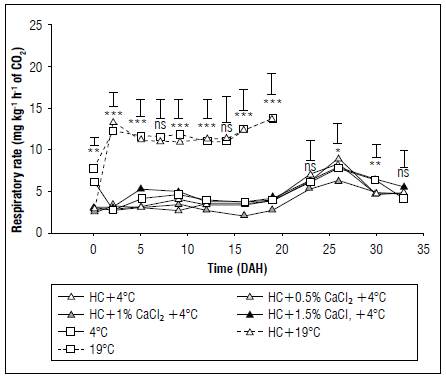
Figure 3. Effect of refrigeration, hydrocooling (HC at 4°C), and CaCl2 on the respiratory rate in cape gooseberry fruits during storage. ns: not significant. *, ** and *** indicate significant effect according to the Anova P≤0.05, P≤0.01 and P≤0.001, respectively. Vertical bars indicate minimum significant difference according to Tukey (P≤0.05).
Color index (CI)
Towards the end of storage (29 DAH), fruits subjected to HC, refrigeration, and doses of 0.5% (+HC0.5CaCl216ºC) and 1% CaCl2 (+HC1.0CaCl216ºC) displayed the lowest RR values, averaging 27% lower compared to fruits refrigerated at 4°C, highlighting the effect of HC and Ca2+ in slowing the respiration process. In this context, it is known that the application of Ca is involved in the synthesis of ethylene and the transduction of ethylene signals; likewise, it reduces and postpones the climacteric peak (Gao et al., 2019), as reported in peaches (Ali et al., 2021) and apples (Xu et al., 2022).
The CI did not exhibit significant differences between treatments during the initial 16 DAH, according to the Anova (P≤0.05). However, at 19 DAH, the CI displayed highly significant differences between treatments (Fig. 4). The highest values were observed in fruits stored at room temperature with and without HC (2.44), while refrigerated fruits had, on average, lower CI values (1.54), emphasizing the importance of refrigeration in delaying the color metabolism change in cape gooseberry fruits. There were significant differences between the different doses of CaCl2 at 19 DAH, where the 1% dose showed the lowest IC values, which implies that this application delays changes in coloration during storage. From 19 DAH onward, the CI maintained a consistent behavior until the end of the post-harvest. However, at 29 DAH, fruits with HC, refrigeration, and 0.5 and 1% CaCl2 (+HC0.5CaCl216ºC and +HC1.0CaCl216ºC) had statistically lower CIs, underscoring the significance of Ca2+ in delaying the color change of cape gooseberry fruits during post-harvest.
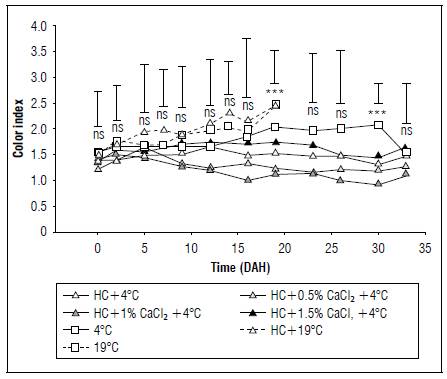
Figure 4. Effect of refrigeration, hydrocooling (HC at 4°C), and CaCl2 on the color index (CI) in cape gooseberry fruits during storage. ns: not significant. *, ** and *** indicate significant effect according to the Anova P≤0.05, P≤0.01 and P≤0.001, respectively. Vertical bars indicate minimum significant difference according to Tukey (P≤0.05).
In this context, the increase in CI is associated with the attainment of a dark orange color due to oxidation processes occurring during ripening, leading to the degradation of chlorophylls (greening) caused by chlorophyllases, peroxidases, and pheophytins (González-Locarno et al., 2020). Moreover, there is an increase in the synthesis of carotenoids, primarily β-cryptoxanthin and β-carotene (Ordóñez-Santos et al., 2017), serving as an indicator of the maturation process of cape gooseberry. The application of CaCl2 in post-harvest becomes crucial, as per Nassarawa et al. (2024), since Ca acts as a metabolic regulator, aiding in retaining the green pigments of fruits by inhibiting enzymes that degrade chlorophylls. Additionally, hydrocooling and refrigeration play pivotal roles in the post-harvest life of horticultural products, slowing down enzymatic and metabolic activities (Duan et al., 2020). This has been demonstrated in melons (Zainal et al., 2019), as hydrocooling kept the luminosity and tone of the skin constant for a longer duration, associated with a deceleration in the ripening process of the fruit. However, Reyes-Medina et al. (2017) found that applications of CaCl2 in cape gooseberry fruits did not affect CI values stored at room temperature.
CONCLUSION
Fruits subjected to hydrocooling (HC), refrigeration, and 1% CaCl2 application exhibited lower mass loss (ML), retained greater firmness, higher total soluble solids (TSS), and total titratable acidity (TTA), along with lower values of pH, reduced respiration rates (RR), and delayed color index (CI) increase. Hydrocooling positively influenced the maintenance of postharvest characteristics, extending the shelf life of refrigerated cape gooseberry fruits up to 33 days after harvest (DAH), with no discernible effect on fruits stored at room temperature (19 DAH). Refrigeration at 4°C played a crucial role in preserving TSS, TTA, and pH in cape gooseberry fruits while maintaining low values for the maturity ratio, RR, and CI.