Introduction
Eryngium caeruleum is one of the endemic plants of Iran with almost unknown potential that is neglected. It is an herbaceous perennial plant growing in northern Iran. E. caeruleum belongs to the family Apiaceae, represented by different species in the country. The plants of this family, especially of the genus Eryngium, could have a great potential for the future production of herbal medicines. This plant is used as a medicinal plant and as a vegetable. The leaves of this plant are used to flavor cooked vegetables in various local dishes and used in soups or consumed mixed with yogurt (Khoshbakht et al., 2007). Few studies have reported the content of total phenolic compounds and total flavonoids, and antioxidant properties of the alcoholic extract of the leaves (Dehghan et al., 2016). This plant is widely used in the medicine and food industry as a diuretic and appetite stimulant (Hashemabadi & Kaviani, 2011). The shoots and roots contain terpenoids, saponins, flavonoids, phenolic acids, polystyrene, and other biologically active compounds (Hashemabadi & Kaviani, 2011; Erdem et al., 2015). Various species of Eryngium contain numerous phytochemical compounds, and at least 127 compounds have been isolated and identified (Wang et al., 2012; Erdem et al., 2015). In addition, the plants of this genus contain various nutrients, including vitamins, minerals, and proteins (Paul et al., 2011).
Using chemical fertilizers in intensive agriculture may cause damage to plant production, soil health, and environment (Anwar et al., 2005). One of food security's most essential tasks is finding a suitable alternative to mineral fertilizers. The employment of these fertilizers has also increased climate pollution (Elser & Bennett, 2011; Tubiello et al., 2015; Bauer et al., 2016). Moreover, biodiversity has decreased significantly in agriculture due to habitat loss (Tilman et al., 2017). The microorganisms and bacteria in soil affect the cycling of mineral nutrients such as nitrogen, sulfur, and phosphorus (Kumar et al., 2018). Considering the beneficial microbial activities, biofertilizers have significant potential to increase the health and productivity of plants and reduce the need for synthetic fertilizers. Arbuscular mycorrhizal fungi (VAM) can form a symbiotic association with plants that benefits both partners through the acquisition and uptake of mineral nutrients, especially phosphorus, from the soil (Barea et al., 2011; Hoseinzade et al., 2016). Mycorrhiza, as a critical component in ecosystems, positively affects quantitative and qualitative characteristics of plants (Harrier & Watson, 2004; Gosling et al., 2006) Examples of the role of VAM in agricultural ecosystems include increasing the active surface area of the root system for better uptake of nutrients from soil, especially under phosphorus deficiency (Kapoor et al., 2007), increasing photosynthesis (Copetta et al., 2006), increasing resistance to drought, salinity, and resistance to pests and diseases (Feng et al., 2002; Pinior et al., 2005; Samarbakhsh et al., 2009), improving soil structure (Celik et al., 2004), and increasing the activity of N2-fixing bacteria (Antunes et al., 2006). The variable performance of mycorrhizal fungi depends on the host plant; many studies have reported the positive effects of symbiosis of plants with mycorrhizal fungi (Jansa et al., 2008; Pellegrino & Bedini, 2014: Derkowska et al., 2015; Palencia et al., 2015). Koozehgar Kaleji et al. (2021) found that using mycorrhiza and organic fertilizers increased the quantitative and qualitative yield of Nasturtium officinale.
Azotobacter is a free-living N2-fixing bacterium that produces various siderophores and can increase the absorption capacity of Zn, Fe, and Mo by plants, as well as the solubility of phosphorus from insoluble compounds; the use of these microorganisms constitutes one of the most effective ways to improve nutrient mobility and absorption (Mrkovacki et al., 2001). Azotobacter are free-living rhizobacteria that promote plant growth by producing auxins, gibberellins, and cytokinins, and making mineral nutrients, especially nitrogen, available to plants (Jnawali et al., 2015). Inoculation with different Azotobacter species increased nutrient availability and synthesis of biologically active compounds, thus, positively affecting plant growth and yield (Rojas-Tapias et al., 2012; Delshadi et al., 2017; Turan et al., 2017; Rodrigues et al., 2018).
Increasing planting density increases competition between plants for growth factors such as adequate space for branching and root growth and the acquisition of light, mineral nutrients, and moisture (Bairagi, 2014). Ahmad and Abdulla (2016) compared the effects of three planting densities (20x20 cm, 30x30 cm, and 40x40 cm) on fenugreek and observed the highest plant dry weight at the highest plant density (40x40 cm). This study evaluated the effects of the joint application of Azotobacter chroococcum and VAM with various plant densities on growth performance and shoot nutrient content of E. caeruleum.
Materials and methods
The experiment was conducted in 2016-2017 in Aboksar, a village in Mazandaran province (northern part of Iran) located at 53°62' E, 36°46' N, and an elevation of 17.3 m a.s.l. with moderate climate (Tab. 1) in a factorial randomized block design with five replicates with 685 plants per replicate and a total 3425 plants.
Factors included three levels of planting density of the E. caeruleum plants (10x30 cm, 20x30 cm, and 30x30 cm), two levels of seed inoculation with VAM fungi (without and with inoculation with Glomus mosseae, a soil-based inoculum of a local isolate, which consisted of 1200 spores/100 g of soil) and two levels of seed inoculation with Azotobacter chroococcum (without and with inoculation); a bacterial culture was produced by the Soil and Water Research Institute, Iran Ministry of Agriculture, using 0.5 L ha-1 at the time point (CFU=108). Seeds from wild plants were surface sterilized with 0.5% sodium hy-pochlorite for 3 min and then washed thoroughly before sowing. For the VAM inoculation treatments, a thin layer of 5 g of G. mosseae inoculum containing 1200 spores per 100 g of soil was spread 2 cm below the soil surface; then, the seeds were sown separately in each plot. A vermicom-post of 5 t ha-1 (animal origin) was calculated and applied on each plot. The size of each plot was 1.5x1.5 m2, and each plot was planted with six rows. The results of the loam soil (Entisols according to the USDA soil classification) and organic manure analysis are shown in Table 2. In the first stage, the basic tests include the determination of soil texture by the hydrometric method (Bouyoucos, 1962), organic carbon by the Walkley and Black method (Nelson, 1982), equivalent calcium carbonate by back titration method, and the electrical conductivity of saturated extract and pH of saturated soil paste (Page et al., 1982).
TABLE 2 Physical and chemical properties of experiment soil and chemical properties of vermicompost.

OC - organic carbon contents; OM - soil organic matter contents; EC - electrical conductivity.
Planting operations were carried out in November 2016, including irrigation, thinning, and weeding. Watering of the plants was done every 4 d. The plants were thinned to achieve adequate density at the 4-5 leaf stage (after the plants were fully established). Finally, the predefined plant density was kept constant in each plot. Vegetative organs were harvested to measure morphological characteristics and essential oil content at the end of the vegetative period (formation of the flowering stem). For this purpose, eight plants per plot were randomly selected, and leaf area and dry weight of leaves were measured and recorded separately. The relative chlorophyll content in plants was measured in leaves of the highest expansion at the pre-flowering stage using SPAD 502 PLUS. The selected plants of each plot were manually harvested and separately placed in the package and labeled. The harvested plants were dried for 10 d under natural conditions without exposure to sunlight. After crushing the leaves, 20 g of the leaf samples were mixed with distilled water in the Clevenger apparatus (Sina Glass, Tehran, Iran) to extract the essential oil. The extraction time for the essential oil was 3 h for all samples. After the oil was dehydrated with sodium sulfate, the oil content was determined as a percentage. To determine nutrient concentrations, the collected leaves were dried and pulverized with an electric mill and digested with sulfuric acid, salicylic acid, hydrogen peroxide, and selenium to prepare the extracts (Emami, 1996). The leaf area was measured using ImageJ software. The amount of essential oil was weighed with a balance of 0.0001 g and calculated as the percentage and essential oil yield in kg ha-1. Nitrogen content was determined using titration after distillation in the Kjeltec Autoanalyzer (Bremner & Mulvaney, 1982). The amount of phosphorus was analyzed by the colorimetric method (yellow molybdenite vanadate) (Emami, 1996). Foliar contents of iron, copper, zinc, manganese, and potassium were measured by atomic absorption, and potassium content was analyzed using a flame emission photometer Jenway™ PFP7 (Emami, 1996). Data were statistically analyzed by analysis of variance (ANOVA) using SAS software v 9.4, and mean comparisons were estimated at a probability level of 5% using the LSD test.
Results
Leaf dry weight
Analysis of variance (Tab. 3) showed that application of biofertilizer and the interaction of plant density and bio-fertilizer had a significant effect on the dry weight of leaves (P<0.01). The mean comparisons showed that the highest dry weights were 2.93 g when treated with VAM at a plant density of 30x30 cm (Tab. 4).
TABLE 3 Analysis of variance with the effects of biofertilizers on Eryngium caeruleum traits.
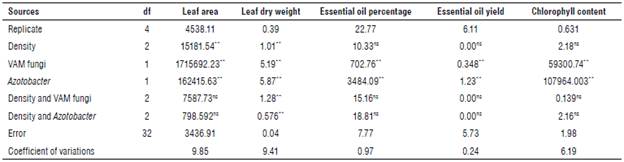
ns, * and **: non-significant and significant at 5% and 1% probability levels of LSD, respectively.
TABLE 4 Mean comparison effect of biofertilizers on Eryngium caeruleum traits.
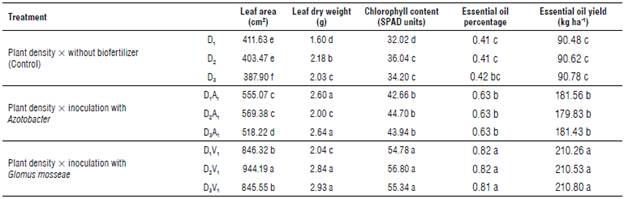
Means in each column followed by similar letters are not significantly different at the 5% probability level- using LSD Multiple Range Test. D1: plant density 30x10 cm, D2: plant density 30x20 cm, D3: plant density 30x30 cm (without biofertilizer).
D1A1: plant density 30x10 cm x inoculation with Azotobacter, D2A1: plant density 30x20 cm x inoculation with Azotobacter,
D3A1: plant density 30x30 cm x inoculation with Azotobacter. D1V1 plant density 30x10 cm x inoculation with G. mosseae,
D2V1: plant density 30x20 cm x inoculation with G. mosseae, D3V1 plant density 30x30 cm x inoculation with G. mosseae.
Leaf area
According to the analysis of variance (Tab. 3), the application of Azotobacter and VAM had a significant effect on leaf area (P<0.01), and density had a significant effect (P<0.01). Based on the mean comparison data, all fertilizer treatments increased leaf area compared to the control group.
The highest and the lowest leaf area was recorded in the VAM treatment (944.19 cm2) and the control group (403.47 cm2), respectively (Fig. 1).
Essential oil content
Analysis of variance (Tab. 3) showed that the VAM, and Azotobacter had a significant effect on essential oil content in leaves (P<0.01). The comparison of the mean interaction showed that the highest content was found in the treatments with VAM and plant density of 10x30 cm and 20x30 cm with 0.82% and the lowest in the control treatment with 0.41% (Fig. 2).
Essential oil yield
The results of the analysis of variance (Tab. 3) showed that the different fertilizer treatments had a significant effect on the essential oil yield of E. caeruleum (P<0.01). A comparison of mean values showed that the VAM treatments gave the highest oil yield with an average of 210.53 kg ha-1, and the control treatment gave the lowest with an average of 90.48 kg-1 (Fig. 3).
Chlorophyll content
The results of the analysis of variance (Tab. 3) showed that the application of biofertilizer had a significant effect on the chlorophyll index (P<0.01). Plant density and its interaction with biofertilizer did not affect relative chlorophyll content. The mean comparison results showed that the highest and lowest chlorophyll content were found in the VAM treatment and control treatment at 56.80 and 32.02, respectively (Fig. 4).
Mineral nutrient uptake
Analysis of variance (Tab. 5) showed that the application of biological fertilizers significantly increased the nutrient content in E. caeruleum (P<0.01). The mean comparison results showed that the highest and lowest nitrogen content was found in the Azotobacter treatment and control treatment with 0.71% and 0.51%, respectively (Fig. 5). Similarly, the highest and lowest phosphorus contents were found in the VAM treatment at 0.19% and the control treatment at 0.08% (Fig. 6). Compared to the control treatment, inoculation with VAM and Azotobacter increased the con- 0.11%. The average comparison also showed that the VAM centration of iron, manganese, zinc, and copper by 1749.5, treatment had the highest contents of potassium (0.599%) 180.9,25.22,14.02 mg kg-1, respectively, and magnesium by and calcium (2.88%) (Tab. 6).
TABLE 5 Analysis of variance with the effects of biofertilizers on foliar nutrient content of Eryngium caeruleum.
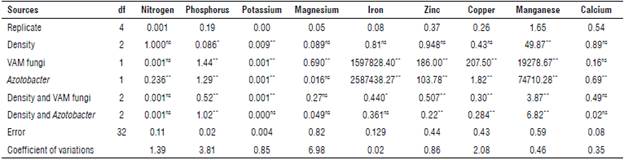
ns, * and**: non-significant and significant at 5% and 1% probability levels, respectively.
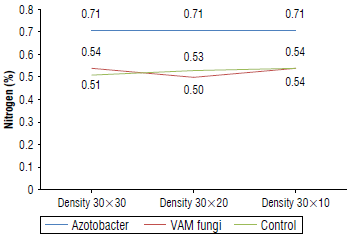
FIGURE 5 Foliar nitrogen contents of Eryngium caeruleum after applying biofertilizers at three planting densities (cm).
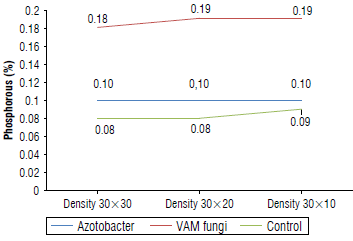
FIGURE 6 Foliar phosphorus contents of Eryngium caeruleum after applying biofertilizers at three planting densities (cm).
TABLE 6 Mean comparison of nutrient content in leaves of Eryngium caeruleum under the influence of biological fertilizer application at different planting densities.
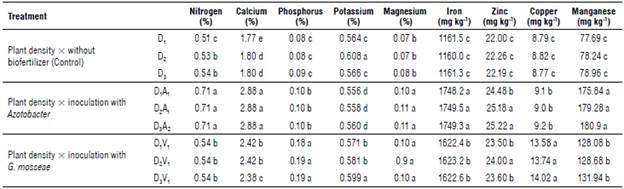
Means in each column followed by similar letters are not significantly different at the %5 probability level using LSD Multiple Range Test. D, plant density 30x10 cm, D2 plant density 30x20 cm, D3: plant density 30x30 cm.
DA: plant density 30x10 cm x inoculation with Azotobacter, D A: plant density 30x20 cm x inoculation with Azotobacter,
D3A2: plant density 30x30 cm x inoculation with Azotobacter. D,V, plant density 30x10 cm x inoculation with G. mosseae, D2V,: plant density 30x20 cm x inoculation with G. mosseae, D3V,: plant density 30x30 cm x inoculation with G. mosseae.
Discussion
The application of VAM and Azotobacter significantly affected the characteristics of E. caeruleum, including morphological characteristics and dry weight, leaf area, chlorophyll content, percent essential oil, essential oil yield, and nutrient uptake (Tab. 3). Vermicompost with high porosity can absorb and store considerable amounts of minerals and release them gradually. In addition, its high water-holding capacity (Arancon et al., 2004) affects the growth of the studied plants. Furthermore, the application of vermicompost, VAM, and Azotobacter increases the growth of the plants and causes a significant difference compared to the control group due to the release of macro and microelements, vitamins, enzymes, and growth-promoting hormones, as well as the increase in the number of efficient soil microorganisms. As shown in Tables 5 and 6, the application of VAM, Azotobacter, and vermicompost improved the morphological characteristics compared to the control group. Table 4 also shows that all measured traits improved when treated with VAM and Azotobacter. The results of this study are consistent with the findings of Koozehgar Kaleji and Ardakani (2018) and Koozehgar Kaleji and Ardakani (2019), who reported an increase in leaf area, essential oil percentage, essential oil yield, and dry leaf weight of E. caeruleum M. Bieb and Froriepia sub-pinnata with the use of organic fertilizers and mycorrhiza. Mycorrhizal symbiosis of fungi with plants can increase nutrient uptake by the plants and yield (Chen et al., 2017). Other experimental results on tomato plants induced by VAM inoculation showed that leaf area and nitrogen, potassium, calcium, and phosphorus content increased (Balliu et al., 2015). Another study observed that the application of Azotobacter and mycorrhiza increased the chlorophyll content of the plant Prosopis chilensis ([Molina] C.E. Hughes & G.P. Lewis) compared to the control (Faramawy, 2014). Determining the optimum plant density could be critical for plant growth and yield. Optimal plant density prevents inter-plant competition and increases product and final dry matter by increasing light, nutrient, and water uptake efficiency and reducing evapotranspiration. The increase can be attributed to the uptake of nutrients, especially phosphorus (Larimi et al., 2014). Other studies have shown that G. mosseae increases the essential oil content of Origanum majorana (L.) (Khaosaad et al., 2006). Also, two species of mycorrhizal fungi (Glomus fasciculatum [Rhizoglomus fasciculatum (Thaxter) Sieverding, G.A. Silva & Oehl], Glomus macrocarpum Tul. & C. Tul.) increased the growth and essential oil concentration of fennel (Kapoor et al., 2004) and coriander (Kapoor et al., 2002). Using biofertilizers increased the yield and essential oil content of Ocimum basilicum L. (Al-Mansour et al., 2018). Since the essential oil yield is related to the percentage of essential oil and the dry weight of the plant, any increase in these two parameters may result in a higher yield of oil. Rueda et al. (2016) showed that inoculation of Azospirillum and Azotobacter with Fragaria vesca L. increased plant height, dry weight of roots and shoots, leaf area, chlorophyll content, nutrient content, and plant yield. Tang et al. (2009) found that maize inoculated with G. mosseae had higher chlorophyll synthesis, significantly improving plant photosynthesis. This improvement was attributed to increased nitrogen uptake by inoculated plants. Inoculation of A. chroococ-cum, Pseudomonasputida (Trevisan), Bacilluspolymyxa or Paenibacillus polymyxa (Prazmowski), and VAM significantly increased root and shoot biomass, chlorophyll, and NPK content in plants (Vafadar et al., 2014). The results on phosphorus, copper, zinc, and manganese agree with the results of Koozehgar Kaleji and Ardakani (2018). Another study showed that the effect of mycorrhizal inoculation on the concentrations of iron, manganese, and copper was significant (P<0.01); inoculation with VAM fungi increased the concentrations of nutrients such as iron, manganese, zinc, and copper by 142, 67.6, 21, and 12 units, respectively, compared to the control treatment (Ortas & Bykova, 2018). The results of this study are consistent with the findings of Zhang et al. (2016), who found that inoculation of mycorrhiza increased phosphorus contents in plants. Root colonization by VAM and bacteria can increase root length and surface area, thus, increasing water and nutrient uptake, especially under drought conditions (Marulanda et al., 2006; Marulanda et al., 2009). Other research results showed that inoculation with mycorrhizal fungi increased the absorption of nitrogen, phosphorus, potassium, zinc, iron, and copper in sesame plants compared to the control (Askari et al., 2018).
Conclusions
Application of VAM and Azotobacter treatments at different plant densities increased the dry weight of leaves, essential oil yield, leaf area, and oil content. In addition, increasing plant density increased yield components. Increases in yield and yield components of E. caeruleum due to the use of a biological fertilizer can be attributed to the increased absorption of nutrients, such as nitrogen, phosphorus, potassium, and a microbial population in the rhizosphere in the soil. Inoculating the seed with growth-promoting bacteria apparently increased the growth of E. caeruleum by creating a cycle that provided nutrients through the production of fungal mycelia, which increased nutrient uptake by the roots and indicated enhanced microbial activity in the soil. Additional tests showed that mycorrhizal symbiosis, vermicompost and Azotobactor applications increased plant growth and yield. The study show that the combination of low-input systems, free-living nitrogen-fixing bacteria, and VAM could be a suitable alternative to chemical fertilizers and complete fertilizers, especially in low-input systems or organic farming.