The use of agrochemicals in the control of pests and diseases in agriculture is a common practice due to the high incidence of various phytopathogens. In strawberry crops, the incidence of Botrytis cinerea is considered a major pathogen, exerting significant economic impacts both pre- and post-harvest, resulting in potential losses of up to 50% of production (Petrasch et al. 2019). While agrochemicals exhibit efficacy under certain circumstances, their application under suboptimal management conditions poses detrimental effects on human health, environmental integrity, and non-target organisms. Most chemical synthesis pesticides do not degrade easily, consequently, they accumulate in the environment, which causes contamination of soil, water, and air (Lengai et al. 2020). Moreover, for a pathogen of high risk as B. cinerea, the development of resistance to diverse fungicide agents has been reported, for example, benzimidazoles and dicarboximides (Avenot et al. 2020) underscores the inadequacies of synthetic pesticides.
The disadvantages associated with the inappropriate and excessive use of synthetic pesticides have catalyzed the exploration of alternative strategies for pest and disease management. Biopesticides based on plant extracts or essential oils constitute an alternative, due to the presence of bioactive compounds of lower toxicity and easily biodegradable (Avenot et al. 2020). For example, saponins are a group of molecules with antifungal, antibacterial, insecticidal, molluscicide and vermicide properties, synthesized by an important number of plants such as those belonging to the Chenopodiaceae and Amaranthaceae families (Ruiz et al. 2017), represent viable candidates for biopesticide formulations.
The quinoa plant (Chenopodium quinoa Willd) is a natural source of triterpenoid saponins, which are found in leaves, stems, flowers, and seeds. Of these structures, the seeds present the highest content of saponins, what are mainly located in the outer layer named the pericarp (Ruiz et al. 2017). However, within the food industry, the presence of saponins in quinoa seeds poses technological challenges, necessitating husk removal through abrasive processes to mitigate saponin content (Jiang et al. 2021), as a consequence, a by-product with an important content of saponins is generated, which is undervalued, leading to the under exploitation of its biological potential.
In this sense, the use of saponins obtained from by-products of the industrial processing of quinoa presents an environmentally sustainable strategy for developing biopesticides effective against diseases such as those associated with B. cinerea. The aim of this study was to evaluate the in vitro and in vivo antifungal effect of saponins extracted from quinoa husks, using the crude extract, and incorporated in a formulation against B. cinerea in strawberry.
MATERIALS AND METHODS
Plant material
Quinoa husks: was collected from the Green Line Origin Products S.A.S located in Popayán, Colombia. The material collected came from local quinoa cultivars of sweet varieties, according to the suggested for batches of 50 to 500 kg (Rag et al. 2019). A representative sample was sieved through a number 70 sieve using a FRITSCH Analysette 3 Rotap equipment (Idar-Oberstein, Germany), to obtain a by-product fraction with a particle size less than or equal to 212 µm (Huaman and Shuan 2018). The material obtained in this stage was used in later studies.
Strawberry fruits: were obtained from a commercial crop located in the municipality of Silvia, Colombia. The collection was done randomly, and the quantity was 500 g of fruits of third classification, in stage three of maturity, as established by the Colombian Technical Standard NTC 4103 of 1997 for strawberry variety Chandler.
Physicochemical characterization of quinoa husks
Quinoa husks were characterized by proximal analysis and total content of saponins.
Proximal analysis
The proximal analysis of the quinoa husks was determined in terms of moisture content, ash, protein, fiber, and ethereal extract, following the AOAC method, (1990) (920.151, 942.05, 984.13, 985.29, and 920.39, respectively). Carbohydrates were calculated by difference.
Total content of saponins
The total saponin content of quinoa husk was determined by applying the microwave extraction and maceration methods, according to the methodology described by Le et al. (2018), Villacis (2018) and Guilcapi (2019), with some modifications. In a flask, 5 g of quinoa husk (particle size ≤212 µm) were weighed then mixed with 100 mL of 47% ethanol and homogenized in a magnetic agitation plate for 30 s, subsequently, the sample was taken to a conventional microwave oven (Samsung AMW831K/XAP, Suwon, South Korea). The sample was subjected to seven irradiation cycles of 20 s each, with a cooling time of 1 min between cycles. Then, the sample was taken to a heating plate and magnetic agitation for 22 min. The temperature was maintained at 50 °C and the agitation at 200 rpm. Once the extraction process was finished, the liquid fraction was separated from the solid fraction by vacuum filtration, then the ethanol was recovered from the liquid fraction by simple distillation. The extract was stored and the solid was subjected to two additional extractions by applying the previously described process. Finally, three extracts were obtained and the concentration of saponins was quantified for each one by UV - VIS spectrophotometry applying the method described by Gianna (2013). The total saponin content was established as the sum of the three concentrations quantified in the extracts and expressed as a percentage dry basis.
Optimization of the extraction process
Quinoa husk was subjected to different extraction conditions by maceration method. The percentage of ethanol in the solvent (50 and 80%), temperature (50 and 60 °C), and extraction time (1 and 3 h) were the variables evaluated. The optimization study of the saponin extraction process was carried out by applying the response surface methodology (RSM), under a central composite design (CCD), for which two levels and three independent factors were defined, including ethanol concentration (X1), time (X2) and temperature (X3) in a factorial arrangement 23. The total number of experimental runs generated by the design was 20, with eight cube points, four central points in the cube, six axial points, and two central points in the axial (Table 1). For the axial points, an α=1.633 was used. The experiments were run randomly. The yield of extraction was calculated for the optimal conditions found by equation 1:
Where SE is percentage (%) of extracted saponins, and ST is percentage (%) of total saponin.
Minitab Software version 2019 was used, the statistical analysis of the model was performed through analysis of variance (ANOVA) with a significance level of 95%; to identify the effects of the factors on the response variable and the model fit, which was corroborated based on the P-value and R-squared. Confirmation runs were performed in triplicate after response surface analysis to verify the accuracy of the optimal condition predicted by the model. To verify the statistical equality of the predicted and experimental saponin percentage, a t-test was run at a 95% significance level.
Determination of saponins content by LB reaction
The determination of saponins was done as described by Gianna (2013). Briefly, the extract was diluted with 96% ethanol at a ratio of 1:10 (extract: ethanol). 1 mL of this solution was mixed with 3.5 mL of LB reagent, the mixture was shaken for 30 s and allowed to stand for 30 min, with two shakings in between. At the end of the reaction time, the absorbance was measured at 417 nm and the results were expressed as a percentage of saponins on a dry basis.
Isolation and identification of the fungus Botrytis cinerea
The methodology described by Apolonio et al. (2017), Marín et al. (2017) and Isaza et al. (2019) was applied. Strawberry fruit infected with B. cinerea were harvested from a commercial strawberry crop in Silvia, Colombia. The fruits were disinfected with 1% sodium hypochlorite, washed three times with distilled water and dried with absorbent paper, subsequently small slices of diseased tissue were obtained, and four tissue fragments were seeded in Petri dishes with potato dextrose agar (PDA) and incubated at 25 °C for 3 days. The developed colonies were purified in a PDA solid medium using the hyphae tip technique. The morphological identification of the isolates was performed based on the macroscopic and microscopic characteristics of B. cinerea. Macroscopic identification was verified by the appearance, texture, and color of the colonies. For the microscopic description, the isolates were observed in a trinocular microscope through the imprinting method. Additionally, a digital image was taken by scanning electron microscopy equipment (TESCAN VEGA3 LM, Brno, Czech Republic) at an acceleration voltage of 10 kV.
Determination of Minimum Inhibitory Concentration (MIC)
The minimum inhibitory concentration of the saponin extract against the phytopathogen B. cinerea was determined by using the agar dilution technique described by Ruiz (2000). Five concentrations of saponin were obtained by serial double dilution: 8.25, 4.125, 2.0625, 1.0312, and 0.5156 mg mL-1. These solutions were prepared from a concentrated extract. Under aseptic conditions, 2 mL of each solution was poured into a Petri dish, then 18 mL of sterilized PDA cooled to 50 °C was added. The dishes were shaken gently until the extract and the culture medium were homogenized. Once the medium had solidified, 10 µl of a spore solution standardized to 1x104 spores mL-1 obtained from an 11-day-old culture of B. cinerea was inoculated. The spore solution was dispersed over the culture medium. A blank was prepared by mixing 2 mL of distilled water with 18 mL of PDA and inoculated with 10 µL of the spore solution.
Incubation was carried out at 25 °C for 76 h, then the spores that germinated on each plate were counted. The MIC was determined as the concentration at which no spore germination was observed. All assays were performed in quintuplicate.
Preparation of saponins formulation
Three emulsion-type formulations were prepared, whose components and their proportions were defined based on the studies conducted by Campolo et al. (2020). The formulations included saponins extract, vegetable oil, and polysorbate 80 and were standardized at three saponin concentrations: 4.12, 2.062, and 1.0312 mg mL-1. To prepare them, the procedure described by Campolo et al. (2020) was used as a reference.
Evaluation of antifungal activity of formulations against Botrytis cinerea under in vitro conditions
The determination of the growth inhibition percentage (% GI) of the formulation obtained against B. cinerea, was made based on the methodology developed by Apolonio et al. (2017), Saha et al. (2018) and Yang et al. (2020). Under aseptic conditions, 2 mL of the formulation was added in a Petri dish, mixed with 18 mL of sterilized PDA, and cooled to 50 °C, and the Petri dish was gently shaken to homogenize. Once the medium solidified, a 5 mm diameter disc obtained from the edge of a 10-day B. cinerea culture was inoculated. A blank and a control (formulation additives and absolute PDA, respectively) were also included. The Petri dishes were incubated at 25 °C and kept under observation until the blank covered the entire surface of the medium, at which time the diameter of the fungal colony developed in each treatment was measured, the measurements were made with a tape measure and crosswise, averaging the two measurements obtained. All tests were performed in triplicate. The percentage of growth inhibition (% GI) was calculated with equation 2.
Where "X" is radial growth (mm) in the blank, "Y" is radial growth (mm) in the treatment.
A completely randomized experimental design (CRD) was applied with three treatments corresponding to the concentration of the formulation, a control treatment, and a blank (additives of the formulation and absolute PDA). The response variable was the percentage of inhibition of pathogen growth. Data were analyzed with Minitab software version 2019, by analysis of variance (ANOVA) (P<0.05) and comparison of means by Tukey's method (P<0.05).
Evaluation of antifungal activity of formulations against Botrytis cinerea under in vivo conditions
The formulation with saponin extract more effective (EF) in the in vitro evaluation was studied, and two additional treatments were included, which corresponded to diluted EF and Carbendazim (methyl benzimidazole-2-ylcarbamate) as chemical control. The concentration of the chemical control was defined as 0.1 mg mL-1 and diluted EF was established according to what was reported by Taborda et al. (2015) for the traditional application of commercial products. Therefore, the concentrations of each treatment were defined as: 4.125 and 0.5 mg mL-1 of saponins for EF and diluted EF. The procedure described by Taborda et al. (2015) and Yang et al. (2020) was followed, with some modifications. Randomly, four groups of washed and disinfected strawberries of five units each were formed. A hole of 2 mm wide by 2 mm deep was made in the central part of each fruit (equatorial location). Subsequently, the fruits were immersed in their respective treatment for 5 s, then these were placed on a tray and taken to a shaker incubator for drying at 30 °C for 30 min. Later, fruits were inoculated with small agar blocks with mycelium of a 12-day B. cinerea culture in the holes made. Afterward, the fruits were placed in glass jars and were left in observation for 96 h at room temperature. The inoculated blocks in each fruit were removed after 24 h (Yang et al. 2020). All assays were done in triplicate. Once the observation time was finished, the measurement of the diameter of the infection caused by B. cinerea was done by taking two measurements in a crossed way, which were averaged to determine the diameter of the lesion. To calculate the control percentage of each treatment, equation 3 was used.
Where "Bd" is the diameter in the blank (mm), and "Td" is the diameter in the treatment (mm).
A completely randomized design (CRD) was applied with three treatments corresponding to EF, diluted EF, and chemical control. The response variable was the percentage of control. Data were analyzed with Minitab software version 2019, by analysis of variance (ANOVA) (P<0.05) and comparison of means by Tukey's method (P<0.05).
RESULTS AND DISCUSSION
Physicochemical characterization of quinoa husks
The physicochemical characterization of the quinoa husk is shown in Table 2. The moisture content (6.52% w/w), ethereal extract (9.52% w/w), and fiber (8.81% w/w); were similar to those reported by Suárez-Rivero et al. (2019) and Paniagua et al. (2020). However, the ash (10.01% w/w) and protein (6.87% w/w) contents slightly deviate from those found by Huaman and Shuan (2018) and Paniagua et al. (2020). These results underscore that quinoa husk is a by-product rich in essential nutrients. The composition of carbohydrates, protein, and lipids in the husk may be attributed to the abrasion generated during quinoa seeds processes, where abrasive methods partially reduce the embryo, causing a loss of nutrients reflected in the resultant scarified quinoa seed by-product (Jiang et al. 2021).
The total saponin content in the husk is 3.33% on a dry basis (Table 2). In relation to this result, variable contents of saponins in quinoa husk have been reported, with values ranging from 1.89 to 22% by weight (Lozano et al. 2012; Huaman and Shuan 2018), which depends on the quinoa variety from which it is obtained; in general, bitter varieties have higher saponin content while sweet varieties have lower levels (Ruiz et al. 2017). The result of the present study corresponds to the previous postulate, since quinoa husks from sweet varieties were used.
Evaluation of saponins extraction process
The analysis of variance (ANOVA) of the experimental data of saponin extraction shows that the P-value of the quadratic model was less than 0.05 and the R-value was 0.8872 (Table 3), which indicates that the model is significant for the experimental data at 95% confidence level. The results show that only the ethanol percentage is significant for both the linear and quadratic models. Temperature and time were not significant for the regression model. Lack of adjustment measures the reliability of the equation to represent the data of the experimental points that are not included in the regression, if the P-values are lower than the significance level, the model does not fit the data well (Ramli et al. 2019). Therefore, the 0.377 value of lack of fit obtained with the quadratic model confirms that the model can give a satisfactory prediction of the percentage of extracted saponins (Table 3). Equation 4 shows the quadratic model generated and used for the surface response analysis, which predicts extractable saponins percentages through varying levels of assessed variables.
The extraction yield was higher when moderate process conditions were applied, which is evident in the contour plots (Figure 1). Between 45 and 65% ethanol and up to 60 °C, the percentage of saponins is higher than 2% (Figure 1A); time versus ethanol percentage shows a saponin yield higher than 2.4% with extraction times and ethanol concentration up to 0.65 h and 60%, respectively (Figure 1B). In Figure 1C, it is observed that between 60 °C and 0.75 h the proportion of extracted saponins is between 2.2 and 2.3%.
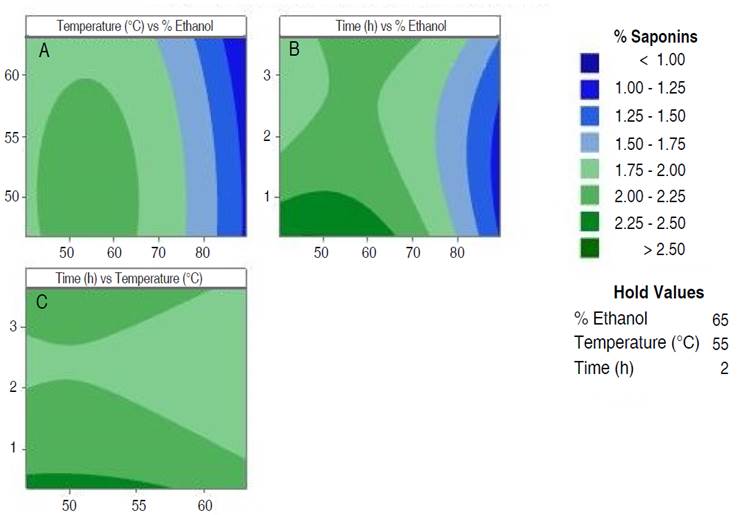
Figure 1 Contour plots of extraction process optimization. A. Temperature vs % ethanol; B. time vs % ethanol and C. time vs temperature.
According to Figure 1, the best extractions are achieved with ethanol percentages between 40 and 60%, temperature lower than 60 °C and time lower than 1 h. To verify this behavior, the optimal point conditions predicted by the software were applied. The prediction was 2.54% of saponins with 47.43% of ethanol, 49.80 °C and 0.367 h. The confirmation runs of the optimal condition, showed an experimental value of 2.47% of saponins, exhibiting no statistical difference (P<0.05). Furthermore, was established that the extraction yield at the optimum point was 74.23%, which represents a significant yield using a simple method and ecological solvent.
In general, the proportion of ethanol in the solvent was the most influential variable in the extraction process, obtaining the highest yield with an ethanol proportion of 47%, this result agrees with the reported by Lozano et al. (2012), Huaman and Shuan (2018), and Villacis (2018), who found the best extraction yields with ethanol proportions between 40 and 50%. However, it is observed that at proportions higher than 80% of ethanol, the extraction is reduced. Consequently, this behavior implies the unfeasibility of anhydrous ethanol as a solvent for the extraction of saponins from quinoa husks, this behavior is probably due to the modification of the degree of polarity of the solvent, since, when increasing the aqueous fraction, the polarity increases and when increasing the ethanolic fraction, the polarity decreases. Therefore, intermediate ethanol concentrations would provide a degree of polarity favorable to the solvent for the solubilization of polar compounds and therefore better extractions of the compound of interest (Hikmawanti et al. 2021).
Isolation and morphological identification of the fungus Botrytis cinerea
The macroscopic and microscopic observation of the isolations allowed to identify the morphological characteristics of the phytopathogen B. cinerea. It was found that the observations corresponded to the characteristics reported by Isaza et al. (2019). Thus, in the isolations, branched and septate mycelium was observed, which presented a whitish to grayish coloration. The conidiophores were observed emerging directly from the mycelium, and these were thin and of radial and vertical growth, and irregularly branched in the terminal portion where these presented groups of conidia in the form of clusters. The conidia were smooth and elliptical in shape (Figure 2). In some cultures, the formation of black sclerotia of round and/or irregular shape was observed.
Determination of Minimum Inhibitory Concentration (MIC)
According to the results (Table 4), no spore germination was observed at 8.25 mg mL-1 of saponins, which corresponds to a final concentration in the Petri dish of 0.825 mg mL-1, so this was determined as the MIC of the extract of saponins against the phytopathogen evaluated. There are few studies in which the determination of the MIC of quinoa saponins against fungal phytopathogens has been carried out. The study carried out by Stuardo and San Martín (2008) report the antifungal activity against B. cinerea of crude and hydrolyzed saponin extracts obtained from quinoa peel. It is indicated that MIC was not found in the range of concentrations evaluated (1-7 mg mL-1) with the extract of saponins in its crude form. On the other hand, triterpenoid saponins obtained from diverse vegetable sources have also been studied in the control of B. cinerea. The MIC of commercial saponin from Quillaja saponaria and the fruit of Sapindus mukorossi was 30 and ≥250 mg mL-1, respectively (Fischer et al. 2011; Porsche et al. 2018), which quite distant from the results found in the present investigation.
In vitro evaluation of formulation including saponin extract
After 9 days of incubation at 25 °C, the formulations inhibited mycelial growth of B. cinerea according to saponin concentrations. The inhibitions of the products containing saponins were higher than 90% (Figure 3). At the highest concentration (4.125 mg mL-1) an inhibition of 99.22% was achieved. The percentage of control was significantly different (P<0.05) among the evaluated treatments. Inhibition was also observed with the formulation without saponins (FWS), which reduced mycelial growth of B. cinerea by 60% (Figure 3).
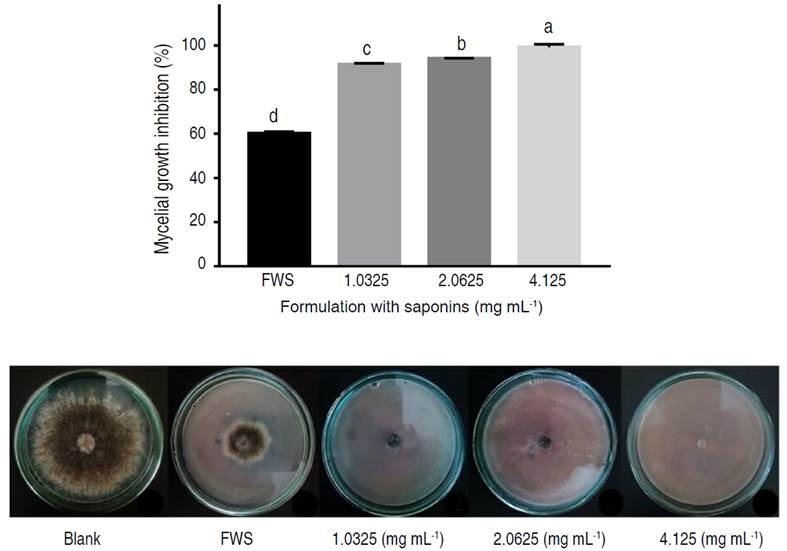
Figure 3 In vitro antifungal activities of the biopesticide formulation with saponin extract against mycelial growth of B. cinerea.
Antifungal inhibition tests were carried out on the formulation additives, where it was found that the surfactant polysorbate 80 inhibited the mycelial growth of B. cinerea, against this behavior, it has been reported that emulsions based on polysorbate 80 present antagonism against spore-forming fungi such as Aspergillus niger (Kaur and Mehta 2017). From the above, it can be deduced that the percentage of in vitro inhibition of mycelial growth of B. cinerea exhibited by the formulated product is caused by a combined effect of quinoa saponins and polysorbate 80. For instance, for the treatment with the highest saponin concentration, the inhibition attributable to saponins is 39.22%, considering the 60% inhibition generated by the formulation without saponins.
No research is reported in which saponins are incorporated into a formulation and their antifungal effect is evaluated; instead, inhibition of B. cinerea has been studied using saponin extracts from quinoa and other plant sources, in their crude form. For instance, Stuardo and San Martín (2008) and Mccartney et al. (2019) found that inhibitions of 32 and 43.7% were achieved with 5 mg mL-1 of saponins, respectively. Similarly, Fischer et al. (2011) reported an inhibition of 36% with 4 mg mL-1 of Quillaja saponin, while saponins obtained from the pericarp of the fruit of Sapindus mukorossi inhibited the growth of B. cinerea by 36% with a concentration of 5 mg mL-1 of saponin (Porsche et al. 2018). These findings indicate consistency between the results of this study and those reported in existing literature.
The toxic effect of saponins against fungi is due to the formation of pores and loss of cell membrane integrity. In the case of triterpenoid saponins, the group to which quinoa saponins belong, the mechanism involves the insertion of aglycones into the membrane, binding to sterols, followed by the association of sugar residues and the formation of the sterol-saponin complex, ending with pore formation and cell lysis (Zaynab et al. 2021).
In vivo evaluation of formulation including saponin extract
Figure 4 shows that the EF treatment presented a significantly different percentage of control compared to the diluted EF and the chemical control. The development of the infection caused by B. cinerea was reduced by 76.14±2.2% with the formulation containing 4.125 mg mL-1 of saponins (EF), while its diluted form (0.5 mg mL-1 of saponins) reached 63.03±6.3% of control, showing no significant difference to the control expressed by the chemical treatment (0.1 mg mL-1 of methyl benzimidazole-2-ylcarbamate). Observation throughout the experiment revealed the aggressiveness of the infection caused by B. cinerea on fruits without application of the controllers. The reduction in disease severity after application of the formulation is evident, and despite the slight reduction in efficacy after dissolution, the product continues to show inhibition over the pathogen.
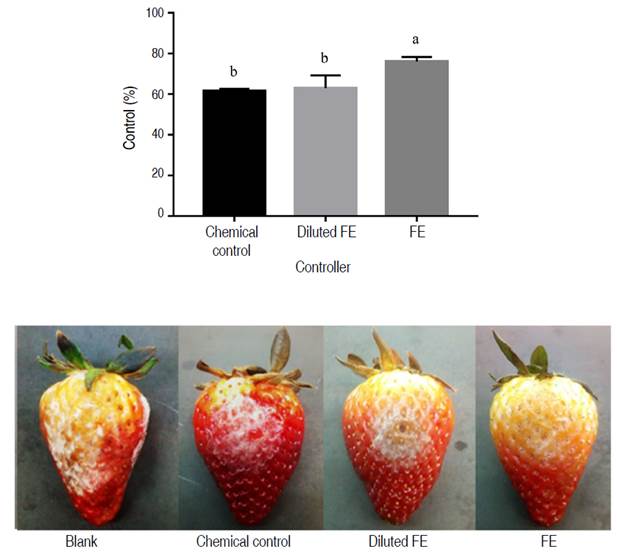
Figure 4 Efficacy of the biopesticide formulation at 4.125 mg mL-1 saponins (FE) and diluted to 0.5 mg mL-1 saponins (diluted FE), compared to the commercial fungicide Carbendazim (0.1 mg mL-1); on the severity of disease caused by B. cinerea in strawberry fruits.
The results were consistent with those obtained in the in vitro evaluation. In both experiments the percentage inhibition of the formulations on B. cinerea was significant; however, there was a reduction in the in the in vivo evaluation. These results are attributable to the influence of environmental factors on the development of gray mold caused by B. cinerea and in the case of infections in strawberry, there is a dependence on the maturity of the fruit (Petrasch et al. 2019). In effect, conditions such as temperature and humidity change in the in vivo evaluation, and the strawberry acts as culture medium, where the pathogen can have its nutrients for its development. Therefore, contribute to the observed fluctuation in antifungal efficacy across experiments.
There are no reports in the literature of research evaluating a formulation with saponins as an active ingredient against phytopathogens in vivo. In contrast, there have been studies evaluating other plant extracts and essential oils, crude or formulated, against B. cinerea, for instance, Yang et al. (2020) reported a 71.9% control of B. cinerea development on strawberries by the effect of tobacco cembratrien-diols (CBT). It should be noted that CBTs are diterpene compounds, molecules that bear some similarity to triterpene saponins.
Hence, the potential of bioactive compounds of plant origin for the control of phytopathogenic fungi of high impact in agriculture is evident. Saponins obtained from quinoa processing by-products are positioned as a viable alternative for the control of fungal diseases that affect crops and agricultural products.
CONCLUSION
The study of saponin extraction allowed obtaining a yield of 74.23% using an ecological solvent and a simple method, a process that was a key step, since it allowed obtaining extracts with a high concentration of saponins for the antifungal evaluation. The biocontrol capacity of quinoa saponins against Botrytis cinerea was determined through in vitro and in vivo tests; the minimum inhibitory concentration (MIC) was 8.25 mg mL-1 and the in vitro evaluation of the formulation reached inhibitions higher than 90%; however, it was observed that polysorbate 80, used in the formulation, also presented an antifungal effect, so that the inhibition attributable to the saponins of the most effective formulation was 39.22%. On the other hand, in vivo evaluation of the antifungal effect of the formulation exhibited higher and equal control percentages to the chemically synthesized fungicide Carbendazim: at 4.125 and 0.5 mg mL-1 of saponins the control percentage was 76.14 and 63.03%, respectively, while Carbendazim fungicide achieved 61.5% control. The results of this study show that quinoa saponins can be a viable option for the control of fungal diseases affecting crops and agricultural products, as well as an alternative for the development of new formulations based on bioactive compounds, which allows for the reduction of environmental impacts and the revaluation of quinoa agroindustry waste.