1. INTRODUCTION
Non-conventional reservoirs (YNC as per its Spanish acronym) propose a new energetic perspective focussed on worldwide energy self-sufficiency; this is achieved through the optimization and massification of the extraction of resources such as Shale Oil and Shale Gas. These type of oil reservoirs have very particular characteristics, making them very difficult prospects to manage. These source rocks contain significant amounts of organic matter in the form of kerogen, showing a low to ultra-low permeability. Hydraulic Fracturing is required to obtain these non-conventional resources; their main objective is the enhancement of the hydrocarbons flow through the creation of the fracture in the formation, connecting the reservoir with the well. (Nolen-Hoeksema, 2013).
To develop shale oil & gas resources in Colombia is necessary overcome several challenges, especially environmental issues, mainly the related to water consumption required for the hydraulic fracturing process and the flowback water management. Hydraulic fracturing in shale reservoirs demand greater amounts of water with respect to the hydraulic fracturing in sandstone reservoirs.
The implementation of technologies for the optimization of stimulation process has been much needed for the oil industry. Therefore in recent years a number of facilities have been set up allowing the transformation of those residual water volumes into fundamental constituents of fracture fluids to be used at later stages. Discussed methodology in this research work will allow to select the more appropriate technology (ies) for the treatment of returned waters in any non-conventional reservoirs in Colombia, taken into account the experience in efficiency and the cost of average treatments in the more important Shale plays of the United States.
2. THEORICAL FRAME
Hydraulic Fracturing Overview
Hydraulic Fracturing is a well stimulation technic starting its industrial use in 1949; it was also used for the enhanced hydrocarbon recovery (Gandosi, 2013). The hydrocarbon production of source rocks is more complicated than reservoirs rocks. The gas and oil remain motionless into the rocks due to low porosity and permeability of source rocks that is why it's necessary to drill horizontally along the formation and then performing a multi-stage hydraulic fracturing process. The hydraulic fracturing process involves injecting a mixture of water, proppant and some chemicals at high pressure to fracture the rock. The proppant placed into the rock serves to keep fracture open, so that the hydrocarbon can flow to the surface when the wells start their production life span.
Fracturing Fluid
Water is a very important element for most of the hydraulic fracturing treatments; it represents 95% of the fluid components. On the other hand, the proppant is other important material. It is about granular particles, such as natural sand or ceramic, which is mixed with the fracturing fluid, and its mission is to keep the fracture open when fracturing operation is over and the pressure released, keeping the desired conductivity of the fracture during the production life of the well (Trombetta, 2012). There are several fracturing fluid types; the more common are the slickwater, linear gels and cross-linked fluids; though it is possible to find gas assisted and hybrid fluids. Different to assisted fluids, the remaining fracturing fluids mainly contain water (approx. 95% in volume), proppant (approx. 4%) and the remaining 1% of the volume is composed of a number of additives (chemicals).
Technology for the flowback treatment
The returned post-frac water (flowback) represents the higher volume of residual fluid for the oil industry. Published figures in 2007 showed that at worldwide level, between 11,1 and 15,9 million of cubic meters of water were generated (Dores, Hussain, Katebah & Adham, 2012). Besides, for the year 2009 some 3,3 trillion m3 of returned water were produced in the United States (Argone National Laboratory, 2009).
There are a number of technologies for the flowback treatment that could be classified in several ways; the most common is linked to the science of carrying the treatment out. There are three important groups for this type of classification. It is important to take into account that some technologies are part of a specific group and others coincide with the overlapping of two or more types of treatment (Ely, J., Horn, A., Cathey, R., Fraim, M. & Jakhete, S., 2011).
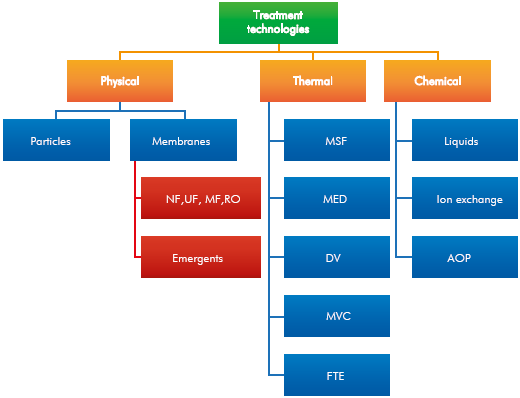
Figure 1 Technologies for the treatment of flowback Notation: NF: Nanofiltration UF: Ultrafiltration, MF: Microfiltration, RO: Reverse Osmosis, MSF: Multi-stage flash distillation, MED: Multi-effect distillation, DV: Dewvaporation, MVC: Steam mechanic compression, FTE: Freeze thaw evaporation. AOP: Advanced oxidation processes.
Physical treatments
These types of treatments are based upon the filtration principle, seeing as the physical remotion of particles by size. Separation might be passive (leaving gravity and/or volumetric flow to do the job), or active (where the energy is used to exert pressure and strength upon the water through the filter or membrane stopping contaminants) (Pierce, Bertrand & Cretiu Vasiliu, 2010). Limitations of this treatment process are mainly linked to the following aspects: membrane manufacturing costs, components linked to the system (spares), continuous contamination of the membrane and the energy requirements to generate the right pressure for the remotion of contaminants. In this way the process is commonly used with feeding currents with concentrations of TDS between 500 and 50 000 ppm.
Among the treatments, the Reverse Osmosis is worth mentioning as the more implemented technology in the oil industry due to its large contaminant remotion capacity (monovalent, divalent and polyvalent ions, amongst others). This can be applied at an approximate rate of 1 000 m3/day. Generally speaking, dense and selective membranes can be used, capable to retain components as small as 0,0001 μm. This process could be efficient for the elimination of sand, lime, slurry, algae, protozoa (5 to 15 um), bacteria (0.4 to 30 um), virus (0.004 to 6 um), humid acids, organic and inorganic chemical products, aqueous salts and metallic and non-metallic ions). The membranes are designed to achieve remotion of the NaCl excess up to 90% (Valero, 2013).
The main cause for failure of the Reverse Osmosis is the contamination of the membrane including deposits of different types of fouling organisms in the surface. The more common contaminants are inorganic contaminants (scales, for example calcium carbonate, sulphates of calcium, siliceous and other species exceeding the saturation index) (Valero,2013), the colloidal contamination (to be typically found via mechanic filtration), biologic contamination and organic contaminants (Zibrida, Amjad, Zuhl & Lewis, 2000).
One alternative for this type of treatments is the Membrane Distillation (MD) whose main feature is the combination of thermal and physical processes to improve the water quality. The underlined benefits with this type of technologies comparing it to the Reverse Osmosis are (Minier-Matar, Hussain, Janson & Adham, 2014): 1) The quality of distilled water, obtained through simple steps different to Reverse Osmosis that requires multiple steps, 2) the quality of the product is not affected by salinity, and 3) low capital cost for its implementation due to very low cost of the construction materials.
Thermal treatments
In this case the energy is used to heat up the flow back that will evaporate later starting its condensation to obtain fresh water. All technologies showed in this section are derived from the energy conservation principle (Ely et al., 2011). The thermal separation processes are still used in places where the energy generation represents relative low costs (Colorado School of Mines, 2009). With the proliferation of Shale gas wells in the United States, the flowback treatment's demand with concentrations higher than 100 000 ppm of TDS has broadened the application of thermal systems for treatment (Dores et al., 2012; Hussain et al., 2014).
The benefits of the thermal treatment are flexibility and solution firmness; flexibility as it works in any type of water (total solids concentration dissolved and suspended (TDS), generating an affluent of good quality equal or higher than 40 000 ppm), and firmness as it eliminates all dissolved and suspended solids also generating a good quality affluent that could be discharged directly into the environment (water bodies). On the other hand, counting the more relevant disadvantages of the thermal treatments, we could mention: flow rates, energy consumption, cost (Ely et al., 2011) and the scales precipitation, very commonly observed in the Multi Stage Flash Distillation and Multi-effect. It is very difficult to achieve high flow velocities in a reduced space and it is very expensive to generate enough energy to obtain water steam and the treated affluent (Ely et al, 2011).
Thermal separation technologies used for desalting, include Multi Stage Flash Distillation (MSF), Multieffect Distillation (MED), Dewvaporation, Steam Compressing Distillation (VCD) and Freezing and Defreezing (Freeze Thaw Evaporation). MSF and VCD are robust and mature technologies used in the water treatment industry with good results for the removal of contaminants.
Chemical treatments
Used processes for residual water treatment in which the transformations have occurred through chemical reactions; these are called individual chemical processes that have been carried out in combination with other individual physical operations and individual biological processes (Metcalf & Eddy Inc., 1995). Liquid chemical treatments have been used historically in the oil industry such as the corrosion inhibitors, scale inhibitors, biocides amongst others. By the other side, the ionic exchange treatment is used for the monovalent, divalent and metals ions remotion of the flowback using resins for that purpose. The ionic exchange technology has a life expectancy of 8 years, requiring a pre-treatment for the elimination of solids. It also requires the use of chemical products for the production of resins and disinfection. Operation costs amount to more than 70% of the total cost of these technologies (Igunnu & Chen, 2012).
On the other hand advanced oxidation processes (AOP), are defined as "those oxidation processes encompassing the generation of hydroxyl radicals in enough quantities to interact with the organic compounds of the environment". The more common AOP processes use ozone combinations (O3), hydrogen peroxide (H2O2), ultraviolet radiation and photo catalysis. One of the consequences of the high reactivity of the oxidant agent, is that the advanced oxidation processes are also known for their low selectivity; but what could be a disadvantage within a production process might be a desirable feature in the case of the elimination of contaminants of residual waters. (Fernández-Alba et al, 2006).
As a summary, Table 1 shows the different contaminants present in the flowback as found in the literature and technologies able to remove them. It is also important to mention that this research was based mainly in technologies used in the development of the more important Shale Plays in the United States.
Table 1 Summary of flowback treatment technologies.
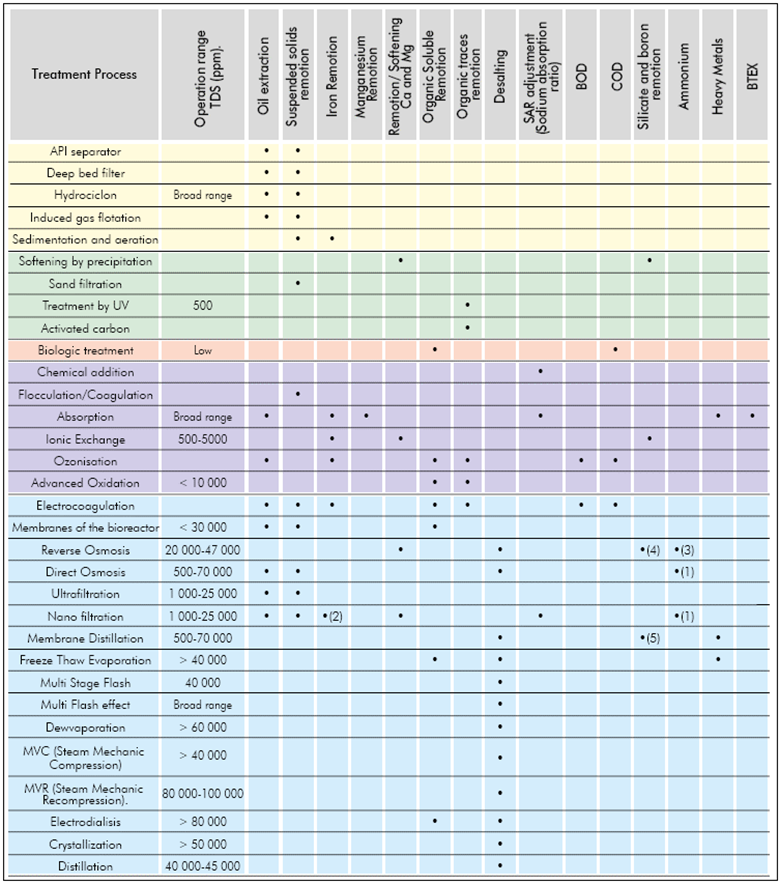
Source: (Colorado School of Mines, 2009; Hussein et al., 2014; Metealf & Eddy Inc., 1995; Igunnu & Chen, 2012; Fernández - Alba, 2006; Shaffer et al., 2013; Guadlip & Paugh, 2008; Pierce et al., 2010; HE et al., 2014; Platt, Burnett, Eboagwu & Vavra, 2011 ; Lee & Frankiewicz, 2005; Webb et al., 2009; Minier-Matar, Hussain, Janson & Adham, 2014; Winter, Koschikowski, & Wieghaus, 2011 ; Jude, 2006; Peeters, & Theodoulou, 2005; Janson et al., 2014; Altela Inc., 2006; Bahar, Hawlader & Woei, 2014; Darwish, 1998; Boysen, 2007; Boysen et al., 1999; Horner, Halldorson & Slutz, 2011; Ortiz, 2013; Arnold & Stewart, 2008; Veil, 2011 ; Ramalho, 1990; Letterman, 1999; Forero, Ortiz & Rios, 2005; Sarria et al., 2005; Ma & Wang, 1998; Arango, 2005; Guohua, 2004; Holt, Barton & Mitchell, 2005)
(1) Remotion 8%, (2) Remotion >99% of MgS04; Moderated remotion of other ores (<90%), (3) Remotion (60%-80%, 4) Less than 50% remotion, (5) Boron Remotion.
The current situation of the oil industry mandates the optimization of the diverse resources. As one of the residues of larger magnitude, the flowback has been re-used to decrease the environment and social impact up to a point, generated by the capture of water within neighbouring communities where hydraulic fracturing activities take place. To achieve this, diverse type of technologies have been implemented and researched to obtain the best possible effect from this residual volume; such is the case of technologies for physical treatment. In spite of its usefulness and evolution within the last years, it is important to identify its major disadvantages such as the previous treatment absorbed by the flowback and the cost of spares; these might be important parameters in the selection of this type of technologies.
Other thermal treatments could be considered as potential options, as these are applicable where other types of technologies could be more expensive to implement. The result of these types of processes is a good one, as these contaminants are almost fully eliminated. Even though, the energy consumption becomes an important practical challenge. On their side, some other technologies for chemical treatment have been also used within the industry such as the corrosion inhibitors. Apart from it some other configurations have come up, such as the AOP under development, and from which promising results have been obtained. It is also important to highlight that there are combinations between the types of treatments, such as the membrane distillation and the membranes bio-reactors as other alternatives for the treatment of returned waters.
3. EXPERIMENTAL DEVELOPMENT
Methodology for the selection of technologies for the flowback treatment.
With the aim to select the more efficient technology (ies) for the treatment of returned waters for the non-conventional reservoirs in Colombia, the following methodology was developed encompassing six steps. Initially the identification of current physical chemical components has been discussed, present in the flowback through the geologic analogy between a Colombian formation and a foreign one, analysis of the fracturing fluids used in that foreign geologic formation and the analysis of the behaviour of the physical chemical components shown in the flowback. Later the flowback volume is calculated with the aim to preselect technologies. Then a study of operational variables is undertaken and the cost implementation, bringing the selection of the right technologies for the treatment of returned waters.
Identification of flowback chemical composition
The proposal in this first step is to identify all chemical components of the flowback coming from the well. Generally speaking this composition is obtained from laboratory analysis. In this research, there is no available a physical-chemical characterization of the flowback coming from a non-conventional reservoir, as the development of this type of reserves in Colombia are still at the starting point. In that sense the following procedure is discussed to be able to identify the different physic chemical components of the returned water in a non-conventional reservoir, including: the characterization of the flowback, through analogies: fracturing fluid analysis used in the Eagle Ford referenced Shale and the analysis of the flow back behaviour which are developed as follows:
Characterization of the flowback using an analogous field in USA
A foreign formation similar to a Colombian formation must be identified in this case, aimed at correlating the physic chemical features of the flowback. According to data as shown in Dong et al. (2014), Lesar (2011), U.S. Energy Information Administration (2011), Murphy (2013) and the characterization of the interval of the bottom of the well La Luna 1, carried out by the Instituto Colombiano del Petróleo (Center of Research, Development, Innovation and Technology-ICP) (2012) where was determined that La Luna formation located in the Magdalena Valley (VMM) and the Shale Eagle Ford, showed similarities in their petro physic and geologic features; as shown in Table 2. In order to establish the similarities between these two formations the following parameters were taken into account: permeability, porosity, TOC (Cerón, Walls & Diaz, 2013), type of hydrocarbons, type of kerogene, temperature and vitrinite reflectance (Ro). Comparing each one of the mentioned parameters between the two formations showed in Table 2, the similarities on data can be ratified.
Table 2 Analogy between the Eagle Ford and the La Luna formations.
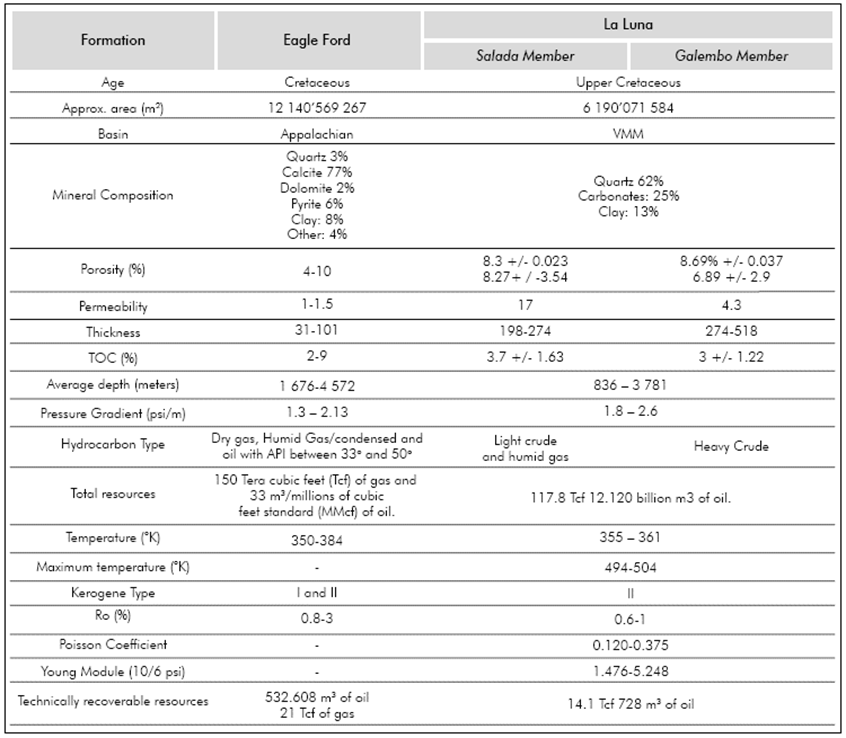
Source: (Dong et al., 2014; Lesar, 2011 ; U.S. Energy Information Administration, 2011 ; Murphy, 2013). Formation data of La Luna shown in the Table, is the result of the comprehensive characterization of the bottom interval laboratory in La Luna well in the ICR 2012
Once the shale Eagle Ford formation has been identified as the foreign formation analogue to the Colombian La Luna, it is assumed that the physic chemical composition of the flowback resulting from this formation in the United States is the base composition of the flowback in a non-conventional reservoir in the Colombian area. In Table 3 the flow back contaminants of the shale Eagle Ford and the water production of the Colombian fields located in the Magdalena Valley are compared, thus for the later works of this investigation, the data from the shale Eagle Ford will be worked upon.
Table 3 Flowback composition in Eagle Ford and production water in Magdalena Valley fields
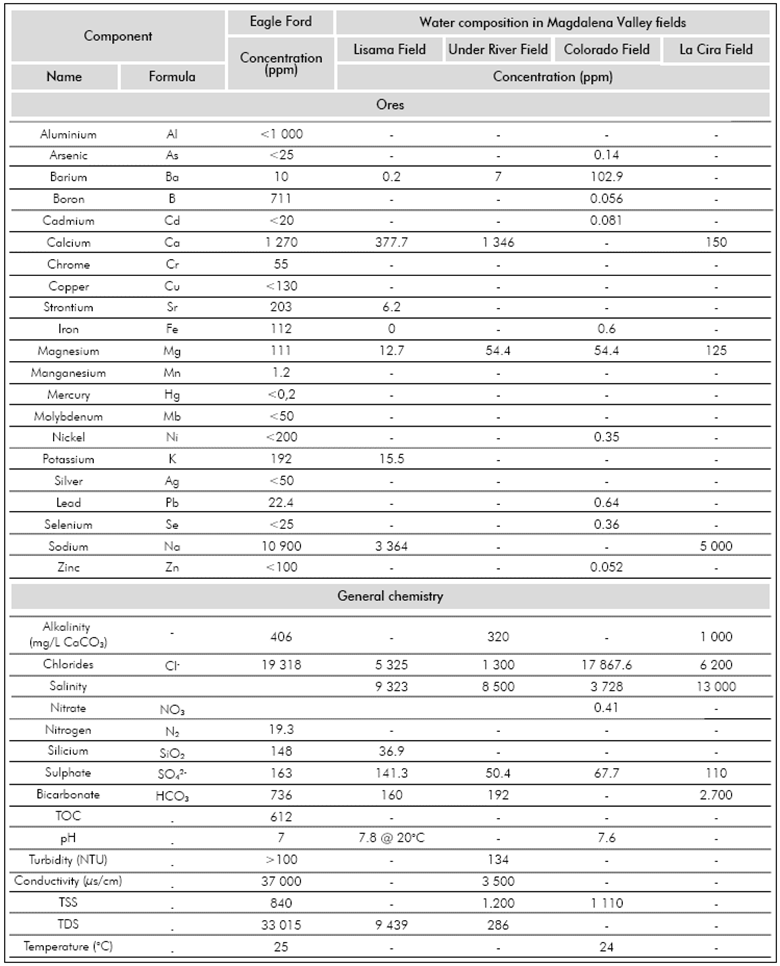
Source: (Slutz, Amderson, Broderick, & Horner, 2012; Halldorson, 2013; Ecolotron, 2014; Naranjo, Muñoz & Zapata, 2010; Benavidez, J. & Jaimes, 2014; Garzón, 2009; Ortiz, 2013)
Analysis of fracturing fluids used in Eagle Ford
As established by the previous analogy, in this section the types of fracturing fluids used in the hydraulic fracturing processes will be identified in the shale Eagle Ford, aimed at establishing the chemical substances affecting the fracturing fluid designed with returned waters so that in this way, the selection of the technologies for the treatment can be undertaken.
The shale Eagle Ford is composed of high ductility formations, subject to anisotropic environment efforts with the tendency to generate bi-wing planar fractures. Under these conditions the characterized stimulate with low fluid volumes and high concentrations of proppant are the right ones to achieve the desired results (Al-Tailji, Smith & Shelley, 2014). Many operators in this shale have obtained the best results with hybrid; crosslinked and slickwater fluids (Al-Tailji et al., 2014; Jaripatke & Pandya, 2013: Cook et al., 2014). Initial wells in Eagle Ford were completed with fluids one hundred percent slick water; the results were not satisfactory however, as they did not generate the enough fracture width a neither they had the capacity for the transportation of high concentrations of proppant. Therefore the change to hybrid fracturing was done using a combination between slickwater and cross-linked fluids. Currently the fracturing fluids design for the completion of the whole Eagle Ford are performed using a pre-pad of slickwater followed by a crosslinked fluid for the pad and proppant transportation steps (Jaripatke & Pandya, 2013).
Across time, the use of certain types of fracturing fluids and some chemical additives for the hybrid fracturing operations in Eagle Ford have changed. Pursuant to data recorded by Robart, Ruegamer and Yang (2013) since January 2011 to June 2011 the proportion of hybrid fracturing fluids pumped at Eagle Ford decreased from 83% down to 63% in all wells. Besides, the use of chemicals in the fracturing fluids showed a decrease. The use of clay stabilizing agents decreased from 62% in the wells in the first quarter of 2011 down to 32% in the wells in the second quarter of 2012. The use of surfactants also showed a similar behaviour, decreasing from 60% to 42% in the wells during the same period.
Amongst the more representative cases of fluid trends used in hydraulic fracturing, two companies are found: EOG and Anadarko. EOG has been active in Eagle Ford in most of the fracturing jobs through the use of hybrid fluids. This company has shown interest in avoiding chemicals specially, such as acids, clay stabilizers and surfactants aimed at optimizing its operations. This analysis cover the hydraulic fracturing jobs in 498 wells since the first of January 2011 to the 30th of September 2012. On their side, Anadarko has diminished the use of hybrid fluids, increasing the use of slickwater fluids in that shale play. This, as the company foresees tangible benefits using these type of fluids and based upon the production records and the reservoirs characterization (Robart et al, 2013). The composition of some fracturing fluids used in some wells by these two companies is shown as follows:
Table 4 Fracturing fluids composition used by EOG and Eagle Ford
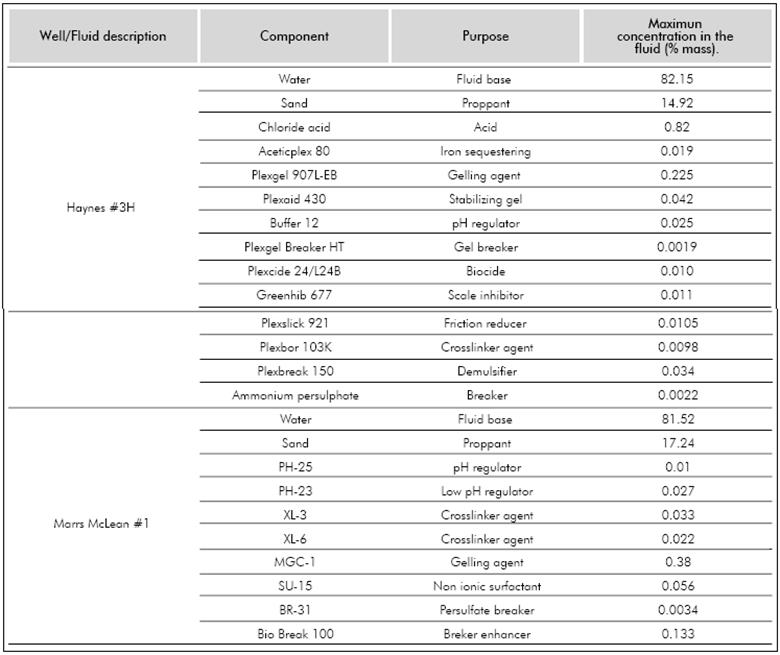
Source: (FracFocus Chemical Disclosure Registry, 2016)
Table 5 Fracturing Fluids composition used by Anadarkoand Eagle Ford
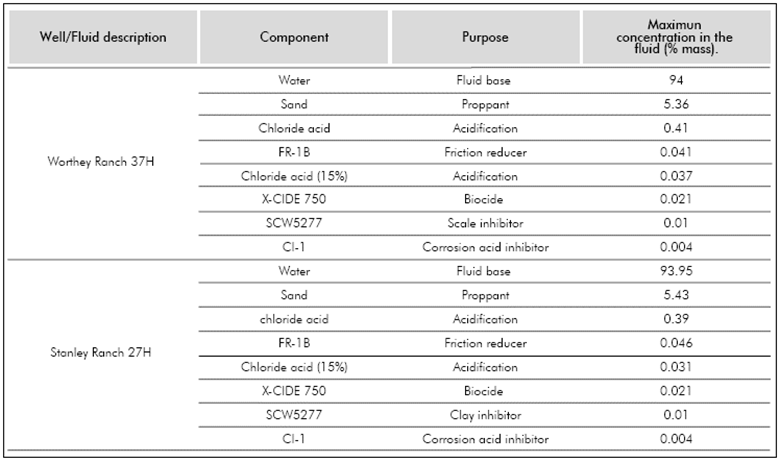
Source: (FracFocus Chemical Disclosure Registry, 2016)
Each one of the additives used for fracturing fluids was the mixed of several chemical substances. It is important to remember that the main objective of this research paper is the reuse of flowback by later stages of hydraulic fracturing, therefore the removal of all possible showed contaminants is not necessary. For these cases, technology should be focused on the elimination of contaminants more affecting the rheological stability of the fracturing fluid, designed with flowback waters. For example, the calcium remotion is necessary due its incidence in two ways on the water quality: 1) it affects the rhéologie performance of the fracturing fluid, but it depends upon the concentration and the fluid's type used. 2) The forming of scale (Calcium carbonate), that can constrain the flow; this should be treated through some treatment technology or through the use of scale inhibitors mitigating its effect. It is also important to consider other elements for the selection of technologies for flowback treatment such as iron, usually removed in some proportion, although this depends upon the fluid used for the fracturing work.
Analysis of the scaling indices of flowback
To analyse the behaviour of the physical chemical components of the flowback, four water stability indices will be analysed allowing identifying whether the volume of water is aggressive, corrosive or scaling. The water is aggressive when this volume has the tendency to dissolve the limestone scales. The water is corrosive when its physic chemical composition favours corrosion in a given metal. Finally, the water is scaling when it has the tendency to form limestone scale. Some methodologies for the calculation of scaling indices are illustrated as follows:
Water stability indices
Langalier Saturation Index (LSI): This index defines the scale potencial of water about to calcium carbonate (CaCO3). It is based upon the effect of the pH on the solubility equilibrium of CaCO3.It allows to determine the stability and the optimums pH of a water. It is also used as an indicator of water corrosiveness. If LSI > 0, the water is oversaturated, the scale can form and CaCO3 precipitation may occur. If LSI = 0, the water is saturated (in equilibrium) with CaCO3, it does not precipitate nor it is dissolved. If LSI < 0, the water is unsaturated, no potential to scale, the water will dissolve CaCO3. The Langelier index calculation is conducted in the following way (De Sousa, Correia & Colmenares, 2010; Varo Galvañ, Chillón & Prats, 2001; Guevara, 2013; Botella, 2014).
Where T is in Kelvin degrees
I= Ionic strength
E= dielectric constant
Ryznar Stability Index (RSI): Empiric index to determine the corrosive or scale tendency of the waters. It is confirmed that the value of this index allows determining the water behaviour. If RSI < 6, indicates that, the water tends to be scale forming. If, 6 < RSI < 7, indicates that the water is considered to be approximately at saturation equilibrium with CaC03. If RSI > 8, indicates that the water is under saturated and therefore, would tend to dissolve any existing solid CaC03. The index calculation of Ryznar is carried out in the following way: (De Sousa et al., 2010; Roberge, 2000):
The term pHs is calculated in the same way as the Langelier index was calculated.
Puckorius Scaling Index (PSI): Similar to the developed Ryznar index. This index is based on the buffering capacity of water and the maximum quantity of precipitate that can form in bringing water to equilibrium. If PSI < 4,5 indicates that scaling may occur. If 4,5 < PSI < 6,5 indicates no corrosion. If PSI > 6,5 indicates tendency to corrosion. This index is calculated in the following way (Guevara, 2013; Roberge, 2000):
The term pHs is calculated in the same way as the Langelier index was calculated.
Larson & Skold index (L&SKI) This index considers chlorides, sulphates and total limestone. It is useful for balanced and purified waters, and especially cold waters. It does not work well with very low limestone waters (desalted waters) or very high limestone waters. If Index < 0,8 there is no corrosion. If 0,8 < Index > 1,2 there is a meaningful corrosion. If Index > 1,2 the corrosion is high. The calculation of this index is carried out in the following way (Guevara, 2013; Roberge, 2000):
Sensitive analysis
Aimed at establishing the physic chemical components affecting the stability indices as previously explained, a sensitivity analysis was carried out. The results can be observed in Figure 3 to Figure 6, noticing three scenarios: (1) Increase of up to 25% of sensitive variable, (2) Base case, and (3) Decreased down to 60% of the sensitive variable. The variable showing the larger dispersion in the increased and decreased scenarios relevant to the base value is the one affecting the stability index.
Table 6 shows a summary of the physic chemical components affecting each one of the water stability indices. These results are later used to preselect technologies for the treatment of flowback.
Apart from the components shown in Table 3, it is important to highlight other component to be considered in the selection of the technologies: such component is TDS, as each technology operates according to a given range of the variable, as shown in Table 1. For this case study it is important to take into account that the returned water provides a TDS concentration of 33 015 ppm (Table 3).
Flowback volume calculation
Pursuant to provided data from Kondash and Vengosh (2015), the average volume of water used for hydraulic fracturing in a non-conventional well in the Eagle Ford is 13 700 m3 for the cases of gas shale formations and 15 060 m3 for the cases of shale oil formations, being an important point of reference for the planning and decision making in the development of the non-conventional resource in Colombia.
It is hope that the projection of the demand of water for the hydraulic fracturing in non-conventional reservoirs in Colombia will be higher as the projects increase as well as the number of wells required to be complete, using this technology. Figure 7 shows the prospection of water demand relevant to the number of wells in which three scenarios are considered (maximum, minimum and average), based upon data reported per well for the shale of Eagle Ford (Osisanya & Haroun, 2015):
With the previous information, the required volume of water is calculated well by well for each one of the scenarios before mentioned, and in function of the number of stimulated wells.
With the total of 18 completed wells through hydraulic fracturing, water consumption will increase to an approximated value of 200 000 m3 for the minimum scenario, 486 000 m3 for the maximum scenario and 343 000 m3 for the average scenario. This shows a tendency toward a growing water demand for the future, which conduce to the necessary reuse of residual volumes generated by the industry these are to be converted into valuable assets for the subsequent stages of hydraulic fracturing.
Once the physical chemical composition of the flowback is known, the calculation of the volume going back to the surface takes place; this is essential information to determine the capacity of the selected technology (ies) for the treatment. This information can be obtained from the reported volume as flowback in stages or previous operations. Because there is not background in Colombia, it will be used data previously shown (Eagle Ford shale in Osisanya & Haroun, 2014) for the water by stage volume and the maximum and minimum number of stages. Therefore, the following results are obtained:
With these data, the calculated average value is 19 079 m3 of water per well. It is assumed that the returning to surface volume is 15% of what was injected. (Halliburton, 2014), therefore the flowback volume is approx. 2 862 m3/well; this value will be used as the capacity of treatment by the selected technologies.
Technologies preselection
A pre-selection of technologies takes place at this stage; these should follow the provided information in the previous methodology stages. In this stage it is possible also to define whether the implementation of one or more technologies is necessary, depending upon the quantity and concentration of existing contaminants in the flowback. Based upon the previously explained specifications (Table 1 information, sensitivity of the indexes of water stability, other contaminants as explained in Table 3, information in Table 7, analysis of the fracturing fluids used in the shale Eagle Ford and the capacity of treatment), the right technologies for treatment are: Reverse Osmosis, Membranes Distillation, Dewvaporation, Vapor Compression Distillation and Flash Multi Stage Distillation.
For this study case, it will not be feasible combine two or more technologies because the selected thermal technologies the flowback don't require pre-treatment or if necessary will require a low consumption of chemical products. Reverse Osmosis represents a different case as this could be combined with other technology to achieve a high quality effluent; however, what is required is the removal of some contaminants for being reused. In this way the effluent obtained through reverse osmosis will allow to design a fracturing fluid with good rheological stability, initially achieved via treatment and also via the addition of new corrosion inhibitors and/or incrustations as shown in Argentina. (Bonapace et al., 2012).
Variable analysis of preselected technologies
In this stage the different variables involved in each technology are assesed: energy consumption, capital cost and treatment efficiency, amongst others. Using a specialized software tool in the topic of treatment design schemes, would improve the aproximation of the real behaviour of the technology(ies), thus the decision would be more reliable. The technical evaluation of each one of the technologies, will allow for the selection to be more focussed on the highest efficiency of the flowback treatment for non conventional reservoirs in Colombia. Four variables were selected for this study. Energy consumption, capital cost, treatmemt capacity and operational cost.
The théorie composition held by the water volumen was also taken into account after the treatment, through the pre-selected technologies. It is worth to clarify that one of the criteria used in the pre-selection was that each technology could achieve a theoretical removal of contaminants in the Flowback between 85 and 90%; this is a range fully dependant on the initial concentration of physico chemical components.
In Figure 8, the higher energy consumptions belong to thermal treatment technologies, were the Multi Stage Flash Distillation is the highest. However, this technology has a high performace (>90%) in contaminants removal. On its side, Membranes Distillation is the technology consuming the lowest, therefore following this variable it would be the more adequate technology for the flowback treatment. On the other hand, Figure 9 shows the capital cost of pre-selected technologies where the higher cost of thermal technologies is identified in respect of the Reverse Osmosis cost; together with the Membranes Distillation, these two latest technologies would be ideal for the treatment.
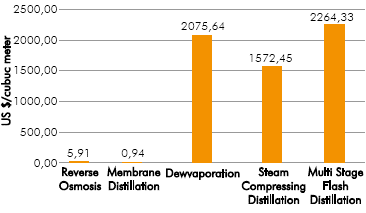
Figure 9 Energy consumption of pre-selected technologies for the Capital cost of the pre-selected technologies for the treatment.
Likewise, it is observed that the Multi Stage Flash Distillation has the highest capacity to treat the Flowback per day (Figure 10). However, the pre-selected technologies such as the Membranes Distillation and Reverse Osmosis accomplish with the specified volume capacity for treatment; thus in an over dimensioned technology, investment would not be feasible from the economic view point. Fort the operation cost's case, Dewvaporation is the technology with the highest operation costs of all technologies (Figure 11); these costs are associated mainly to the maintenance of the treatment system, therefore this would be the first option to be discarded as high efficiency at lower cost is sought after.
In Table 7, the physical chemical composition of the flowback before and after the treatment through each one of the pre-selected technologies is theoretically compared. In detail, all comply with the contaminant removal of interest to make possible to design a new fracturing fluid with a desirable rheological stability.
Cost of pre-selected technologies implementation
In this stage the viability of the implementation of the pre-selected technologies from the economic view point is assessed. It is important to take into account whether the technology is implemented on site or at a distance from the well, as this will imply changes in the final costs. In Table 8, the capital and operational costs in USD/m3 can be observed for each one of the pre-selected technologies. In this way it is possible to conclude that the best option from the economic focus would be Membranes Distillation Technology.
Technology selection
According to provided information in each one of the methodology steps, it is concluded that the best option for the flowback treatment in the non-conventional reservoirs is the Membrane Distillation, as it might have the capacity to remove (~90%) non desirable materials such as calcium, magnesium, sodium, total solids dissolved and chlorides, having a big incidence in the future behaviour and performance of the fracturing fluid. Also it is important to highlight that although it has technologic characteristics for thermal treatment, energy consumption for treated cubic meter is the lowest of all pre-selected technologies (Figure 7) making it as a viable economic and accessible option. Finally and in spite of this technology having a membrane being a possible common and constant cause of failure, the treatment rate per day is high and sufficient enough to take the flowback water for the design of fracturing fluid into optimum conditions.
4. RESULTS ANALYISIS
Taking into account the information collected from each one of the available technologies for the treatment of lowback waters and the analysis of the submitted information in each stage of the proposed methodologies in this article, the right technology to carry out the flowback treatment in the non-conventional reservoirs in Colombia would be Membrane Distillation technology; even having the capacity to eliminate the needed contaminants for the flowback, and the right physical-chemical composition for the design of fracturing fluids, it also has the capacity to treat the required volume. Also, total costs for the implementation of this technology in site, are the lowest (0.94 USD/ m3) in respect of the implementation costs of other technologies. Also other costs associated to operations of this technology are found in second place in respect to what other technologies show. (Figure 10). Through this technology the total projected benefit would be 15% equal to the flowback volume required for later stages of hydraulic fracturing.
It is important to highlight that the result obtained through this posed methodology in this research work is based upon a similar case that should be validated in later studies; including representative samples of lowback coming from non-conventional reservoirs in Colombia, and the implementation of a software tool, allowing to have a better approximation to the real behaviour of the treatment technology (ies).
5. CONCLUSIONS
A methodology allowing selecting the technology (ies) for the treatment of the flowback in non-conventional reservoirs in Colombia was proposed.
It included technical parameters and experienced at real scale in the field of each technology, the analysis of sensibility of four water stability indexes, the correlation of physic chemical characteristics between the formations geologically similar and the cost of implementation.
The methodology submitted by this article constitutes a fundamental tool for the planning and decision making processes within the exploitation framework of non-conventional resources in Colombia. The total benefit projected would be 15%, equal to the flowback required for subsequent stages, in this way decreasing the environmental impact upon the regions where the hydraulic fracturing operations are undertaken.
Based upon the obtained results through the use of the proposed technology, Membrane Distillation would be the adequate technology to carry out the flowback treatment for non-conventional reservoirs in Colombia.
As second option for this type of treatment in Colombia, Reverse Osmosis is proposed taking into account that it has the capacity to treat considerable volumes of flowback and it also has the capacity to remove any type of ions.
Each one of these pre-selected technologies will manage efficiency in the removal of contaminants up to 90%, even though it is important to mention that this value depends upon the initial concentration of physical chemical components showed in the flowback.
The limestone at CaCO3 is the parameter affecting three of the four stability indices analysed in this research work. Under the above, the selected technology was based upon this parameter, as wells as upon the concentration of TDS, chlorides, bicarbonates and other physic chemical components.
Available technologies for treating flowback water and the recent advances in the development of hydraulic fracturing fluids enable a sustainable development to the environment of unconventional reservoirs projects in those countries where the use of fresh water is increasingly restricted or those places with water shortage.
Results obtained through this research work are based upon a similar case, therefore, later studies are required validating the veracity of this methodology. For that purpose, any later stages of this work should include representative samples of flowback waters of non-conventional reservoirs in Colombia, and using a software tool allowing obtaining a best possible approach to the real implementing technologies.