1. Introduction
Enzymatic hydrolysis of granular starch has been used as an effective tool to better understand the starch granule structure. Starch granule is organized in amorphous areas and regions of higher and lower crystallinity, and the transition between them is gradual. The crystalline area is constituted of linear fractions of amylopectin, whereas branch points and amylose are the main components of amorphous areas [1,2].
Differences in the enzymatic susceptibilities of starches have been attributed to the interactions of many factors such as starch source, granule size, and extension of association between starch components, ratio of amylose and amylopectin content, crystallinity, polymorphic type, type of enzyme, and hydrolysis conditions such as concentration, pH and temperature [1,3-5]. Franco [6] reported that the enzymatic attack on the large granules of cassava and corn starches (>16 μm) was characterized by considerable corrosion of granule surface, mainly in the radial direction. Starches with different polymorphic patterns (A, B, or C) have shown different susceptibilities due to enzymatic hydrolysis. According to Hoover y Zhou [1], granular starches (C-type) are more susceptible to pancreatic α-amylase than granular starches with high amylose (B-type), but less susceptible than granular starches (A- type).
The enzymatic hydrolysis of granular starch also causes significant changes in the functional properties of starches such as gelatinization temperatures, gel formation, and paste viscosity, which are very important to define the industrial uses of starches. Hydrolyzed starches obtained with enzymes have their molecular weight reduced and show reduction of paste viscosities and swelling power [7]. Cassava, sweet potato, Peruvian carrot, and potato starches were hydrolyzed by bacterial α-amylase at 37°C for 48 h, and the physicochemical properties of the residues from hydrolysis were determined. Cassava starch was the most susceptible to enzyme attack displaying 20.9% of hydrolysis, whereas potato starch was the most resistant with 5.9 % [5]. These study showed that Cassava and sweet potato starches displayed an A-type X-ray diffraction pattern, while Peruvian carrot and potato starches showed a B-type pattern. These results suggested that hydrolysis occurred in amorphous and crystalline areas of the granules. The B type diffraction pattern in conjunction with the big granule size of the potato starch may have contributed to the greatest resistance of this starch to hydrolysis. The purpose of this study was to assess the dextrin production around of pasting temperature of cassava starch, and determine the morphology, structure and functional properties of the hydrolyzed starch residues, for future industrial applications.
2. Materials and methods
2.1. Source of materials
Starch cassava samples were supplied by the company “Almidones de Sucre S.A.S”, which located in Corozal (Sucre, Colombia). The enzyme α-amylase from Bacillus licheniformis (Novozyme®), with enzymatic activity 129 KNU/g was used in the hydrolysis of starch, enzyme/substrate ratio (E/S=0.81, 1.3, 2.6 KNU/g), temperature (T= 70, 80 and 90 °C) and reaction time (15, 17.5 and 20 min).
2.2. Enzymatic modification of cassava starch
The method applied by Matute [8], was used to determine α-amylase activity. To modify the cassava of starch, 120 mL of starch slurry to concentrations of 20, 40 and 60 g/L of starch was prepared, and 315 μL of the hydrolyzing enzyme was dissolved in it. The starch slurry was maintained in agitation at temperatures of 70, 80 and 90°C, with pH of 5.5 (0.1mol/L sodium citrate buffer). During the reaction, samples were taken at timed intervals according experimental design (15, 17.5 and 20 min) to determine reducing sugars using the dinitrosalicylic acid method, Miller [9] and to calculate dextrose equivalent percentage (DE) and yield (Y), which was defined as the ratio between the amount of starch at the end of enzymatic hydrolysis and the initial amount of starch at the beginning of the hydrolysis process. The reaction was stopped by using hydrochloric acid (2 mol/L) to decrease the pH to 1.5 over the course of 5 min and then using sodium hydroxide (2 mol/L) to increase the pH to 6. Finally, the starch was allowed to settle, the supernatant was removed and dried at 40 °C up to a moisture content nearly 12 % w.b.
2.3. Scanning electron microscope (SEM)
Dehydrated cassava starch samples were placed in a vacuum evaporator and covered with 9 mm-carbon tape and gold-palladium coating. The samples were analyzed in a scanning electron microscope (JEOL, JSU-5600 LV, Japon and SEI, detectors, USA) operating at 15 kV accelerating voltage as reported by Sánchez [10].
2.4. X-ray diffractometry (XRD)
X-ray the diffractograms of starches were recorded with a diffractometer (Panalytical, X'Pert MPD, Switherland) operating at 1.8 kW, 40 mA. The Diffractograms were acquired over 2θ range of 4 to 45°, with a step of 0.013°, a time step of 59 s, divergence grating of 1° wide, a dispersion grating of 1°, a 10 mm mask, and a solar grating of 0.07 rad. The method described by Nara y Komiya [11], was used to determine the material’s crystallinity degree (CD). This method estimates the ratio of absorption peak areas (due to crystalline regions) and total sample area throughout integration [12]. The CD was calculated using Equation 1.
Where, A C corresponds to the crystalline areas of absorption peaks and A A is the diffractograms amorphous area. The CD of the starch samples was quantitatively determined with the program Origin 8.0®, trial version. (Origin Lab Corporation, USA).
2.5. Fourier transform infrared spectra (FTIR)
Infrared spectra of native and modified starches were acquired using a Spectrometer of Fourier Transform Infrared (Thermo Scientific, IS50 FTIR, USA) in the region from 4000 to 500 cm-1, the analysis was conducted with the software Thermo Scientific OMNIC. The crystals were obtained by mixing 20 mg of starch with KBr at a ratio of 1: 5 (starch: KBr). Thirty-two readings were collected at a resolution of 4 cm-1 [13].
2.6. Amylose content
The amylose content of the starch was determined in accordance with the methods of ISO [14], using the standardized iodine colorimetry method. Potato amylose was acquired from Sigma Aldrich (St Louis. MI, USA) and was used as the standard for generating the calibration curve. The absorbance of the starch-iodine mixture was read at 620 nm.
2.7. Cold water solubility (CWS)
Cold-water solubility of the starches was determined following the method described by Schoch [15] with some modifications. Starch sample (0.5 g) was added and solubilized in distilled water (50 ml) with manual stirring. Starch suspension (25 ml) was then transferred in tubes and centrifuged at 920 x g during 10 min. A 20 mL aliquot of the supernatant was transferred to a tared Petri dish and dried in an oven at 110°C during 4 h. The cold water solubility (CWS) expressed in percentage was defined as shown in Equation 2.
2.9. Water holding capacity (WHC)
Water holding capacity is defined as the amount of water retained by the sample without being subjected to any stress, the method described by Sosulky [16] with some changes was used. A sample starch suspension (12 ml) was prepared with distilled water at 10% (w/v) and 30 °C. The sample suspension was mixed with a vortex, 10 g were transferred to a centrifuge tube and centrifuged at 800 x g during 30 min. Then, the hydrated solid was weighed after removing the excess of water. The water holding capacity expressed in percentage was calculated as shown in the equation (Eq. 3).
2.9. Freeze-thaw stability (FTS)
Native and hydrolyzed cassava starches suspension (5% w/w) were heated in water bath at 90 °C for 10 min, cooled to room temperature and storage at -4.0 °C during 24 h, followed by 1.5 h in a water bath at 30 °C. These samples were centrifuged at 2800 × g during 15 min and the amount of water separated after the freeze-thaw cycle was determined. Then the samples were frozen again at -4.0 °C and the same procedure was repeated. Measurements were carried out during three freeze-thaw cycle. The syneresis was calculated as the ratio between the weights of the separated liquid from gel and the gel collected after centrifugation [10].
2.10. Pasting properties
Various pasting parameters of starches such as: maximum viscosity (Vm), pasting temperature (Tp), final viscosity (Vf), final viscosity at 95 °C (Vf-95), breakdown (Bd), and setback (Sb) were determined using a Rheometer (MCR 302, Anton Paar, Austria) and a rapid analyzer starch (Anton Paar, SAA24-2D/2V, Austria). The Approved Methods of the American Association of Cereal Chemists [17] was used to determine the viscosity profiles of the starch dispersions. Starch suspensions (2 g) were prepared to determine the viscosity profiles of the starches using a 23 min standard test, which involved: initial equilibrium at 50 °C for 1 min, heating from 50 °C to 95 °C over 7.5 min; holding at 95 °C for 5 min; cooling from 95 °C to 50 °C over 7.5 min, and holding at 50 °C for 2 min.
2.11. Experimental design
A central composite design was applied to examine the combined effects on the degree of hydrolysis, yield, the functional properties, morphology and structure of cassava during enzymatic hydrolysis of three independent variables: enzyme/substrate (E/S=0.81, 1.3, 2.6 KNU/g) ratio, temperature (T= 70, 80 and 90 °C) and reaction time (15, 17.5 and 20 min). A comprehensive study of the effects of these process parameters and their interactions was carried out with the aid of the statistical software (R). Experimental data to a second order model were adjusted to equation (Eq. 4).
Where β0, βi, βii and βij are regression coefficients for the intercept, linear interaction terms and quadratic, respectively and X is the independent variable.
3. Results and discussion
3.1. Enzymatic modification
Two products were obtained from the production of enzymatically hydrolyzed cassava starches: liquid dextrin with different DE and starch granule residues. Table 1, summarizes the results of Y and DE of the hydrolyzed starches solutions. Equation 5 explains this behavior with a regression coefficient of 92.49% and lack fit of 0.41 for DE, indicating that the model is appropriate for describing the behavior of the phenomenon. Obtaining DE values of between 13.92 and 92%. The temperature had a greater effect over DE. The yield values were obtained between 0 and 45.5%. Furthermore, hydrolyzed starch residues (Y) were obtained when the process temperature for the modification starches was close of the pasting temperature of native cassava starch (69.4°C), and the yield was reduced with the increment of the reaction temperature, but E/S ratio increased yield slightly.
Table 1 Yields and percentage of dextrose equivalent (DE) in the production of enzymatic starches.
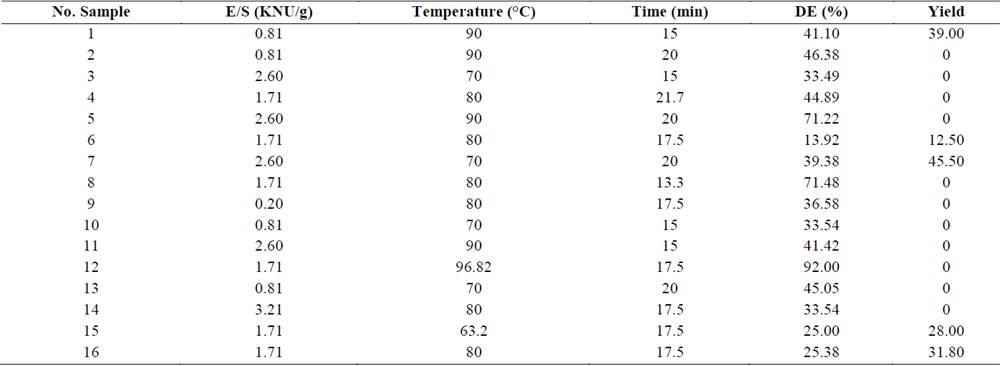
Source: Authors
Analysis of variance showed that temperature had a significant effect on the DE obtained (p<0.05), while neither the other factors nor their interaction had effects. When the enzymatic modification was performed at over 90°C, which corresponds to the maximum activity conditions of the α-amylase enzyme, DE values maximum were obtained. This phenomenon is related to the starch gelatinization process, which begins at around 69.4°C in native cassava starches. This process involves the breaking of the hydrogen bonds and the bond strength that keep the crystalline structure organized, resulting in the loss of the intragranular order, which promotes the absorption of water and the leaching of hydrated molecules to the outside of the granule. Thus the starch thus becomes more digestible as the degree of gelatinization increases and present a most availability to the enzyme action [2,4].
3.2. Scanning electron microscopy (SEM)
Scanning electron microscopy revealed the effects of the enzyme attack on the starch granules. The granules of native starch cassava have spherical shapes with truncated extremes and some oval shapes, according to results reported by Sívoli [18]. After enzymatic hydrolysis, cassava starches showed granules degraded mainly on their external surface and possibly internal surfaces (Fig. 1) according to results reported by Khatoon [19], enzymes cause alteration on the granule surface and degrade the external part by exo-corrosion. When endo-corrosion occurs, the granule is corroded forming small pores through which the hydrolyzing agents penetrate into the granule, indicating that hydrolysis occurred by two type of attack, either tangential or radial (exo-corrosion and endo-corrosion), changing its internal structure. In the surface of native starch granules, no pores or pits were observed. When cassava starches were hydrolyzed, the granule morphology was different to the native starch, granule degradation increases when temperature and time also increase, close of temperature of major enzymatic activity of the α-amylase. The sample No 7 revealed a higher degradation than the other samples, which corresponds to the greatest time and E/S ratio. SEM images are different to the reported by Chen and Zhang [20], where the reaction temperature was below the gelatinization temperature (50°C) with a glucosidase enzyme and not show the destruction of the granules by gelatinization process. After hydrolysis, cassava starch showed some granules degraded on their external part, indicating that hydrolysis occurred by exo-corrosion, and it was not uniform for all granule, enzymes cause alteration on the granule surface and degrade the external part by exo-corrosion. When endo-corrosion occurs, the internal part of the granule is corroded forming small pores through which enzymes penetrate into the granule. In this study, it was not possible to observe any endo-corrosion of the starches after hydrolysis.
3.3. X-ray diffraction (XRD)
The XRD diffractograms of native and starch residues hydrolyzed by α- amylase are shown in Fig. 2, peaks were observed at 15°, 17°, 18° and 23°, these starches presented an A-type diffraction pattern. This type of pattern is characteristic of starches from tubers with low degrees of crystallinity, since the double helixes from the amylopectin in their structures are packaged in a monocyclic shape and consist of 4-8 water molecules, forming a less organized structure. The peak intensity and the crystallinity degree (Table 2) of enzymatic starch residues increased lightly during hydrolysis, the results of XRD for enzymatic starch residues indicated that amorphous structure was hydrolyzed faster than crystalline structure. This observation agrees with Shariffa [2], who suggested that amylolysis primarily occurs in the amorphous region of starch granules.
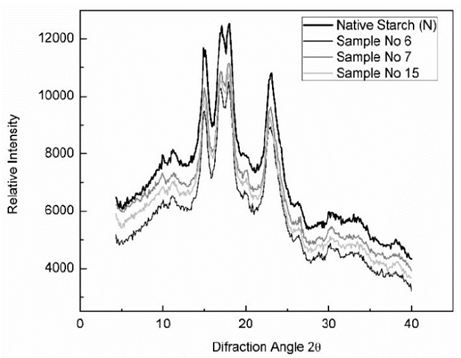
Source: Authors
Figure 2 X- ray diffractograms of native cassava starch (N) and samples of residue of hydrolyzed cassava starches (No 6,7 and 15)
Table 2 Functional and physical properties of untreated cassava starch (Native) and Samples (No 7, 6, 15) residue of hydrolyzed cassava starches.

Source: Authors
Hydrolyzed cassava starch had a reduction in relative crystallinity compared to control starch. It is feasible that, apart from amorphous region, a portion of the amylopectin in crystalline regions was also degraded [21]. Rocha [5] concluded that cassava starch showed increased susceptibility to enzymatic attack because the amylose-amylopectin packaging complex, gives a less rigid and organized structure. Results show that A-Type, are more susceptible to α- amylase than are the B- and C- type starches [22,23].
3.4. Fourier transform infrared spectroscopy (FTIR)
Fig. 3 shows, the FTIR spectra of the native cassava starch and the hydrolyzed cassava starches. The FTIR spectroscopy has been used as a quantitative tool and describes the organization and structure change in starch. The residue of hydrolyzed cassava starches (samples No 6, No 7 and No 15) showed an increase in absorbance than native starch, in the region between 800 and 1500 cm-1 provided highly overlapping and complex spectra making the band assignment more complicated and dominated by the vibrations of glucose molecules [19]. Infrared absorption at 928 - 930 cm-1 was attributed to the glycosidic linkages in starches, the absorption bands at 1020 - 1250 cm-1 are related with CH2OH mode as well as the C-O-H deformation mode, the infrared absorption region 1630- 1640 are related with the tightly water, the FTIR spectra in the region 2800- 3000 cm-1 was due to the C-H stretching mode and the O-H stretching mode of starch was observed in the region of 3000-3600 cm-1 [24]. Starch residues had changes in absorbance compared to the native starch, but do not showed major changes in the organization and structure of starches.
3.5. Amylose content
The amylose content in native cassava starch was of the 19.46%, result similar to the reported by Rocha [5]. After the hydrolysis process, the amylose content decreases slightly concerning native starch (Table 2). These results agree with those reported in cassava starch, corn and sago treated with enzymes α-amylase [21]. Also, higher amylolytic degradation was observed, when was increased the E/S ratio and period of hydrolysis corresponding to treatment No. 7, reflected in the decrease of amylose, an increased degree of crystallinity and morphological alterations in the hydrolyzed starch, resulting in the granule exo-corrosion. Studies report that amylolytic enzymes can hydrolyze both fractions of amylose and amylopectin, simultaneously, although it is unclear what fraction is more susceptible to enzymatic attack [2,5]. However, Uthumporn [21] reported that the enzymes attack and preferably hydrolyze the amorphous region where the linear chains are accommodated, causing a decrease in amylose content in the granule.
3.6. Cold water solubility (CWS), water holding capacity (WHC) and freeze-thaw stability (FTS).
Table 2 shows the CWS and WHC of modified and native cassava starches. The solubility behavior of the starches depends of amylose content, lipids content, granule structure, amylose - lipid complex and the leaching of amylose [25]. The results showed, an increasing of the CWS and WHC of hydrolyzed starches compared with the native cassava starch. Similar results were reported in hydrolyzed starches of corn, cassava, sago and beans [20]. This result can be explained because amylose (amorphous region) in cassava starch hydrolyzed was degraded, resulting in better absorption of water and solubilization of the starch granules. Furthermore, the change in the CWS and WHC of modified starch compared to native starches is closely associated with the decrease of amylose content and increased peak maximum viscosity. This change is related to the morphological and structural alterations suffered by the granule during hydrolysis [2].
FTS in cassava starch was influenced by treatment with amylolytic enzymes. a significant increase (P<0.05) syneresis in the native for each refrigeration cycle starch was observed; which is associated with the regrouping of linear chains of amylose and amylopectin, originated closer interaction between them by hydrogen bonds that release water, presenting a strong syneresis as shown (Table 2) in the third refrigeration cycle [26].
In the starches treated enzymatically, the phenomenon of syneresis decreases significantly with the hydrolysis process, which is most evident in the treatment No 7 and No 15, possibly due to decreased amylose or depolymerization of amylose molecules producing areas more linear, argue that the gelatinization and starch retrogradation are closely related to the amylose content [5], results consistent with the results obtained in treatments No 7 and No 15 in the third refrigeration cycle, where samples showed a decrease of amylose content and lower retrogradation (Sb) compared to native starch. The enzymatic hydrolysis produced changes in the structure of amylose, increasing capacity of remove water. Also, the amylose gels retrograde faster and achieve the retrogradation limit after two days, while the amylopectin crystallizes after five days. The reduction of syneresis in hydrolysed starches is associated with the increasing of the crystallinity
3.7. Pasting properties
Fig. 4, shows the gelatinization profiles of the native and modified cassava starches, and Table 3 shows the pasting properties. A reduction in values of pasting parameters (Vf, Vf-95, Sb) was observed in starch residues hydrolyzed by α-amylase. Values of Tp and Bd of starches residue hydrolyzed varied from 71.4 to 75.5 °C and 3450.1 to 5048.7 cp, which were higher than native starch (69.4 °C and 1744.3 cp) respectively, the increase in the breakdown viscosity (Bd) indicate a greater instability of the paste in the residues starches hydrolyzed and a reduction in the (Sb) shows a decrease in cohesive properties. The residues starches hydrolyzed showed higher values of Vm than native starch, due to the reduction of amorphous region and lixiviation of short chains and amylose, an opposite behavior was reported for hydrolyzed corn starches with hydrochloric acid and hydrolyzed cassava starch with bacterial α-amylase at 37 °C for 48 h [5,25], and hydrolysis of granular starch at sub-temperature gelatinization [21].
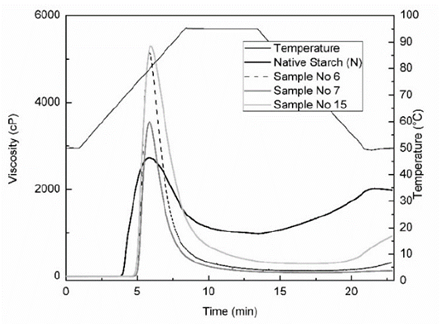
Source: Authors
Figure 4 Viscoamylograms of native cassava starch (N) and samples of residue of hydrolyzed cassavastarches (No 6, 7 and 15)
Table 3 Pasting profile of native cassava starch and Samples (No 7, 6, 15) residue of hydrolyzed cassava starches.

Source: Authors
The pasting temperature (Tp) of residues of hydrolyzed cassava starches increased with the crystallinity degree (CD) and decreased the amylose content due to the reduction the amorphous region of the granule. The native starch showed a greater Sb and amylose values than hydrolyzed starches; therefore, is more susceptible to retrogradation or lose water (syneresis) after 24 hours, that is accompanied by gradual increases in rigidity and a phase separation between solvent and polymer chains when the gel is exposed to the effects of freeze - thaw cycles, causing a decrease in water-holding capacity (WHC), which is fairly common for most native starches aging gels. Also, there is an increase in the cold water solubility (CWS) in the residue of hydrolyzed cassava starches, this is important in the production of liquid beverages.
4. Conclusions
The structural characterization did not show substantial changes in the organization and structure of starches, but the granule morphology was different to the native starch and granule degradation increased with the increment of temperature and time. The native starch presented a greater Sb and amylose values than hydrolyzed starches, thus is most susceptible to retrogradation or lose water (Syneresis), that is accompanied by gradual increases in rigidity and a phase separation between solvent and polymer chains when the gel is exposed to the effects of freeze - thaw cycles, causing a decrease in water-holding capacity (WHC). Also, had an increase in the cold water solubility (CWS) in the residue of hydrolyzed cassava starches. The procedure of how is carried out the hydrolysis of native starches produced different effects on the properties of hydrolyzed starches.
The enzymatic starch can be used as: anti-aging agent of bread, in humectant products, in the preparation of beverages by increasing the solubility in cold water and the decrease of syneresis at a long time (third cycle) and the preparation of yogurts, purées, sauces, creams, cake shop. Avoid using enzymatically modified starches in products where high temperatures are required due to low resistance to these processes