1. Introduction
The addition of gelling agents to culture broths, proposed by Robert Koch in 1880, became a point of inflection in the microbiological field because it allowed the culture of pure isolated colonies [1]. Gelling agents offer a solid and stable structure within the media culture, which absorbs nutrients from the broths to diffuse them to the microorganisms, and acts as a physical barrier that supports the growth of individual colonies, allowing their easy isolation [2,3].
The most widely used gelling agent in the microbiological field is agar-agar, which has been widely distributed since 1905 [4]. Agar allows microorganisms to be incubated at temperatures of up to 37° C because of its high gelation temperature [5]; the high transparency facilitates the differentiation of colonies; the high firmness and low adhesiveness favors the distribution of microorganisms on the media’s surface [1]. Because of these characteristics, agar-agar became an input in high demand in the biotechnology industry, which, during 2009, consumed 10% of its global production [4].
One of the largest problems associated with agar has been the overexploitation of its source of origin - the marine red alga Gelidium spp - which is projected to influence the species conservation with irreparable consequences [6]. For this reason, some of the major producing countries of this algae, such as Morocco, Spain, and Portugal, decreased its harvest, while Japan almost completely abandoned its manufacture [6], causing a 20% decrease in global production [6,7]. Currently, there is no evidence that the product’s shortage is temporary, because there are no methodologies for the artificial cultivation of this algae, and there are few countries that produce it [4,6].
Research into the replacement of bacteriological agar has been carried out with several gelling agents. Babbar & Jain (2006) proposed xanthan gum; Rygaard et al. (2017) proposed gellam gum; Taintor (2003) and Datta et al. (2011) proposed t-carrageenan and κ-carrageenan, respectively; and Das et al. (2015) compiled information in their review article demonstrating the possibility of guar gum and locust bean, among others. In these studies, it was possible to find potential gelling agents with the desired characteristics, such as the formation of stable slants, but with undesirable properties, such as low firmness, high adhesiveness, and brittleness. Carrageenan, despite having highly desirable characteristics, is a product derived from marine algae, so large-scale exploitation could provoke a threat equal to that of agar [6].
A possible solution was proposed by Ozgur et al. (2017), who established a mixture design to quantify the synergies between the components to substitute pectin in acid sucrose systems. As a result, they obtained a formulation that maximized the desired characteristics in the mixture and attenuated the undesired ones, finding a potential substitute of the pectin in the studied conditions. Additionally, to avoid the overexploitation of some of the natural resources, it emerges as a novel solution to the shortage of agar, the implementation of matrices from agroindustry. Research has demonstrated the gelling properties of borojó [13] and whey protein [14], which are abundant resources that have not been studied for the formulation of gels with microbiological application.
As a consequence, the aim of this work was to propose a mixture-design screening to establish partial or complete substitutes of bacteriological agar in terms of firmness and adhesiveness (texture), using several matrices with known gelling or thickener capacity.
2. Materials and methods
2.1. Materials
To test solid media in Petri dishes, the following components were used: agar (Fischer scientific, St. Louis, USA), gellam gum (Spectrum, New Brunswick, USA), k-carrageenan (Sigma Aldrich, St. Louis, USA), ghatti gum (Spectrum, New Brunswick, USA), phase-gel borojó extract (Borojoa patinoi) according to Rodríguez-Bernal et al., (2016), whey protein isolate (WPI; Davisco, Eden Prairie, USA), xanthan gum (Sigma Aldrich, St. Louis, USA), guar gum (Cimpa, Bogotá, Colombia), locust bean (Spectrum, New Brunswick, USA) and arabic gum (Fischer Scientific, Massachusetts, USA).
To prepare basal media M9, the components and the concentrations used per liter were as follows: 6g Nα 2 HPO 4 (Merck, Darmstadt, Germany), 3g KH 2 PO 4 (Panreac, Barcelona, Spain), 1g NH 4 Cl (Merck, Darmstadt, Germany), 0.5g NαCl (Merck, Darmstadt, Germany), 3mg CαCl 2 (Bioquigen, Vancouver, Canada), 1g yeast (Fischer scientific, St. Louis, USA) and 1ml of 1M M g S0 4 (Merck, Darmstadt, Germany).
2.2. Mixture design
To determine the effect of the concentration of the components (factors) on the firmness and adhesiveness of gels (response variables), a screening mixture design was carried out using Design Expert 10 software (Stat-Ease, Minneapolis, USA); the limits of the components in the mixture (levels) are 0.5% for agar, gellam gum and carrageenan, and 3% for borojó extract, arabic gum, ghatti gum, guar gum, xanthan gum, locust bean, and WPI. The obtained results were evaluated by analysis of variance (ANOVA), and then adjusted to different polynomial regression models to select the best fit, determined by the highest correlation coefficient (R2) close to 1.0, and a non-significant lack of fit (p>0.05) [15]. Subsequently, an optimization step was carried out by evaluating the software's desirability function, establishing as optimized variables the maximum firmness, and minimal adhesiveness and agar concentration [12]. The optimal mixture with the desirability close to 1.0 was physically recreated and the texture variables measured. Results obtained for the response variables were compared to those obtained for the reference medium (agar 2%).
2.2. Preparation of solid media
Gels were prepared in distilled water at 90 °C by weighing the amounts of the components on an analytical balance and stirring on a heating plate until a homogeneous solution was reached. Subsequently, they were sterilized in an autoclave (Sturdy, New Taipei, Taiwan) at 121° C for 20 min. The sterilized gels were served in 9 cm Petri dishes, covered with parafilm and allowed to gel at room temperature for 1 h to measure firmness and adhesiveness parameters.
2.3. Firmness and adhesiveness measurement
The firmness and adhesiveness of the mixtures was determined with in a single compression test in a TA XT plus texturometer (Stable Micro System, Surrey, UK) with a 5 kg load cell and a 6 mm cylindrical test piece (p / 6); the pre-test and during test speed was 1 mm/s; the displacement was 5 mm and the trigger force of 1 g [16,17]. Firmness was reported as peak force and adhesiveness as the negative area of the force vs. time graph for each mixture [18]; when the firmness of the gel did not exceed the trigger strength (1 g) was reported as <1 g and its adhesiveness as unmeasurable data (UMD).
2.4. Microbial growth
Soil samples of 100g were collected at a depth of 10 cm from soil at La Sabana University (4°51'25.5"N 74°01'59.3"W). The soil was sieved and homogenized using serial dilution method (10-3-10-6) into Petri dishes using a basal media M9 into two different gelling agents for duplicates (1) agar 2% (2) optimal mixture. Then the results on the counting were analyzed by ANOVA test.
3. Results and discussion
3.1. Mixture design results
Texture results obtained for the different mixtures are shown in Table 1. Treatment 18 in Table 1 had the highest firmness according to the experiment but represents just half the firmness of the reference media (Agar at 2%). The most adhesive treatments were those composed of guar gum. In 10 treatments, it was not possible to measure adhesiveness because its firmness was lower than 1 g. Neither firmness nor adhesiveness recorded from tested mixtures reached similar values obtained for the reference.
Although during the experimental design agar, gellam, and carrageenan weren’t tested as sole components in a mixture, it has been reported in the literature that these gelling agents form thermoreversible gels at low concentrations that interact with other agents through hydrogen bonds [4,5,19].
Treatments with a single component (15, 17, 20, 21, 22, 23, and 24 in Table 1) under the experimental conditions tested cannot form gels, this behavior was previously reported for ghatti [20], arabic, xanthan, guar gums, locust bean [21] and WPI. On the other hand, when they were mixed with another component, such as agar, gellam, or carrageenan, a solid steady structure is developed. For these reasons, the possible synergistical or antagonist effects that the mixture causes during experiments for each component, will be discussed in the following paragraphs
3.1.1. Borojó
Borojó extract was the only component that, mixed with agar (Treatment 5), gellam (Treatment 12), and carrageenan (Treatment 19), formed a gel whose firmness was unmeasurable (firmness >1 g). This behavior is probably the result of the sterilization temperature (121 °C) and the borojó extract pH of 3 [22], which could have hydrolyzed the polysaccharides responsible for gelling in agar, gellam gum, and carrageenan [4,5,19]. Although a buffer solution could be used to avoid the acid hydrolysis of the polysaccharides, the gelling of the low methoxyl pectin of borojó occurs at pH lower than 3 [23]. For these reasons, it could be said that there is an incompatibility in the gelling conditions of agar, carrageenan, and gellam gum, and in future research, this component will be discarded. No previous reports in the literature of borojó mixtures with these gelling agents were found.
3.1.2. Xanthan gum
Treatment 1 (Table 1) demonstrates that xanthan gum’s firmness in a mixture with agar and gellam gum is less than 10 g, which is less than 10% of the control, but its adhesiveness is one of the highest in the study.
Table 1 Texture results for mixture design experiment.
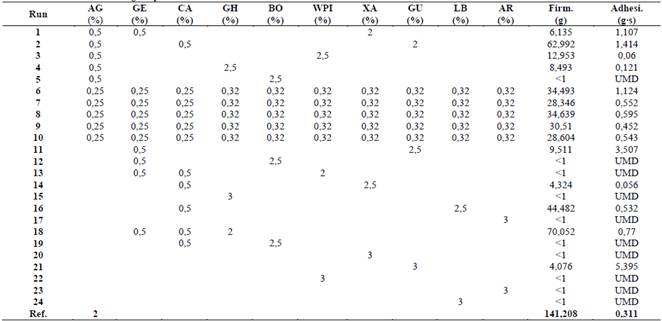
(AG) agar, (GE) gellam gum, (CA) carrageenan, (GH) ghatti gum, (BO) borojó extract, (WPI) whey protein isolate, (XA) xanthan gum, (GU) guar gum, (LB) locust bean, (AR) arabic gum, (Firm.) firmness, y (Adhesi.) adhesiveness, (UMD) unmeasurable data.
Source: author's elaboration.
The literature reports that xanthan gum form weak gels with low firmness and high adhesiveness, both of which increase with its concentration [24]. In this study, was found that whole mixtures with xantham gum were undesirable for their high adhesivenesses and low firmnesses. A possible explanation for this behavior could be its chemical structure which consists of a helicoidal stiff bonding [25]. This structure forms highly ordered networks with weak intermolecular forces that can hinder the creation of new hydrogen bonding with agar and gelan [25,26].
Raina et al. (2011) reported that xanthan mixtures, agar in proportions 0.7%:0.3% and 0.6%:0.4%, formed structures with a firmness between 42 g and 60 g, with an adhesiveness between 32 and 40 g∙s.
In both studies, texture and adhesiveness in the mixtures were lower and higher, respectively, than agar. The main reasons for data differences are because Raina et al. (1) uses basal media rich in salts (MS and B5) instead of distilled water that favors the distribution of the gelling molecules of the xanthan gum [25] and (2) the probe size used was larger than the one used in this study for the texture affecting the result of the texture measurement [27].
3.1.3. Whey protein isolate
During the test with whey protein isolate (WPI), it was observed in Treatments 3 and 14 (Table 1) that the mixture before sterilization forms a yellow homogeneous gel (data not shown), but after sterilization the gel was separated into two phases (polysaccharide-protein or polysaccharide-water), impeding the formation of a stable three-dimensional network. This behavior is because the temperatures reached during sterilization of the treatment exceed denaturation temperature of α-lacto albumin and β-lacto globulin of approximately 80 °C [28], forming a cloudy solution or a clot-like gel [29]. Whey protein has already been used for mixes with gellam gum, which could confirm that problems came not from the mixture but from the process conditions [30].
3.1.4. Guar gum and locust bean
Treatments 2, 11, and 21 (Table 1) with guar gum and 16 with locust bean (Table 1) presented a high viscosity, which hindered their homogenization and dosage in Petri dishes as reported by Das et al. (2015). In Table 1, it was also observed that Treatments 21, 11, and 2 resulted in the highest adhesiveness with values of 5.3, 3.5, and 1.4 g s, respectively.
Treatment 2 also presented the second highest firmness of the experiment (62.9 g), which was 44% of the firmness of control, but its adhesiveness was more than three times higher. Raina et al. (2011) also reported high firmness results (107-261 g) associated with synergies between agar and the galactomannans of guar gum and locust bean, while the high adhesiveness is attributed to interactions between galactomannans molecules. The synergistic mechanisms was explained by Grisel et al. (2015), in their study in which they propose that interactions between these gelling agents increases with the number of unsubstituted hydroxyl molecules of the galactomannans, which are proportional to the galactose/mannose (G/M) ratio. For locust bean, a G/M ratio of 3 implies that it has a greater number of non-substituted hydroxyl groups than guar gum with a G/M ratio of 1.3 [32]. This arrangement of the molecules probably causes, in Treatment 16, the highest firmness in dual component mixtures resulting from hydrogen bonding between locust bean and carrageenan. Higher viscosities in treatments with guar gum are related with the interaction of manose-manose molecules [31]. For future studies, it is recommended to focus on locust bean hydrogen bonding and discarding guar gum for its high adhesiveness.
3.1.5. Ghatti and arabic gums
Treatment composed of ghatti gum and carrageenan (18 in Table 1), shows a firmness of 70.1 g, which represents the highest firmness during experiment, with 50% of control (Table 1). This behavior probably was caused by the formation of hydrogen bonds between the molecules of galactomannans, arabinogalactans, and molecules of agar, carrageenan, or gellam gum [33]. Even when, during experimental design mixtures with arabic gum and agar, gellam and carrageenan were not tested, the same behavior is expected as in ghatti gum, because both have arabinogalactans and galactomannans structure [34]. The application of these two gums is one of the most attractive mixture found in the experiment, because, despite not gelling in concentrations of 3%, they can interact and promote the firmness of the gels to form stable surfaces, without increasing adhesiveness as guar gum does.
3.1.6. Mixture of ten components
Treatments 6-10 represent a mixture of ten components (Table 1). It is observed that these treatments are the only ones with borojó, which forms a gel and allow sterilization of WPI without splitting into two or more phases. This behavior was attributed to smaller proportions of each component decreasing its negative effects on the texture of the whole mixture.
3.2. Modeling and optimization
3.2.1 Modeling fitment
The polynomials with the highest adjustment, which describe the behavior of the response variables according to the composition of the mixture and its ANOVA, are presented in Table 2, Eq. 1 (firmness) and Eq. 2 (adhesiveness):
The ANOVA results of the mixture design are shown in Table 2. The linear model has a significant effect for both response variables and that the adjusted models should describe the behavior of the texture of the gels by their coefficient of determination (Table 2). In Eq. 2, itwas observed that carrageenan is the component that contributes the most to mixture firmness, while borojó and xanthan gum are the ones that most decrease it, and Eq. 2 shows that, as was expected from the literature guar gum is the component that most increases the adhesiveness [32]
Table 2 ANOVA for the adjusted variables to mixture design: firmness and adhesiveness

Source: author's elaboration
3.2.2. Optimization by desirability function
Fig. 1 presents the desirability graph for the optimal result of the mixture design. It is observed that, under optimization conditions (maximum firmness, and minimum adhesiveness and concentration of agar), the mixture with a desirability of 0,976 was composed of 0.5% gellam gum, 0.5% carrageenan, and 2% arabic gum.
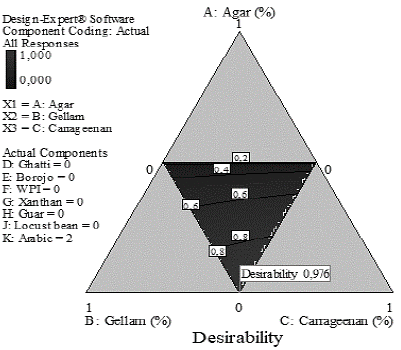
Source: author's elaboration
Figure 1 Desirability graph for optimal mixtures restricted to the maximization of firmness and the minimization adhesiveness and the concentration of the agar.
The optimal mixture presents a firmness 15% higher than agar (Table 3) so the desirability function proposes a mixture capable of substitute agar in firmness.
On other hand, an economical valuation of the mixture demonstrates that this optimal mixture could reduce 25% of cost compared to the reference media (agar 2%), this comparison was based on the cost of the materials used in this study.
3.3. Microbial evaluation
The counts of microbial cells on serial dilutions are shown in Table 4. ANOVA analysis result into not significative differences between both gelling agents (p>0.05). This behavior means that optimal mixture does not affect the viable count of microorganisms, according to the theory that gelling agents are inert into culture media [3].
Table 4 Serial dilution cell counts in soil

Results in the Table 4 are presented by mean of microbial counts (desv.est).
Source: author's elaboration
Further analysis should be carried out to stablish what are the effects of the gelling agents on the microorganisms such as diameter growth, ecological diversity etc.
4. Conclusions
The mixture design proposed in this research allowed the discovery of a substitute formulation to completely replace the bacteriological agar in terms of texture, an elementary property of traditional solid media used for culturing micro-organisms. The mixture, composed of carrageenan, gellam gum, and arabic gum, is 25% cheaper than agar 2% and exceeds its firmness, probably due to synergistic effect associated to hydrogen bonding. Matrices such as borojó and WPI, under the conditions studied, were not viable in terms of texture for later use in the replacement of microbiological agar. This result was achieved by using a screening mixture design, with several components but with a few treatments, which allowed the easy discarding of some matrices and the focusing on those with desirable properties. In conclusion, this research establishes a methodology for future studies to find a solution to the problem of agar scarcity and a new mixture capable of substitute agar in terms of firmness and the growth of the soil sample tested.