1. Introduction
Pervious Concrete (PC) is an especial concrete whose main characteristic is its high permeability, which is related to the porosity of the PC. Since PC is a coarse-aggregate concrete, it facilitates the creation of large interconnected voids that allow the passage of water at high speed [1-5].
Porosity in PC is governed by mix proportion, grain size distribution, typology of the aggregates and the applied compaction process [6-8]. These variables conduct PCs with high variability in hydraulic terms. Generally, the porosity in PC varies from 15 to 30% [9,10] so that the material is considered pervious.
Permeability is the most important property of PC, this is derived from the Darcy´s law for water flow in porous media, and contributes to the PC objective that is the flow of surface runoff through its structure quickly and safely [11-13]. The methodology to measure the permeability can affect the result. According to the literature, two types of tests are applied: Falling or Constant [9-12]. Values of permeability coefficient from 1.5 to 30 mm/s were found [10,14,15].
The correlation for permeability and porosity for PC has been widely studied by some researchers (Fig. 1 and Table 1, Eq. 1, Eq. 2, Eq. 3, Eq. 4, Eq. 5, Eq. 6). The relationship of these two parameters has predominantly an exponential tendency. However, some authors consider that its trend is linear (considering a proportionality between the two properties), as shown in Fig. 1 and Table 1 there are enormous differences with the results found among the correlations.
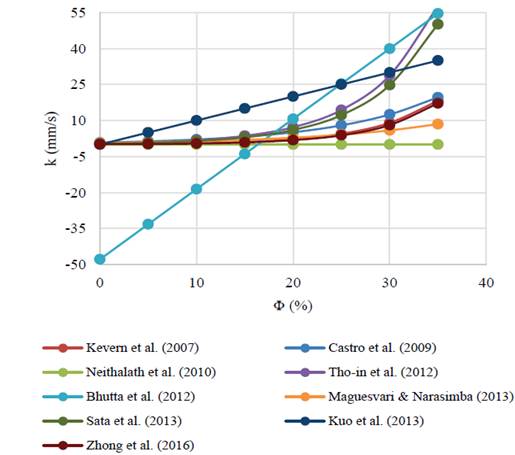
Source: The Authors.
Figure 1 Correlations for permeability and porosity found in literature. Review.
Table 1 References and correlations for permeability and porosity found in literature review.
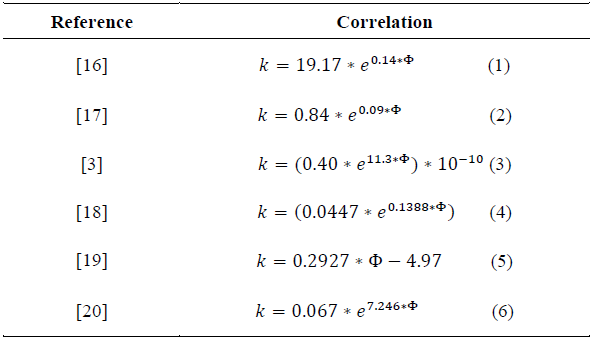
Source: The Authors.
After all, the results found applying these correlations are not entirely realistic, since in most cases for porosities equal to zero, there are permeability values, and in others it gives negative values.
On the other hand, very high permeability values (>40mm/s) were found and for PC it is difficult to reach this limit of permeability. Therefore, the boundary conditions in which these two hydraulic parameters vary have not been established.
Additionally, these proposed correlations do not help in making decisions to run a PC in the field, since they do not establish that there may be a PC that meets the minimum mechanical parameters and in turn it is hydraulically efficient.
PC is generally composed of cement, coarse aggregates (conventional or recycled), water and admixtures (additives and additions). The choice of the grain size distribution of the coarse aggregates depends on the final properties requirement: a continuous grading is recommended if greater strength is required; on the other hand, greater permeability may be obtained using more uniform particle sizes [21-23]. According to the literature [1], the cement ratio (w/c) of PC varies from 0.22 to 0.40.
When conventional aggregates were used to make PC the compressive strength of pervious concrete varied from 3-30 MPa [1,24,25]. Additionally, the use of recycled aggregates has been incorporated in the composition of PC obtaining good results in hydraulic terms [26]. The porosity of the PC manufactured with CCW varied from 23 to 26 %, and the compressive strength ranged from 7 to 12 MPa (which is lower when compared to PC with conventional aggregates) [13,26,27]. These differences are related to the high heterogeneity and the nature of this type of aggregates.
As its mechanical properties are so varied, PC can be used in parking lots, parks, light traffic pavements, pool edges and walkways [28]. For these applications, the runoff reduction and the need to contribute to the recharge of aquifers are the main required properties, and they are directly related to the hydraulic properties instead of the compressive strength [1,25]. These facts made the PC an environmentally friendly material and with the prominent potential to contribute to the solution of the problems related to the runoff.
An experimental program was developed, with the intention to answer these research gaps which initially contemplated the mechanical and hydraulic characterization for PC produced with 4 types of aggregates (electric furnace slag (EFS), ceramic waste (CW), recycled concrete (RCA) and basalt). Then, the verification of compliance with the minimum parameters of the international standards and finally a new model for the hydraulic properties (k-Φ) were proposed and validated with other researcher’s results.
2. Research significance
PC has a very heterogeneous hydraulic behavior, in other words the material can have an equal porosity and two different coefficients of permeability. This is related to the grain size distribution of the aggregates, the amount of cement paste used, the method of compaction executed and the interconnectivity of the voids. These parameters have been studied and there are still great controversies on the contribution of each of them with the interconnectivity of gaps [10].
Therefore, this work studies the possibility to create a correlation of hydraulic properties that allows to identify how efficient the PC will be in terms of permeability based on its porosity, taking into consideration two major laws of hydraulics such as Darcy and Bernoulli's laws. For this, an experimental program divided into four phases was established, as shown in Fig. 2.
As shown in Fig. 2, phase 1 regards the mechanical characterization of the PC mixes and the evaluation of the experimental results; in phase 2 the hydraulic properties of the mixtures are studied; phase 3 compares the experimental data with the requirements established in international standards, and finally, in phase 4 a proposal equation to correlate the hydraulic parameters (porosity and permeability) is presented.
The proposed model will allow to identify how efficient the PC is hydraulically, allowing to find PCs that have a balance of their hydraulic properties, and at the same time comply with the mechanical parameters required by international standards. Therefore, this correlation would be of great help in order to successfully disseminate and apply the material in the project and work.
3. Experimental program
3.1. Materials
The cement used in this study was a CP II-F-32 (Brazilian denomination). This presents a limestone filler addition content of 6-10% that allows a reduction of the clinker in its manufacture and therefore is more environmentally friendly. Tap water was used in the production process to fulfill the standard requirements.
Four different types of aggregates were used in this study (Fig. 3): Basalt, Electric Furnace Slag (EFS), Ceramic Waste (CW) and Recycled Concrete Aggregates (RCA). Notice that basalt is obtained by quarrying process, and thus it is a non-renewable resource, and besides it is the most common aggregate in southern Brazil. That is the main reason why Basalt is used as a reference in the study. On the other hand, EFS, CW, and RCA are denominated sustainable aggregates. EFS comes from the processes for melting scrap metal (recycling) and making new steel products, whereas CW and RCA from the waste generated during construction and demolition of civil infrastructures, the first one of the traditional construction systems, and the second of the construction with structural masonry.
Table 2 presents the density, materials finer than 75 μm, water absorption, bulk density and determination of material finer than 0.075 mm sieve (fines content) for each type of aggregate. These properties were obtained following the requirements of the Brazilian standards NBR NM 53/2003 (ASTM C 127-84) [29], NBR NM 46/2003 (ASTM C 117 - 90) [30], and NBR NM 45/2006 (ASTM C 29 - 90) [31].
The values of these properties are important to correct the water absorption of each aggregate without changing the water-to-cement ratio (w/c) of mix proportion and therefore affecting the mechanical properties of the mixtures [32]. The aggregate that presented higher absorption was CW, what is related to its heterogeneous nature [13,15,20], as expected the density of the sustainable aggregates is lower than the reference due to its production process and nature.
All mixtures were produced with a maximum size of aggregate of 9.5 mm, regardless of the type of aggregate. This was considered to simulate a typical composition of PC [12,21,22]. This type of concrete composed with the selected maximum size presents reasonable mechanical properties without affecting the hydraulic features [23,24]. Fig. 4 presents the particle size distribution of all aggregates used in the study.
The grading curves of the aggregates show a uniform distribution, regardless of the type of aggregates. This is recommended by ACI 522R-10 to produce PC [28]. Moreover, the uniformity coefficients (Cu) [35] of Basalt, EFS, CW, and RCA are 2.20, 1.80, 2.16 and 1.86, respectively. These values are close, so the difference presented among the grading curves is not significant. Apart from that, since Cu values are lower than 5, the grading considered uniform guarantees high hydraulic properties (porosity and permeability) [35].
3.2. Mixture design and production process
To analyze how the type of aggregate affects the final properties of the PC, a unique reference mix was considered. This presented a content of cement and aggregates equal to 420 kg/m3 and 1369.20 kg/m3, respectively. The water-cement ratio (w/c) was also the same for all the mixtures 0.34. The total water content was corrected considering the water absorption results obtained for each type of aggregate [13].
All mixes were produced in a concrete mixer with a capacity of 150 liters. First, the aggregates and 50% of the total water were mixed during 120s. Then, the cement and 25% of the total water were added and mixed during 120s more. Finally, the rest of the water was added and all the materials were mixed for 60s. Notice that this production process was already used in former research studies [12].
Once the PC was mixed, it was used to produce two types of cylindrical samples (100x200mm and 100x150mm, respectively) and slabs (750x250x50mm). Cylindrical samples were placed on a flow table and compacted with 20 blows in two layers, whereas compacting process for the slabs was performed with 5 rubber hammer blows around the mold. In order to reduce the variability introduced by the pouring and compacting process, the same technician was designated to perform the entire procedure for all mixes.
Samples and slabs were placed inside a curing room in the first 24 hours to ensure good curing conditions (temperature and humidity). Then, specimens were demolded and cured submerged in water until the test age.
3.3. Test Methods
3.3.1. Mechanical properties
The mechanical properties of the PC evaluated at an age of 28 days in this study were: compressive strength and flexural tensile strength (Fig. 5). Firstly, to estimate the compressive strength of the PC, cylindrical samples (100x200 mm) were tested following the requirements of the NBR 5739/2007 (ASTM C39/39M-18) [36]. A total of seven samples was tested for each mix. On the other hand, the flexural tensile strength of the mixes was assessed testing four samples (750x250x50mm) regarding the standard NBR 12142/2010 (ASTM C78/C78M-18) [37].
3.3.2. Hydraulic properties
To evaluate the hydraulic properties of the PC mixes two tests were performed: porosity and permeability. Firstly, the test performed to assess the porosity was the same used by Sandoval et al. (2017) in previous research’s [12]. This method consists of filling all concrete pores of a cylindrical sample (100x200mm) with water and correlate the total water volume with the porosity [28, 29].
In that sense, porosity (Φ) was obtained using Eq. 7, that considers the volume of water (Vw) and the total volume of the sample (VT). Notice that five samples for each mixture were tested to obtain this property.
On the other hand, it is important to remark that currently there is no standard to assess the permeability for PC [28]. Concerning that, two types of test are commonly used to measure the permeability coefficient: the falling head permeability test [3,8,10,11,38] and the constant head permeability test [39-44].
In this study, the constant head permeability test was adopted, since according to Sandoval et al (2017) [12] it reduces the variability of the results and the experimental results obtained are sensitive to changes in the aggregate properties. Fig. 6 shows the permeameter used, which uses cylindrical specimen of PC with section and height equals to A and L, respectively, and a known water volume (q). During the test, the time (t) that the water column needs to pass through the specimen is measured.
Then, the permeability coefficient (k) is calculated using Eq. 8. In this study h was 520 mm and equal for all the tests. A total of five cylindrical samples (100x200mm) was tested for each mix.
4. Results and analysis
The results of the experimental program are presented and discussed in this section. Firstly, Fig. 7 presents the mechanical properties results obtained: compressive strength and flexural tensile strength. The results are presented considering the type of aggregate used in the mixture and they show graphically the results deviation.
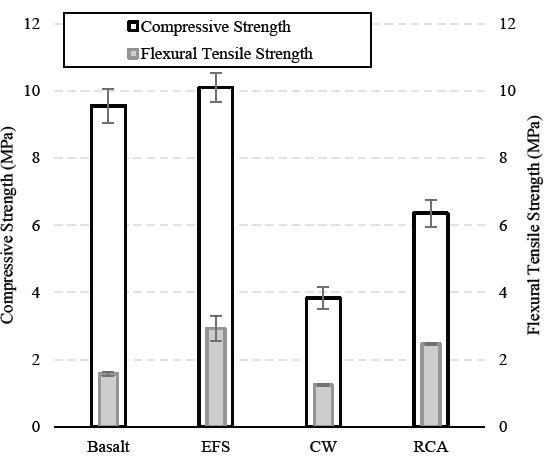
Source: The Authors.
Figure 7 Experimental results for mechanical properties: compressive and flexural strengths.
Regarding the compressive strength results, these varied from 3.3 to 11.0 MPa. This variation agrees with the findings in the literature review [10,28]. Performing a significance analysis with the software ANOVA a p-value lower than 1x10-5 was obtained, indicating that the results presented significant differences considering the type of aggregate used in each mix. The compressive strength of EFS was around 5% higher when compared with Basalt. On the other hand, the compressive strengths of CW and RCA were around 60% and 34% lower, respectively, when compared with Basalt. This suggests that the nature of the aggregates is an important parameter to be considered. The low bulk density of the CW and RCA (Table 1) indicates that they are more porous, heterogeneous when compared to the others aggregates. This difference affects the mechanical properties of PC. Regarding the flexural tensile strength results, these follow the same tendency observed in the compressive strength results as expected [45]. In this study, the flexural tensile strength is on average 40% of the compressive strength agreeing with the results found in the literature review [41].
Fig. 8 presents the results of the hydraulic properties: porosity (Φ) and permeability (k). The results presented consider the type of aggregate used in the mixtures production and the results deviation.
The experimental results show that the porosity varied from 19.1 to 28.6%, whereas the permeability varied from 4.3 to 15.3mm/s. Both parameters presented the same tendency: increases in porosity entailed a higher permeability agreeing with the results found in the literature [14,46].
Besides, in order to analyze how the type of aggregate influences the results, a significance analysis using the ANOVA software was carried out. As a result, a p-value lower than 1x10-5 was obtained, which indicated that there were significant differences in the results of porosity and permeability among the PCs produced with different types of aggregates.
Considering the nature of the aggregates, PC produced with CW, which presented a higher water absorption (Table 1), showed the highest porosity (27.06%). The Basalt aggregate contained more fine grains than the other types, leading to a reduction of final porosity of the material. On the other hand, EFS presented the lowest content of fines, and lowest value of Cu (1.80) entailing an average porosity of 24.75%, which was lower than the one produced with CW.
5. Verification of compliance of international standards and guidelines
In this section, the mechanical and hydraulic properties are analyzed to study the feasibility of using sustainable aggregate in the production of PC. Concerning that, the average values obtained in the presented experimental program are gathered and compared to the requirements presented by the American guidelines ACI 522R-10, the Brazilian standard NBR 16416/2015 and VTT-R-080225-13 guidelines. Therefore, Table 3 shows the average results of the compressive strength, flexural tensile strength, porosity and permeability for each of the studied mixtures. Finally, the mechanical and hydraulic requirements for PC presented in ACI 522R-10, NBR 16416/2015 and VTT-R-080225-13 are presented. The presented requirements are intervals of the research conducted on PC, and in some cases minimum values according to the type of application required, however there are still some gaps regarding the knowledge of the material.
According to the compressive strength results the only concrete mixture with sustainable aggregate that complies with the standards was the EFS, in the case of CW and RCA its values were lower than the required intervals 46.6% and 6.47% respectively.
Table 3 Summary of experimental results and requirements for different standards and guidelines.
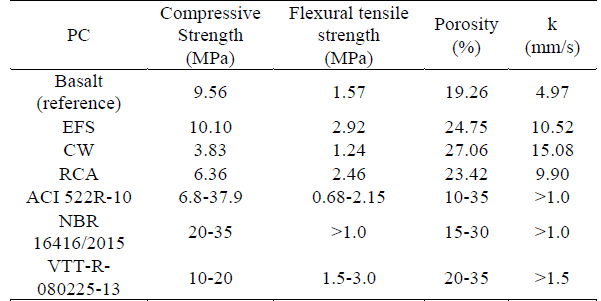
Source: The Authors.
Comparing the flexural tensile strength results with the standards the BFS, CW and RCA meet the ranges proposed in the standards, therefore the EFS can be used in low traffic pavements, parking lots and roadways, and the CW and RCA only in walkways. In hydraulic terms, the concrete mixtures with sustainable aggregates comply with the porosity and permeability intervals of the standards and guidelines.
6. Correlation between porosity and permeability
Darcy’s law and Bernoulli’s law can be applied in PC. Darcy’s law allows he calculation of the flow
, as well as the Bernoulli equation allows the calculation of the velocity, and making the next approach also allows the calculation of the flow
and
The material work as a homogeneous porous medium and its interconnected voids build a network of internal channels, then the PC meets both conditions, and a correlation between hydraulic properties (permeability and porosity) could exist as shown in Eq. 9.
Where:
K = permeability of PC (mm/s)
α = permeability test parameter (mm/s)
Φ = porosity of PC
n = hydraulic efficiency coefficient of PC
i = Hydraulic gradient (permeability test parameter)
g = Acceleration of gravity
h = hydraulic load (permeability test parameter)
Eq. 9 proposes a relationship between infiltration velocity and charge loss with
, which is represented by a parable of degree n using and empirical equation shown in Fig. 9. The
relationship expresses the hydraulic efficiency of the interconnected pores. The
ration increases with the reduction of charge loss, and, as a consequence, the increase of the permeability. This ratio considers the maximum and minimum limits which has these two hydraulic parameters the first, “
” (impervious material) where for a zero porosity in the PC (ideal condition) the permeability will be equal to zero. And the second limit “
” (ATotal=Apores) which has a porosity of one hundred percent the permeability would be maximum. Fig. 9 presents the correlation of k and Φ between these limits.
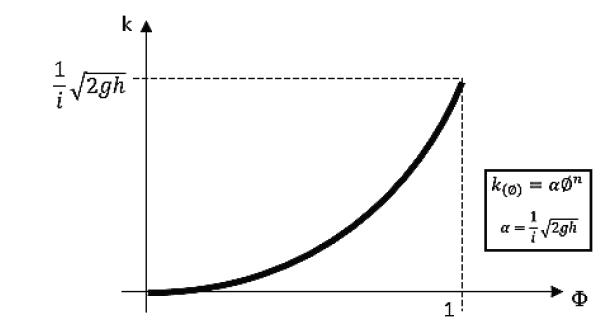
Source: The Authors.
Figure 9 Limits of relation of porosity and permeability (boundary conditions).
Eq. 9 meets the boundary conditions (limits) proposed in Fig. 9
for maximum permeability and
for minimal permeability. The coefficient
of empirical equation showing in Fig. 9 may be determined by non-linear regression, or analytically for each experimental data.
Using the experimental results of porosity and permeability presented in Fig. 8, a non-linear regression using Eq. 9 was performed with the IBM SPSS statistic software Fig. 10 was plotted, showing that the experimental results have a variation coefficient (R2) of 0.98.
As shown in Fig. 10, the curves of the non-linear regression are very close regardless of the type of aggregate used, the values of the coefficient “n” were 3.32 for the basalt, 3.35 for the EFS, 3.29 for the CW, and 2.27 for the RCA. Then there is no significant difference in the “n”-coefficients studied. These two parameters can be correlated, presenting an R2 coefficient equal to 0.97, considering the average results.
In order to validate the proposed correlation, Eq. 9 was used to perform non-linear regressions with experimental data obtained from other researchers [7,23,30,40-42] using IBS SPSS Statistic software. Fig. 11 shows that the proposed correlation presents good fit for all the considered experimental results, being the minimum, the maximum and the average R2 values equal to 0.98 and 0.97 respectively.
As shown in Fig. 11 a different value of the coefficient “n” was obtained from each experimental data from the literature, varying from 2.5 to 5.6. The coefficient “n” is explained as a characteristic of PC directly related to hydraulic efficiency of the internal channels. The voids in the PC are like a series of channels and their efficiency is related to their size and their interconnection, therefore there can be two hypotheses. The first where the PC has a low porosity with a high permeability that is more hydraulically efficient, represented by a coefficient “n” less than 3. And the second where the PC has a high porosity and a permeability less hydraulically efficient, represented by a coefficient “n” greater than 3 as shown in Fig. 11. Therefore, each pervious concrete is characterized by a specific “n” and depending on the needs of a work the PC that best suits them can be chosen.
7. Conclusions
It was possible to propose a correlation of the hydraulic parameters (Φ and k) based on the Darcy’s and Bernoulli’s laws.
The proposed Equation was tested based on the experimental data found in the laboratory and data from other authors showing excellent correlation in all cases, thus demonstrating the predictability of the proposed model.
The proposed correlation adjusted to the theoretical boundary conditions in which both porosity and permeability vary.
The “n” coefficient defined as the hydraulic efficiency of the PC controls the correlation between permeability and porosity.
In practice, lower values of the coefficient n result in PC with higher permeability (more efficient in terms of hydraulic performance).
In mechanical properties, it was demonstrated that for both compressive and flexural tensile strength, the nature of the aggregate used was more important, in this case, the EFS mechanical properties exceed those of the reference (basalt).
The porosities of the PCs with sustainable aggregates were higher when compared with the reference (basalt), since the sustainable aggregates presented lower density, greater water absorption and lower content of fines allowing the formation of high porosities.
According to the verification of the standards and guidelines it is possible to make PC with sustainable aggregates that meets the minimum mechanical and hydraulic properties for the construction in low traffic pavements, parking lots, roadways and walkways.