1 Introduction
Irrigated agriculture accounts for almost 70% of fresh water consumption worldwide [1]. Due to high agricultural demands of available fresh water and the mandatory stance to avoid its deterioration, it is important to discuss the reuse of low quality water in agriculture [2], which may positively or negatively affect the environment.
Further, northeastern Brazil has sedimentary basins with hundreds of oil fields, featuring an annual extraction of approximately 44 million barrels [3] coupled to produced underground water [4].
The chemical composition of this type of produced water is rather complex. In fact, it may contain high concentrations of dispersed oil, soluble salts, heavy metals and other compounds [5].
Due to such characteristics, it should never be discarded in great volumes on the surface and it may not be reused without previous treatment. Otherwise, it may affect negatively soil properties and cause the accumulation of heavy metals and salts at toxic levels and in plants´ vegetal tissues, besides altering the soil´s pH [6].
On the other hand, when properly treated, produced water may be used for irrigation of crops whose products are not addressed for human consumption. They partially supplement the lack of natural water sources and raise nutrient rates in soil and plants and the amount of organic matter in the soil [7,8].
Current paper analyzes the effects of irrigation with produced water treatment, diluted in underground water, on the chemical characteristics of argisol cultivated with forage palms.
2 Materials and methods
Assay was performed between January and September 2018 in a greenhouse at the Department of Agrarian and Forest Sciences (DCAF) on the campus of the Universidade Federal Rural do Semi-Árido (UFERSA), in Mossoró, RN, Brazil, at 5º 12’ 11.25” S and 37º 19’ 25.77” W. According to Köppen´s classification, the region´s climate is BSh [9].
Soil was classified as typical dystrophic red argisol [10], retrieved from the UFERSA´s Experimental Farm Rafael Fernandes, in Alagoinha, in the rural area of Mossoró.
Samples retrieved from depths between 0 and 0.10 m and between 0.10 and 0.20 were collected by driller and spade from six different sites (zigzag mode) in the area. Two compound samples were produced.
The soil´s chemical analyses (Table 1) were performed in the Laboratory of Soil, Water and Plant Analysis (LASAP) of UFERSA, following methodology described by [11].
Table 1. Initial chemical characteristics of soil used in the assay
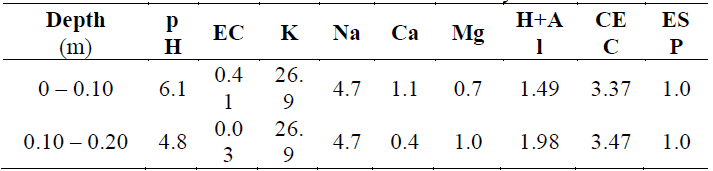
pH - potential of hydrogen; EC - electric conductivity (dS m-1); K - potassium (mg dm-3); Na - sodium (mg dm-3); Ca - calcium (cmolc dm-3); Mg - magnesium (cmolc dm-3); H+Al - potential acidity (cmolc dm-3); CEC - cation exchange capacity (cmolc dm-3); ESP - exchangeable sodium percentage (%).
Source: The authors.
Produced water was retrieved from a petroleum field in the rural district of Jucuri, Mossoró RN Brazil. Water was stored and treated with AGEFLOC DW-3753 on the experimental site. AGEFLOC DW-3753 is an organic polymer, strongly cationic, a polyelectrolyte for flocculation of suspended matter in oil fields and water in general.
Underground water used for the dilution of produced water treatment was retrieved from a tubular well under the administration of the Water and Sewerage Company of Rio Grande do Norte (CAERN).
Treatments followed recommendations by [12] for wastewater dilution in underground water to minimize salinization and sodification risks in the soil. The following treatments were assessed: a) T1 - 100% of underground water (UW), control; b) T2 - 75% UW and % of produced water treatment (PW); c) T3 - 50% UW and 50% PW; d) T4 - 25% UW and 75% PW; e) T5 - 100% PW.
During the experiment, samples from the five treatments were collected every two months, totaling four samples per treatment. Chemical analyses (Table 2) of dilutions were performed according to Standard Methods for the Examination of Water and Wastewater [13].
Table 2. Mean rates and standard deviation of chemical characteristics according to dilutions of produced water treatment.

pH - potential of hydrogen; EC - electric conductivity (dS m-1); Na+ - sodium (mmolc L-1); K+ - potassium (mmolc L-1); Ca2+ - calcium (mmolc L-1); Mg2+ - magnesium (mmolc L-1); SAR - sodium adsorption ratio (mmolc L-1).
Source: The authors.
Twenty-five 20L pots were placed in a row on top of bricks at a height of 0.19 m. Every experimental split, equivalent to a pot, was placed at a spacing of 1.2 m between blocks and 0.50 m between splits of the same block where the forage palm was planted. Each pot was filled with approximately 1.0 kg of pebbles (n.1), covering the bottom of the base, a polypropylene net on the pebbles and 12 kg of soil.
Localized irrigation system was employed, consisting of five 62 L PVC reservoirs for each treatment, five circulation electric pumps Metalcorte/Eberle, self-ventilated, model EBD250076 and 16 mm lateral lines which conducted dilutions of produced water to the pots in which micro-type emitters were installed, with mean discharge of 0.01 m3 h-1.
On January 25, 2018, palms of the species Nopalea cochenillifera (L.) Salm-Dick, cultivar Miúda, were planted in each pot. Palms were half buried in the soil, with their cut side facing the soil, compacted manually for better fixation and growth. The palm´s hydric demand was determined by adjustment coefficients on reference evapotranspiration (RE0), based on Penman-Monteith´s equation [14].
First irrigation with dilution of produced water treatment occurred on February 5, 2018, according to treatments. Irrigation occurred every seven days. There was no fertilization since it would mask data on soil and plant analyses at the end of the assay. Palms received an amount of nutrients in the dilutions of produced water treatment.
At the end of the assay, simple samples of stored soil were collected in 25 experimental splits with an auger, at layers 0 - 0.10 and 0.10 - 0.20 m.
Samples were then stored in labeled sterile plastic bags and sent to the Laboratory of Soil and Water Analyses (LASAP) of UFERSA to determine their chemical properties: potential of hydrogen (pH), with pH-meter; electric conductivity of the soil saturation extract (ECse), with conductivity-meter; potassium (K) and sodium (Na), extracted by Mehlich-1 and flame photometer; calcium (Ca) and magnesium (Mg), extracted by KCl 1 mol L-1; potential acidity (H+Al), extracted by calcium acetate 0.5 mol L-1; cation exchange capacity (CEC), by the sum of base rates (Ca, Mg, Na and K) and potential acidity (H+Al); exchangeable sodium percentage (ESP), by eq. (1), following standards described by [11].
Assay was performed in a randomized block design (RBD), with five replications, in subdivided splits, with splits comprising treatments (T1, T2, T3, T4 and T5) and subsplits comprising soil depth samples (0 - 0.10 m and 0.10 - 0.20 m).
Statistical analyses comprised mean of soil data rates for the two depths. Data underwent variance analysis by F-test at 5% probability and by Tukey´s test at 5% probability, when significant. Orthogonal contrasts were made for multiple comparisons of averages.
Tested contrasts were a) treatment T5 vs other treatments (T1, T2, T3 and T4); b) treatment T4 vs treatments T1, T2 and T3; c) treatment T3 vs treatments T1 and T2; d) treatment T2 vs treatment T1. The combination of contrasts with treatment averages was expressed as follows, according to [12].
C1 = 12 m1 + 12 m2 + 8 m3 +10 m4 - 42 m5
C2 = 30 m1 + 30 m2 + 20 m3 - 80 m4
C3 = 6 m1 + 6 m2 - 12 m3
C4 = 6 m1 - 6 m2
Statistic program Sisvar 5.6 (System for Variance Analysis), developed by [15], was employed for statistical analysis.
3 Results and discussion
Table 3 shows mean rates, coefficient of variance (%), standard errors and probabilities obtained by Tukey´s test, orthogonal contrasts of average of treatments applied according to analyzed variables.
Soil´s pH did not demonstrate any significant effect at 5% probability with regard to tested treatments. However, there was a significant effect for orthogonal contrast C4 at 5% probability, indicating that soil´s pH in treatment T2 differed statistically from T1. According to [16], chemical classification of soil employed in the assay showed weak alkalinity (7.1 - 7.8) and very high pH (> 7.0), by agronomic classification.
Table 3 Chemical properties of soil irrigated with dilutions of produced water treated with underground water.
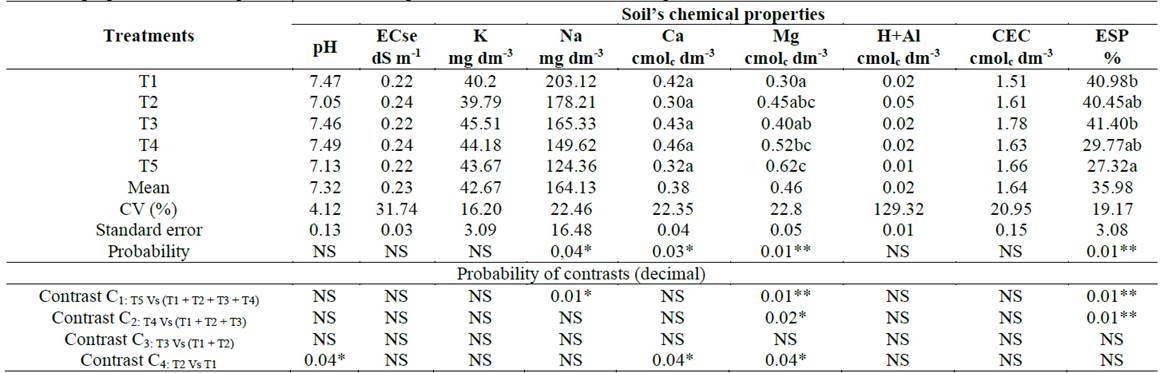
T1 - underground water only (UW), T2 - 75% UW and 25% produced water treatment (PW), T3 - 50% UW and 50% PW, T4 - 25% UW and 75% PW, and T5 - PW only; pH - potential of hydrogen; ECse - electric conductivity of saturation extract of the soil; K - potassium; Na - sodium; Ca - calcium; Mg - magnesium; H + Al - potential acidity; CEC - cation exchange capacity; ESP - exchangeable sodium percentage. C1 = 12 m1 + 12 m2 + 8 m3 +10 m4 - 42 m5; C2 = 30 m1 + 30 m2 + 20 m3 - 80 m4; C3 = 6 m1 + 6 m2 - 12 m3; C4 = 6 m1 - 6 m2.
Means followed by similar letters in the column do not statistically differ by Tukey´s test at 5% probability.
** and *Significant at 1 and 5 % probability by F-test, respectively.
NS - Not significant at 5% probability by F-test.
Source: the authors.
There was a statistical difference at 5% probability for Na and Ca rates and 1% probability for Mg and ESP, when compared to tested treatments.
Lowest Na rate in the soil was reported for treatment T5 (124.36 mg dm-3), due to produced water treatment with low rates of the element when compared to other treatments (Table 2). Albeit under different experimental conditions, [17] reported that increase in the concentration of produced water caused an increase in Na, whereas Mg and Ca decreased.
Orthogonal contrast revealed significant effect at 5% probability when compared to contrast C1 and showed that Na rate in the soil with treatment T5 differed from that in the other treatments.
Na is a cation that enhances the expansion of diffused double layer and, consequently, causes the dispersion of soil colloids, followed by its movement throughout the soil´s profile. When colloids are dispersed and in movement, they obstruct pores and interfere in soil´s physical properties (hydraulic conductivity, infiltration, aeration) and thus in its production capacity [18]. Further, high rates of Na may cause nutritional deficiency of Ca and Mg. The latter are displaced and become unavailable since the roots do not penetrate the sub-surface [19].
Highest rate of Ca was reported for soil with treatment T4 (0.46 cmolc dm-3), as a probable consequence of a higher pH rate of the soil with the treatment. In fact, rise in pH causes complexation or exchange of ions H+ and Al3+ by base cations such as Ca2+, with an increase of saturation per base [20].
Orthogonal contrasts revealed significance at 5% probability for contrast C4 and indicated that Ca rate in the soil with treatment T2 differed from T1.
Highest Mg rate was obtained for soil with treatment T5 (0.62 cmolc dm-3) due to a greater concentration of the element in produced water treatment when compared to underground water. Significant difference at 1% probability for orthogonal contrast C1 showed that the rate of the element in the soil with treatment T5 (treated produced water only) statistically differed from the others. There was a significant effect at 5% probability for contrasts C2 and C4, revealing that Mg rate with treatment T4 differed from T1, T2 and T3. Mg rate of treatment T2 differed from T1.
Soil´s ESP had a similar behavior as Na since it is dependent of the element´s concentration. In fact, there was a decrease in ESP when greater proportions of produced water treatment in the dilutions, with mean rate between treatments at 35.98%. According to some classification criteria of soils affected by salts [21], the soil under analysis is sodic, featuring ESP ≥ 15% and EC ≤ 4 dS m-1. There was a significant effect in orthogonal contrasts at 1% probability for contrasts C1 and C2. The above revealed differences between ESP of treatment T5, when compared with the other treatments, and between ESP of treatment T4, when compared with treatments T1, T2 and T3.
Fig. 1 shows rates of pH, ECse and K of the soil at the end of the experiment, at layers 0.0 - 0.10 and 0.10 - 0.20 m. There was a decrease in pH rates for all treatments, with decrease for T1 and T4 throughout soil´s profile (Fig. 1A). Similar results were obtained by [22] after sunflower crop cycle with diluted produced water treatment, featuring pH decrease throughout the soil´s profile for all tested treatments. Soil´s pH is one of the main factors that affect plants´ growth and development, with best rates at 5.5 - 6.5 range [18]. However, mean pH rates in the two soil layers under analysis for all treatments were above the established limits, probably affecting the availability of nutrients for plants.
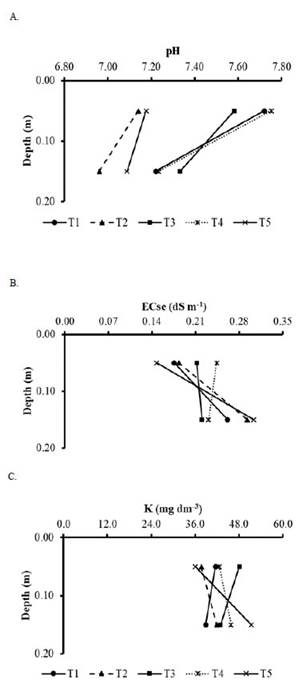
Source: The authors.
Figure 1. Rates of pH (A), ECse (B) and K (C) of soil, according to depths, for different treatments applied.
Fig. 1 B demonstrates an increase in ECse rates from the uppermost layer to the lowest one, in all treatments, with the exception of T4 which showed a mild decrease. However, in all treatments, ECse was low by 2 dS m-1, indicating that there was no salinity in the soil and that effects on crops in general were negligible, according to the Soil Science Society of America [21]. Rise in ECse in the deepest layers may be associated with solubility of salts at the upper layer and posterior leaching to the soil´s lower layers where they accumulate [23]. Results differ from those registered by [22,6], with a decrease of ECse from the surface layer to the deepest one, associated to a greater concentration of salts dissolved at the uppermost layer of the soil.
Fig. 1 C reveals that treatment T5 had the lowest K concentration at the soil´s uppermost layer, with the highest accumulation rate of the element at the deepest. The other treatments did not have significant variations throughout the soil´s profile. Since K is a monovalent ion, it has high mobility in the soil but may easily lose it through leaching, especially in soils with low CEC (such as soil in current study) which received high amounts of Ca and Mg, or rather, elements that compete with K in soil adsorption [18].
Fig. 2 A shows that there was an increase in Na rates according to depth, probably due to the element´s leaching. Result is different from that by [22] where an accumulation of Na was reported in the uppermost layer, associated with the soil´s water evaporation during the application of dilutions of produced water treatment in sunflower crops in argisol.
Ca concentrations in the two layers (Fig. 2 B) revealed depth increase, except for treatment T4 where a decrease occurred. However, treatment showed a higher Ca rate in the soil´s uppermost layer. Under different experimental conditions, [24] demonstrated a decrease in Ca rates from the uppermost to the lowest layers in soil irrigated with filtered produced water treatment by reverse osmosis.
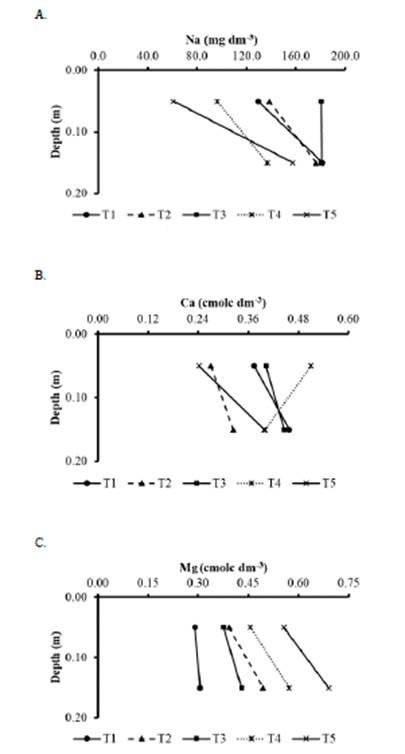
Source: The authors.
Figure 2. Rates of Na (A), Ca (B) and Mg (C) in the soil according to depths for the different treatments applied.
Throughout the whole soil profile, Mg concentrations for all treatments had similar behavior since the accumulation of the element occurred in deep layers (Fig. 2 C). Lowest and highest Mg rates were reported for treatments T1 and T5, respectively, in the two layers analyzed. The above may be due to produced water treatment with higher Mg rate when compared to underground water.
Greater accumulation of Ca and Mg in deeper levels may be due to the sandy texture of the soil under analysis, which reduced the adsorption capacity of cations in the soil and enhanced leaching.
Fig. 3A reveals increase in potential acidity rates (H+Al) in deep layers for treatments T3 and T4, with a decrease for the others. Treatment T5 had the highest rate at the surface. However, H+Al rates are very low in both layers since the soil´s pH is 5.5 times higher. Consequently, the concentrations of exchangeable acidity (Al3+) are practically nil and its presence discarded.
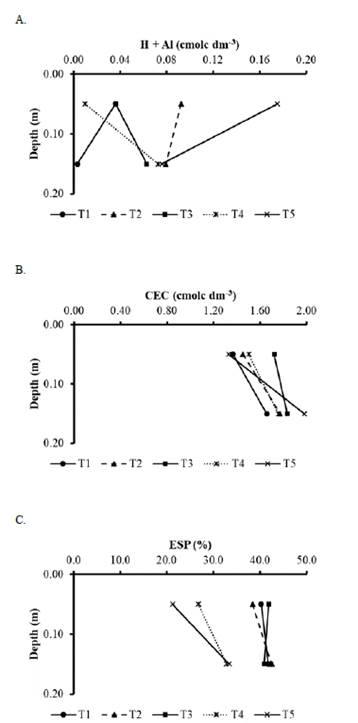
Source: The authors.
Figure 3. H+Al (A), CEC (B) e ESP (C) rates in soil, according to depths, for different treatments.
CEC increased in the lowest soil layer for all treatments, with the greatest variation for T5, throughout the entire soil profile. In fact, T5 had the lowest rate at the surface and the highest in the deepest layers, when compared to treatments (Fig. 3B). CEC behavior was similar to that in Ca and Mg cations throughout the soil´s profile. In fact, variable is partially dependent on these elements and this fact indicates that the soil stored a great amount of exchangeable cations at the deepest layers, or rather, leaching took place.
Since ESP is directly proportional to Na concentration, there was a similar behavior between the two variables. As in Na, treatment T3 had the highest ESP rate at the surface, whereas the lowest occurred in T5 (Fig. 3 C). A similar result was reported by [25] for the irrigation of the castor tree. The authors attributed rise in ESP to greater saline concentration in filtered produced water and, mainly, to the higher exchangeable sodium rate when compared to that in other types of water.
4 Conclusions
In the case of the soil´s chemical properties, sodium, calcium, magnesium and Exchangeable Sodium Percentage (ESP) were significantly affected by applications of diluted produced-treated water. However, there was a significant increase in magnesium rates solely by dilutions.
Maximum culture potential rates may not have been affected due to low capacity of the soil´s cation exchange and to pH rate, which was not the best for the culture´s development.
Irrigation with diluted produced-treated water did not trigger soil salinization since it produced low electric conductivity rates of the soil´s saturation extract. The above indicated the non-occurrence of toxicity specific to ions in soil solution. However, leeching of movable ions, particularly sodium and magnesium, was detected to the soil´s deepest layer.
Highest sodification risks were detected in soil merely irrigated with underground water (T1 - control treatment), when compared with the other treatments.