1. Introduction
Bridges are important structures for economic and social development of communities and become the civil infrastructure of a country to support communication between points including any kind of barriers [1]. There are many design criteria for these structures in order to obtain safety, reliability and durability. However, bridges are prone to develop certain pathological defects due to different aspects: aggressive environment, chemical attacks, more than expected intense traffic, and low quality material, among others, which causes a fast deterioration of their structure. Accordingly, inspection and maintenance activities to preserve and extend useful life of these structures are required [2-4].
Bridge inspection is not a regulated activity in Bolivia. This is the reason why it is not performed in most cases, unlike other countries where specific standards are enforced. In Europe, for example, routine inspections are performed on a yearly basis and detailed inspections are performed as required or according to inspector advice, every five years [5]. In Brazil, an inspection must be performed every year and detailed inspections every five to eight years [6]. In the United States, inspection frequency is every two years [7,8]. Despite this, however, 23% of structures are deficient or operationally obsolete [9]; it is reported that useful life of a bridge in the United States is 42 years, even when they have been designed and built to be used during 50 years [10].
Among main pathological defects found during bridge inspection, iron corrosion, fissures, fungus, and efflorescence, among others [3,11]. Being corrosion the most common and costly issue for intervention and maintenance activities [12].
Usually, bridge inspection is visual and performed by certified inspectors who assess different elements [2]. Even so, this approach involves some inconveniences such as lack of reliability and high variability of results, which are mostly based on each inspector’s experience [13,14]. Therefore, including different tests is required in order to give a more precise diagnosis. Core extraction is a destructive test to determine resistance to concrete compression, traction and flexion in existing structures [15], as well as finding internal defects [16]. Combined with non-destructive tests, results are trustworthy [17]. Despite specific information that could be provided by this essay, it is limited to small volumes of concrete.
Non-destructive testing poses different advantages for inspection processes such as promptness and effectiveness. Available literature presents advantages and limitations, as well as guidelines to be used during inspection and evaluation of structures. These include: Ground Penetrating Radar [2], Impact-Echo [18], electrical resistivity [19], infrared thermography [20,21], carbonation depth by phenolphthalein spraying [3], and chloride penetration [22], among others. Nevertheless, there is a wide variety including different physical principles [14,24].
This article is intended to apply different destructive and non-destructive testing methods to analyze pathological defect appearance in superstructures of five bridges located in Chapare-Bolivia region, since they show evident issues and no inspection or maintenance programs are available in the area. Tests used included sclerometer, carbonation depth and chloride penetration in order to verify results with core extraction.
2. Methodology
Five bridges on the Cochabamba-Santa Cruz Road (RN4), located in Chapare region in Bolivia were selected (Fig. 1). This road is part of the national route and has been used for 51 years since it was built.
2.1 Selected bridges
a) Málaga Bridge: It is located in chainage 85+650 (RN4), canted by 10%, and including a slope of 4.58%.
This bridge does not show deviation but certain developing curvature with 82.56-meter radius and consists of three sections, each 21.60 m long, resulting in a total length of 64.80 m.
b) Ronco Bridge: It is located in chainage 90+950 (RN4), canted by 10% and including a slope of 6.97%.
This bridge shows a deviation of 13º and its developing curvature is 79.58-meter radius. It includes a single section 21.60 m long.
c) Ronquito Bridge: It is located in chainage 91+125 (RN4), canted by 10% and including a slope of 0.30%. This bridge shows a developing curvature including 102.31-meter radius and a single section 21.00 m long.
d) Roquemayu Bridge: It is located in chainage 98+100 (RN4), canted by 10% and including a slope of 1.35%. This bridge does not show any deviation, but its developing curvature includes 119.37-meter radius and a single section 21.00 m long.
e) San Jacinto Bridge: It is located in chainage 99+550 (RN4), canted by 7% and including a slope of 2.30%. This bridge includes three sections 22.70, 23.00 and 22.70 m long, respectively, with a total length of 68.40 m.
2.2 Characterization of the study area
The study area, Chapare Region in Bolivia, is located in a subtropical area, characterized by high relative humidity and constant rainfall, 3801 mm per year. Average annual temperature is 25.2°C [26]. Fig. 2 summarizes historical data for rainfall and temperature in the region during 1982-2012 period.
2.3 Preliminary and detailed inspection
Initially, a preliminary inspection consisting in a visual test identifying the main characteristics for place, weather and relative humidity, visible issues and specific location for every bridge, its access and ability to perform testing was performed.
Subsequently, a more detailed inspection, surveying the total of pathological defect appearance, using a checklist and photographs was performed. Then, non-destructive (silver nitrate spraying, phenolphthalein spraying and sclerometer) testing and destructive (core extraction) testing were implemented for a better characterization of pathological defects found. Procedure adopted for each essay is described below:
a) Colorimetric method by silver nitrate spraying:
UNI 7928 Italian Standard [27] was considered. This standard establishes silver nitrate solution with a concentration of 0.1 mol/l, this is 17 g of AgNO3 per 1 liter of distilled water. During inspection, cracks in small areas of the concrete structure were found, where then a solution of silver nitrate was sprayed. This area remained in a lightened environment (sun exposure) so that photochemical reaction between the solution and the concrete surface took place. Afterwards, a photographic record for the visual analysis of surface coloring was performed. Three points per bridge were determined.
a) Colorimetric method by phenolphthalein spraying:
In order to perform this test, CPC-18 RILEM Standard [28] was considered. This standard establishes a solution of 1g phenolphthalein dissolved in 100 g of 70% ethylic alcohol. After being prepared, phenolphthalein solution was stored in a recipient for further use. During inspection, cracks in small areas of concrete structure were made with a hammer drill and phenolphthalein solution was then sprayed. Subsequently, a photographic record for a visual analysis of surface coloring was performed. This allowed measurement of carbonation depth. Three points per bridge were registered. It is worth mentioning that this test was performed on extracted cores.
b) Sclerometer Testing: In order to perform this test, 7584 NBR Standard [29], which guidelines an appropriate performance, was considered. In order to start the test, assessed areas where dried and polished with a prism to obtain a smooth finish on the surface. A PROCEQ Original Schmidt N hammer was used. A total of 8 tests per bridge were registered.
c) Core extraction: C42/C42M-04 Standard [15] was considered. This standard provided guidelines to obtain, prepare and test cores extracted from concrete in order to determine compression resistance, a value that is to be analyzed in this investigation. Cores were 10 cm in diameter (D) and 20 cm long (L) with corrections whenever L/D ratio was less than 1.75. Two cores per bridge were extracted in points where sclerometer testing had been previously performed.
Fig. 3 shows in detail the tests performed in all five bridges studied.
3. Analysis and discussion of results
3.1 Pathological appearances
During visual inspection, fissures and concrete disaggregation exposing armatures were found in every case. Latter issue is due to steel corrosion process, which gradually evidenced reinforcement exposure with oxidation and loss of cross section (Fig. 4). In addition, it was also evidenced that in some cases it was produced by a motor vehicle accident on the bridge (Fig. 5).
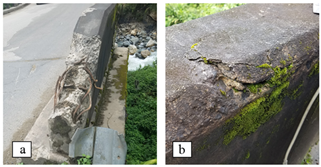
Source: The authors.
Figure 4 Disaggregation of concrete and armatures exposed: a) San Jacinto and b) Ronco bridges.
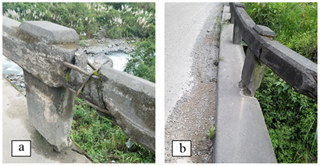
Source: The authors.
Figure 5 Structures impacted by accidents: a) Roquemayu and b) Ronquito bridges.
Another pathological defect found was excessive vegetation, fungus and moss due to high relative humidity in the air and constant rainfall in the region. These issues are shown in Fig. 6.
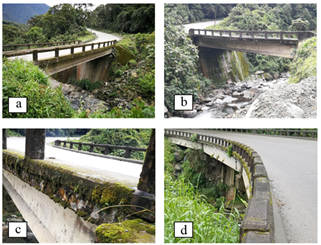
Source: The authors.
Figure 6 Vegetation, fungus and moss: a) Ronco, b) Ronquito, c) Roquemayu, and d) San Jacinto bridges.
It was observed that 100% of the bridges show every issue considered, except for Málaga Bridge, which does not present vegetation (plants) in its superstructure.
Additionally, during inspection it could be verified that some bridges present their drainage systems obstructed by garbage or vegetation, thus evidencing lack of cleaning service (Fig. 7).
3.2 Carbonation depth
Even though corrosion was evidenced in very bridge through concrete disaggregation, additionally, three carbonation depth tests (C1-C2-C3) with colorimetric method by phenolphthalein were performed on every bridge. Table 1 presents results of testing performed.
When concrete was broken in some points, iron corrosion was observed. This was verified by carbonation depth testing, since concrete coating showed no coloring compared to concrete found in inner layers (Fig. 8). It is important to mention that every bridge showed advanced carbonation, which reveals decrease in concrete alkalinity, hydrogen potential (pH) values below eight, which generates iron depassivation [30], that produces corrosion in bars evaluated, although points where carbonation depth was zero, showed corrosion is not present in the whole bridge, but only in some areas.
This corrosion process is due to carbon dioxide (CO2) present in the environment and high relative humidity in this region, as dry pores show no CO2 in progress in concrete due to lack of water. Otherwise, whenever the pores are full of water, carbonation process has ideal conditions to progress until reaching the iron [12]. Additionally, temperature is high in the area, which favors progressive corrosion [31].
3.3 Chloride penetration
When applying a colorimetric method by silver nitrate spraying no results were found in any of three points tested per bridge (P1-P2-P3), which was expected, because chloride penetration is mainly present in marine environments [32,33], and this method does not generate any results when pH is below ten [34]. As shown in previous section, corrosion process by carbonation is present in every bridge.
3.4 Surface hardness rate
Table 2 shows results for surface hardness rate per bridge. Málaga Bridge has the highest average, 48.6, and the least dispersion data, standard deviation of 2.1. Ronquito Bridge showed a lower average, 38, and a significant dispersion, standard deviation of 3.2. Nevertheless, the greatest dispersion regarding average results involved Roquemayu Bridge with a value of 5.3.
Fig. 9 shows a box-and-whisker plot of results for surface hardness per bridge. It is evident that every bridge, except for Ronco Bridge, present a negative asymmetry, the average is below the median, which shows data concentrate on the upper part of distribution. In the case of Ronco Bridge, data concentrate in the lower part.
Málaga Bridge shows that 50% data ranges between 46.5 and 50.3 of surface hardness rate, an interquartile range of 3.8 showing that ranges are close, and value dispersion is low when compared against graphics for other bridges. In contrast, Roquemayu presents the highest interquartile range, 3.8, thus showing a wide value dispersion for surface hardness rate.
It is important to consider that sclerometer test results are influenced by concrete age and carbonation [35,36]. As previously mentioned, studied bridges are old and present carbonation in some areas, thus generating variability in results. However, rate values for surface hardness are used to compare dispersion among these bridges. It was noticed that concrete in Málaga Bridge is better qualified than concrete found in Ronquito Bridge and that concrete in Roquemayu Bridge is the most heterogeneous.
3.5 Core extraction
Table 3 shows results for core extraction, compression resistance and carbonation depth. Additionally, resistance values were added to estimated compression according to surface hardness rate for points E1 and E8. Even though resistance estimation through models reported in literature, -including the manufacturers-, is not completely reliable [23], in this case, they will only be used to be compared against values obtained. It is worth mentioning that estimated values for compression resistance were corrected for carbonation depth, following manufacturer’s recommendations [36].
Table 3 Results for core extraction.
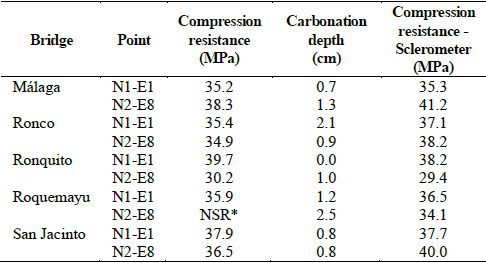
*NCT - No compression test was performed due to iron present in the core
Source: The authors.
Compression resistance is high in cores, greater than 30 MPa. These values are appropriate and recommended for this type of structure [37], but carbonation presence is evident in almost every extracted core.
Fig. 10 shows both core extraction and compression testing. In the case of N2-E8 for Roquemayu Bridge, compression test was performed according to C42/C42M-04 recommendations [15]. This core presented iron and oxidation products (Fig. 11).
A correlation between compression resistance in cores and sclerometer testing was made as shown in Fig. 12. A high positive linear relation could be observed (R 2 =0.695). Nevertheless, significance of correlation coefficient must be determined.
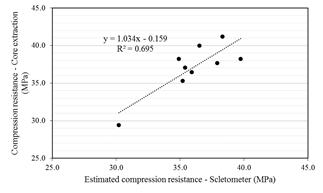
Source: The authors.
Figure 12 Correlation between compression resistance in cores and sclerometer testing.
Significance refers to the probability that this correlation coefficient (R) comes from a population whose coefficient has value zero. This is why two hypothesis are posed: 1) H0:R=0, calculated correlation coefficient comes from a population whose correlation coefficient is zero, and 2) H1:R=0, calculated correlation coefficient comes from a population whose correlation coefficient is other than zero.
A t-student test with eq. (1) is applied.
Where: N, data number, 9 in this case, considering that point N2-E8 was not included in the correlation. Value calculated for t is 3.99.
Value tα,N-2=2.36 is obtained in t-student distribution tables for α=0.05. Then: t > tα,N-2, therefore null hypothesis (H0) with an error probability of 0.05 is rejected. Obtained correlation does not come from a population characterized for having zero correlation. There is a relation between compression resistance both in core and sclerometer testing.
Even though a relation between compression resistance in cores and sclerometer testing is present due to results obtained, determination coefficient (R 2 ) is lower when compared against results found in literature [23]. This is mainly due to data amount. For current investigation, cores were extracted in 10 points only, from which only 9 could be correlated. A greater number of data will enable making different correlations and proposing different models for better adjustment.
Concrete in bridges is acceptable in terms of compression resistance, but corrosive processes that produce disaggregation and armature exposition compromise it. For this issue, different techniques for structural recovery could be implemented, but corrosion process is advanced, and risk of collapse is present. Intervention or structures replacement should be implemented once they serve their useful life [30].
This study has been limited to four tests and an inspection to bridge superstructures, due to difficult access to other areas, and rushing rivers in this region. For a better characterization of defaults and a global study, other non-destructive tests cited in literature may be implemented [14,39], and even an Unmanned Aerial Vehicle (UAV) [40] could be used in difficult access areas such as bridge infrastructure.
4. Final considerations
Bridges in this study show no maintenance service, and they are highly deteriorated. Humidity is a common factor that causes appearance of different issues such as fungus, vegetation and moss, as they also favor corrosive process.
Many corroded portions were detected in the bridges, mainly developed by carbonation. This process could not enable chloride detection.
Non-destructive tests applied generate qualitative and disperse values, but allow comparison and characterization of pathological issues, thus complementing preliminary (visual) inspection. This way, a more accurate diagnosis about structure status is possible.
A model that relates compression resistance in both core and sclerometer testing was found. Although limited, it generates a starting point for further studies in these structures.
Results must be considered by institutions in charge of providing regular inspection and maintenance programs. This way, a proper performance of these structures, mainly in areas where environmental conditions favor a rapid concrete deterioration, may be guaranteed.