1. Introduction
The semiarid region in Brazil covers an area of approximately 982,563 km². The area is demarcated along the 800 mm annual isoethite, with an Thorntwaite Aridity Index (1941) below 0.50, and drought risk above 60% [13].
Rainfall distribution is unpredictable, with long periods of drought, while the annual average temperature is between 23 and 28 °C and the relative humidity is approximately 50%. Annual average precipitation impacts directly on vegetation and soil formation, as well as on society, and climate is thus a major driver of physical, social and ecological processes in this region [24].
The highest probability of rain is from February to May. Rainfall is usually of high intensity, which contributes to soil erosion. Drought is experienced from June through to January [20]. In addition to the direct impact of rain on soil, the pedological characteristics of shallow, rocky soils, and not infrequently sandy A horizons, contribute to soil erosion [16].
A lack of knowledge and good management practices have contributed to soil degradation in semiarid regions [14]. Certain agricultural practices increase vulnerability of the soil to erosion as a result of rain, causing surfaces to become impermeable. Rain falling on bare soil results in the breakdown of soil particles. Splashing of the dispersed particles causes pore obstruction and the formation of a surface seal or a superficial crust [5,17].
The superficial crust influences several soil properties, such as the rate of water infiltration, the hydraulic conductivity of the soil, and runoff, which favors erosion [21]. There has been little research about waterproofing which results from a decrease in the volume of water stored by the soil, despite that fact that it compromises germination and reduces the gas exchange between the soil and the atmosphere.
Waterproofing needs to be investigated further, particularly in semiarid regions where vegetation coverage is scarce and waterproofing and erosion processes occur quickly and severely after precipitation events. Arboreal, shrub, and herbaceous vegetation dominate the Caatinga. This vegetation type is characterized by perennial leaves, with leaf fall occurring at certain times of the year [9], leaving the soil even more exposed at such times to the effects of rain. Dead vegetation (mulch) can be used as a management technique to reduce the effects of rain on soil.
Despite the negative consequences, few studies regarding the erosion process have been undertaken [7], highlighting the need for further research regarding the formation of the surface seal and the monitoring thereof, which is fundamental to soil preservation.
It is thus important to study the soils in the semiarid region of Brazil, especially the cause and effects of soil erosion from water and understanding how to combat surface waterproofing using mulch. The objective of this research is to determine how to achieve balance and sustainability through conservation planning practices, and good land use and management practices.
2. Material and methods
The study was carried out in the Alto Ipanema hydrographic basin, in the municipalities of Arcoverde and Pesqueira, Pernambuco, Brazil, as shown in Fig. 1 (created using ArcGIS® 10.2.2).
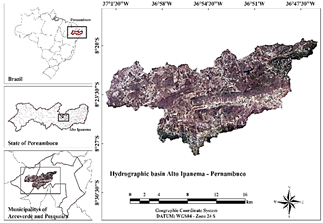
Source: The Authors.
Figure 1 Spatial location of the study area, in the hydrographic basin of Alto Ipanema, Brazil.
The study area is located at Latitude 08º21'28" (S) and Longitude 36º41'45" (W), at an altitude of 654 m. According to [8], the experiment was undertaken in Ultisols. According to Köppen, the local climate is classified as BShw' (warm semiarid), with an annual average temperature of 23 °C. The region also has an annual average rainfall of 607 mm, with a potential evapotranspiration of 2000 mm per year. The physical and chemical characteristics of soil samples taken at depths of between 0-0.20 and 0.20-0.40 m in the study area, are shown in Table 1.
Table 1 Physical and chemical characteristics of the Ultisols in the study area.
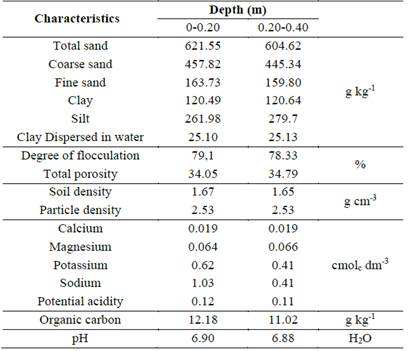
Source: The Authors.
The sampling was undertaken in a randomized design, in a 3 × 2 factorial scheme. The soil was studied under three successive applications of simulated rainfall (0, 24, and 48 h), for both soils with mulch (SWM) and soils without mulch (STM). This was repeated four times.
The variables analyzed were not normally distributed as confirmed using the Kolmogorov-Smirnov, Shapiro-Wilk, and Bartlett tests. Thus, to reduce the coefficient of variation, they were transformed into √x. Through the analysis of variance test (ANOVA), a series of variables were evaluated, such as hydraulic flow characteristics, water and soil losses, aggregate stability, and resistance to penetration. Using the Sisvar software [10], statistical analyses were performed, comparing the treatment averages using the Tukey test at the 5% significance level.
The sample erosion plots measured 3 m x 1 m wide, with the largest dimension in the direction of the slope of the soil, which was 7%. The plots were 0.25 m high and built with metal plates, with approximately 0.10 m driven into the ground. A gutter was located at the base of the plate to collect surface runoff. Two treatments were adopted on the plots: bare soil and soil covered with air-dried Brachiaria decumbens grass, at a rate of 3 Mg ha-1.
A simulator was used to generate rainfall. A Veejet 80-100 spray nozzle with an electronic system to perform oscillating movements was used to simulate rain [18]. This was placed 3 m above ground level and supported in a rectangular frame with removable steel tubes forming four feet. The simulator was powered by a submerged pump that captured water from a 1,000-liter capacity box.
Rainfall observations over 29 years in the Alto Ipanema basin [19] informed the data for the simulated rainfall. A rainfall duration of 30 min, with an intensity of 54.63 mm h-1 and an erosivity index (EI30) of 1,202.87 MJ mm ha-1 h-1 were generated.
Rainfall events were simulated over 24-h intervals, at 0, 24, and 48 h from the first rainfall event. The kinetic energy per millimeter of rainfall was equal to 0.271 MJ ha-1 mm-1, calculated using the Wischmeier & Smith equation (1958) [19]. The total kinetic energy was calculated by adding the kinetic energy of each increment, which was equal to 22.02 ha-1.
Eq. (1), proposed by [3], was used to calculate the water loss, determining the flow volume.
where: Wl = water loss (%), Rb = runoff blade (mm) and Trb = total rainfall blade (mm).
Runoff water samples were collected for 10 s in the collecting channel and used to determine disaggregation rates and soil losses. The samples were stored in 1-L volumetric plastic pots, using the method proposed by [3]. In the laboratory, these samples were weighed and left to rest for 24 h so that the soil present in the water could be determined. The supernatant was then suctioned off, and the remaining soil was placed in a forced ventilation drying oven for 72 h at a constant temperature of 65 °C, for later quantification of the dry soil mass.
Following the methodology presented by [3], the soil breakdown rates (Sd) were determined using Eq. (2):
where: Sd = soil disaggregation rate (kg m-2 s-1), Mdr = mass of disaggregated dry soil (kg), Pa = plot area (m2) and Dc = duration of collection (s).
The soil losses were obtained following the method described in [3], using Eq. (3):
where: Sl = soil losses (kg m-2), F = flow (L s-1), Sc = sediment concentration (kg L-1), Ic = interval between collections (s) and Ap = area of the plot (m2).
For the penetration resistance test, soil samples were collected before and after the three simulated rainfall events (0, 24, and 48 h) using PVC volumetric rings with a diameter of 0.05 m. The extraction of soil in the rings was performed using an Uhland sampler from the manufacturer SONDATERRA®. The samples were wrapped in plastic and stored to retain moisture and structure until they were taken to the laboratory.
The samples were subjected to a voltage of approximately 10 kPa to reach the field-capacity point. Once this was done, the samples were weighed and tested in the bench penetrometer, which has a nominal load of 20 kg and an operating speed of 0.01 m every minute. The needle used was 4 mm in diameter, which penetrated the soil (0.02 m), and the results were presented in force (Kgf) × time (min) graphs. MS Office Excel 2007 software was used to transform the values of force (kPa) into pressure (MPa).
Hydraulic conductivity was analyzed using the method proposed by [23], with modifications. Unformed samples were collected before and after the three intervals of simulated rainfall (0, 24, and 48 h) in both types of treatments, in SWM and STM, totalling 48 samples.
The polished soil slide method was used for surface sealing analysis. Sections of PVC tubes with a diameter of 0.075 m and a height of 0.05 m were used as soil samplers.
A sample was collected as a control before the rainfall simulations were commenced. After the rainfall events of 0 and 48 h, samples were collected from the plots with the two treatments, namely SVW and STM. After collection, the samples were allowed to air dry for ten days to achieve uniform moisture content.
After the drying process, the samples were saturated with acetone and subsequently impregnated with Aradur HY 951 resin, which was diluted in styrene (unsaturated aromatic hydrocarbon), following the method proposed by [12]. After impregnation, the samples were cut into vertical pieces on the surface, about 0.05 m thick. The pieces were glued with Araldite GY 260 onto thin sections (0.026 × 0.076 m) for use in an optical microscope, with the face of the block polished with a mechanical sander.
The section was then polished manually with diamond paste (6 to 12 μm) until the blades reached the desired thickness (30 μm). Thickness control was performed visually using a microscope, to achieve the gray color of the quartz.
With the aid of an optical microscope, a micromorphological description was performed, following the criteria proposed by [6] to present the features and general characterization of the blades for the different treatments and simulated rainfall intervals.
3. Results
There was a significant reduction in the rate of disaggregation and soil loss when comparing SWM and STM, as shown in Table 2. This indicates that the use of mulch on a bare soil surface decreases soil degradation by disaggregating particles from the impact caused by raindrops. However, there was no significant difference for either treatment (with cover and without cover) when observing the loss of soil and the rate of disaggregation between the intervals of applications of simulated rainfall (0, 24, and 48h).
Table 2 Evaluation of soil loss (SL), disaggregated rate (Dr), and water loss (WL) for STM and SWM at different times of rainfall application (TRA).
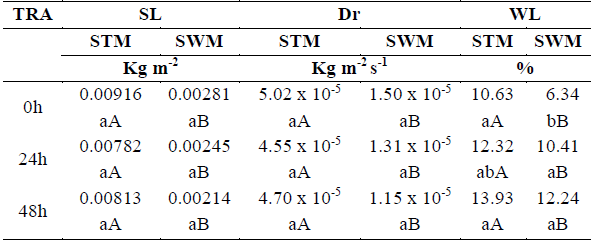
Means followed by lowercase letters in the same column and uppercase letters in the same line for each observed variable do not differ at the 5% level of significance, using the Tukey test.
According to [2], the Alto Ipanema hydrographic basin has an area of 6,209.67 km2. Across the basin, this could result in a projected soil loss of 39,431.41 tons for the 0-h interval; 33,345.93 tons for the 24-h interval and 37,195.92 tons for the 48-h interval. This represents a large amount of soil lost in the study area after rainfall events.
Likewise, the projected disaggregation rate for the entire hydrographic basin is large, with a rate of 218.59 tons per second in the first interval (0h); 201.19 tons per second in the second interval (24h) and 220.44 tons per second in the third interval (48h).
The projected amount of disaggregated soil and soil lost for the whole basin would have significant impacts in terms of soil degradation, as well as social consequences, as essential nutrients lost reduce yield from the subsistence crops cultivated in the area.
Water loss was significantly reduced with the use of mulch (Table 2), which proves the effectiveness of this technique in combating soil degradation and retaining water.
The use of mulch on the soil surface reduced the runoff by approximately 42% for the first interval of simulated rainfall, followed by a reduction of 19% for the second interval and 12% for the third interval (Table 3).
Table 3 Evaluation of runoff (ER), infiltration (I), initial runoff time (IRT), and hydraulic conductivity (ko) for STM and SWM at different times of rainfall application (TRA).
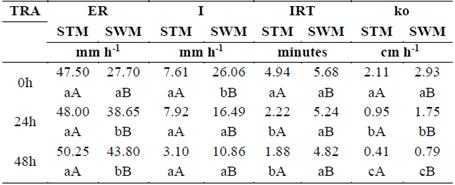
Means followed by lowercase letters in the same column and uppercase letters in the same line for each observed variable do not differ at the 5% level of significance, using the Tukey test.
Source: The Authors.
These results indicate that the mulching technique used to reduce runoff proved to be efficient in practice. Soil erosion was reduced, and infiltration was increased by mitigating runoff, [22] as infiltration and runoff are inversely proportional in an intense rainfall event [12].
Another variable presented in Table 3 is the infiltration of water into the soil. This was significantly influenced by the use of mulch to protect the soil. In addition to decreasing the speed of runoff, mulch also increased the height of the liquid blade, which favors water infiltration in the soil [1]. Thus, STM showed reductions in water infiltration of 70, 52, and 71% for the intervals of 0, 24, and 48 h, respectively, when compared to SWM.
The greater the volume of water that infiltrates the soil, the greater the water resources available for agriculture to the small rural producers in the area, which is significant, especially when considering that region is water scarce.
Hydraulic conductivity (Ko) was statistically different both for the rainfall intervals and for SWM and STM. The statistical difference between intervals is because the soil became denser with each rainfall event, making it difficult for water to seep into the soil (Table 3).
There was a significant difference between the treatments when analyzing the resistance to penetration in the topsoil (Table 4). No significant differences were observed between the rainfall intervals in both the SWM and STM plots. However, there was a significant difference between the values for SWM and STM, which indicates that the technique of covering the soil with mulch is efficient in combating surface sealing.
Table 4 Evaluation of resistance to penetration (RP) and moisture (M) in the field capacity at a depth of 0-0.02 m, for STM and SWM at different times of rainfall application (TRA).
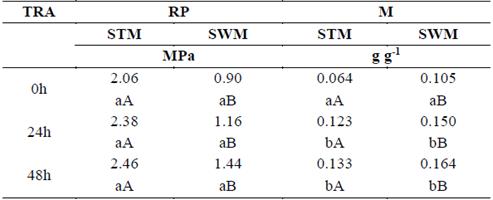
Means followed by lowercase letters in the same column and uppercase letters in the same line for each observed variable do not differ at the 5% level of significance, using the Tukey test.
Source: The Authors.
For both treatments (STM and SWM), the greatest resistance to penetration occurred after the application of rainfall at 48 h, which is evidence of the density of the soil due to the simulated rainfall.
There was a difference between the STM and the SWM plots with respect to humidity resulted from 10 kPa of tension. The discoveries presented greater resistance to penetration and lower percentage humidity. This confirms the theory that mulch reduces superficial crusting of the soil, increases infiltration and soil moisture, and consequently reduces soil erosion.
Micromorphological analysis revealed that initially the soil on the control slide had two distinct areas, referred to as zone 1 and zone 2. Zone 1, close to the surface had a thickness of approximately 0.05 m and there was an abrupt and parallel transition to the next zone. The second zone was the subsurface, containing thicker materials distributed randomly (Fig. 2A).
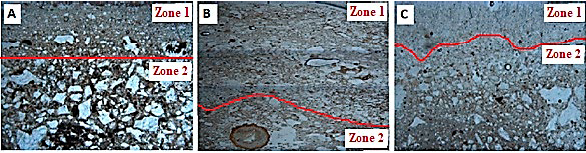
Source: The Authors.
Figure 2 Zones 1 and 2 in the soil micromorphological sample. Control (A); STM (B) and SWM (C) after the application of rainfall 0 h.
However, after the rainfall simulation event of 0 h for STM (Fig. 2B), the first changes from the control were evident. The two areas on the blade were less apparent. Zone 1, close to the surface, had a thickness of approximately 0.02 m and was composed of finer materials (fine sand + medium sand + clay). Zone 2 presented a smaller amount of clay, distributed in an undulating and abrupt manner.
For SWM, the interval 0 h was less disturbed. Zone 1 remained at 0.05 m with points reaching a maximum of 0.01 m, with the mainly medium and fine grains. The transition from this zone to the second was wavy and clear (Fig. 2C).
The blades corresponding to the 48-h interval in the rainfall simulations did not present in zone 1 because the continuity of the rain completely eroded zone 1, leaving zone 2 unchanged, as the rainfall effect was erosive to a depth of 0.02 m (Fig. 3).
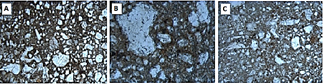
Source: The Authors
Figure 3 Zone 2 in the soil micromorphological sample. Control (A); STM (B) and SWM (C) after rainfall application at 48 h.
The blades showed clearly that the surface seals were not registered. This is a strong indication that the lack of aggregation in the soil did not allow the formation of superficial crusting. However, the loss of zone 1 in both treatments at 48 h showed that the sheer force of the rainfall was such that not even the use of mulch was could prevent this.
Failure of the surface seal results from insufficient wetting and drying cycles in the reorganization of the fine material. These cycles are required for the formation of superficial crusting [11].
The formation of the surface seal is the result of a series of factors, including temperature oscillations and repetitive cycles of soil moistening and drying, according to [4].
4. Conclusions
The use of mulch reduced soil loss by up to 73.68%, the breakdown rate by up to 75.53%, water loss by up to 40.36%, and resistance to penetration by up to 56.31%. In addition, SWM showed an increase in infiltration of up to 250.32% and a delay in runoff at the beginning of a rainfall event.
The use of mulch reduced the resistance to penetration, maintaining the critical range (2 to 3 MPa) and providing adequate conditions for root development.
In both SWM and STM, erosion occurred between the grooves owing to the slow laminar flow regime. However, the mulching technique preserved the microstructure of covered soil, while the soil showed changes in porosity and microstructure without mulch, in addition to the loss of clay.
If surface seals were not present, mulch was effective in preserving soil porosity and microstructure for the 0-h rain because of the lack of soil aggregation and the absence of wetting and drying cycles.