1. Introduction
The chemical industry, as an important link in the industrial sector, offering economic growth, job creation and product diversity, must consider the principles of green chemistry in its industrial research base to improve production processes and minimize environmental impact. Within the chemical industry is the production of surfactants. The surfactant industry consumes large quantities of petrochemical raw materials such as linear alkanes, 1-alkenes, n-alcohols, alkylbenzenes and ethylene oxide among others, and natural fats [3]. Surfactants are primarily produced from petrochemical and oleophilic raw materials, which serve as key active components in various industries.
The market for natural surfactants has been growing and is estimated to reach 17.27 billion dollars in 2022 with an annual growth rate (CAGR) of 5.1% between 2017 and 2022 [6,7]. In Colombia, several industrial plants produce anionic surfactants [1]. The non-ionic surfactants used in Colombia are imported; most of them are ethoxylated fatty alcohols from Europe, Asia and North America, which generates high import costs. Non-ionic surfactants have become increasingly important, until today they represent more than 25% of the total production of surfactants. The raw materials for their production are mainly coconut oil and ethylene oxides. According to data from a study carried out by the Medellín mayor's office in 2019, the income of the most representative companies in this sector in Colombia was approximately 8.894 billion pesos in 2018, with imports in the same period of approximately 122 million dollars [2]. To meet the internal demand for economically competitive detergents, it is essential to explore alternative production methods that consider both raw materials and technology. The viability of these methods depends on their compliance with government environmental regulations, which encourage the adoption of green chemistry principles.
The processes required to produce surfactants depend on the type of surfactant, with reactions of strong acids such as sulfuric and oils [4]. Conventional surfactant production processes require robust temperature control equipment ranging from 0 °C to 100 °C with batch or continuous flow reactors, equipped with pressure controls, agitators, or rotating disc heads. For ethoxylated alcohols in sulfonation operations, the yields are between 35% and 50% and have conversions greater than 93% with residence times for sulfonation of fatty acids of 16 to 30 minutes for batch-type reactors and flow rates for sulfonation reactors [4]. The production of alkyl glucosides requires times of 120 min at temperatures between 90 °C and 120 °C with constant stirring and with yields of less than 50% [5].
Nonionic surfactants are made up of a long alkyl chain and an uncharged, but highly polar, group. The polar group must be hydrophilic enough for the molecule to be soluble in water. The most used polar groups are polyols (sometimes natural sugars), polyoxyethylenes (polyol ethers), diamines, and ethoxylated amines. Another class of non-ionic surfactants is alkyl polyglucosides, which are produced by condensation in an acid medium of high molecular weight alcohol on a sugar-containing hydroxyl on the anomeric carbon such as aldoses.
The most applied method for obtaining glycosides is known as Fischer glycosylation, another method of obtaining is Koenigs-Knorr for the alkylation of glycosidic halides [3]. Intermediate processes with different oils and reactors have been studied for the production of surfactants. Esterification occurs as an intermediate reaction in the APG manufacturing process, Boffito D. [8] demonstrated the energy decrease and conversion increase by 45% when using an unconventional reactor assisted with ultrasound. In a similar work [9], the production of fatty alcohol from wheat straw is related to the production of an alkyl polyglucoside (APG) through the glycosylation reaction.
Anionic surfactants derived from coconut oil can be efficiently produced using ultrasound technology, which ensures homogenization and yields up to 98%. The reaction times are as short as 30 minutes, and the temperatures remain below 65 °C while operating at frequencies between 20 and 40 kHz [10]. Other research works have used different types and concentrations of carbohydrates such as mannose, xylose, and glucose dissolved in alcoholic solutions in reactions assisted with high-frequency ultrasound (550 kHz) [11]. On the other hand, the use of microwave radiation can reach up to 30 GHz, this produces a sudden increase in temperature (in the order of milliseconds), related to dielectric heating, which derives from the mobility (friction and collisions) of dipoles that try to adjust to the electric field and the molecules with the dipole moment. The energy that is created after stabilization is electrostatic and can be imagined to be like the alignment of charges with the passage of electric current or the interaction of two dipoles caused by a polar solvent [12].
Microwave-assisted processes are usual in the current industry, an example of this is the production of paper [13-15], in the same way, in the treatment of the oil palm fruit [16], reactions of lignin esterification and sulfonation [17] and other investigations summarized by Lopez-Sanchez and Priecel [18], in which it is concluded that the use of this kind of technology offers some advantages with respect to conventional methods in terms of times and yields of production. Therefore, this knowledge is a guarantee for alternative use in the production of surfactants.
The present work focuses on the evaluation of the microwave reaction conditions of nonionic surfactant of the alkylglucoside type, as an alternative for the production under the application of green chemistry: fewer solvents and short reaction times. In this work, it is proposed to evaluate the performance of the reaction in the synthesis of an alkyl glucoside (nonionic surfactant) from sacha inchi oil, which is around 49% content in the seed of the plant [19,20]. Sacha inchi is considered an alternative for the substitution of illicit crops and employment generation in Colombia [21]. To the best of our knowledge crude sacha inchi oil has been not studied to produce alkyl glucosides. In addition, the oleochemical industry in Colombia is experiencing sustained growth and has an opportunity to further improve infrastructure for the development of raw materials and intermediate inputs. Hence, alternative uses must be studied. In general, the use of surfactants has transcended from their commercial applications in food, cosmetics, cleaning, pharmaceuticals, among others, to the development of new polymeric composite and biopolymers blends in the packaging sector [22-24].
2. Materials and methods
Sacha inchi oil extracted from crops in the municipality of Jamundí, Valle del Cauca and D(+)glucose anhydrous of Panreac de 97.5% purity, p-toluenesulfonic acid monohydrate of sigma-aldrich, 98.5% purity. The Discover SP 600635 microwave equipment was used as the reactor.
The esterification reaction was carried out with the following molar ratio: 1:4.7 (glucose: sacha inchi oil) and catalyst according to conditions studied by Adisalamun, 2012 [25] in a proportion of 2% [11]. This was dissolved in 8 mL of water to generate hydrolysis according to Table 1. The microwave radiation process was operated with a constant power of 200 W and variable times. The reaction temperature was 70 ºC.
Table 1 The proportion between reactants for microwave-assisted reaction.
Sample | Amount of substance (%) | Reaction Time (min) | |||
---|---|---|---|---|---|
Water | Catalyst | Glucose | Oil | ||
APG-10 | 41.0 | 2.0 | 10.0 | 47.0 | 10 |
APG-20 | 20 | ||||
APG-30 | 30 | ||||
APG-40 | 40 |
Source: The authors.
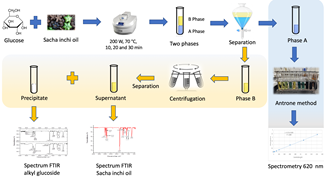
Source: The authors.
Figure 1 Scheme for obtaining alkyl glucoside using a microwave-assisted approach.
In all the samples, two phases were obtained, one aqueous and the other oily, which showed emulsification. An additional separation was carried out in the oily phase that presented high turbidity by means of a centrifuge at 1000 rpm for 5 min (see Fig. 1).
2.1. Characterizations
2.1.1 UV-Vis spectrometry
The spectrometric analysis of the dilutions was performed on a Genesys 20 Thermo Scientific equipment. Tungsten lamps at 620 nm. A standard 1% wt./wt. glucose solution in distilled water was prepared. At the same time, a 0.2% wt./wt. anthrone solution in 97% sulfuric acid was prepared, this process was carried out at a temperature of (0 °C, avoiding light exposure on the sample [26].
3. Results and discussion
3.1. Glucose yield
To corroborate the possible reaction, the phases A obtained were analyzed with respect to the amount of glucose that did not react in the different assays. The method used was colorimetric using 98% purity Anthrone as a reagent [26]. The method uses sulfuric acid at 97% purity. The reaction created in the mixture is the formation of furfural or hydroxymethylfurfural due to the hydrolytic action and the dehydration of the monosaccharides by the action of sulfuric acid, the substance obtained (see the reaction, Fig. 2) is green in color and the reading is done at 620 nm.
The calibration curve obtained in the quantification of glucose is shown in Fig. 3.
The results related to the quantification of glucose in phase A are presented in Table 2. These were obtained by clearing the equation of the line, according to the Beer-Lambert law, considering that Y is the absorbance obtained from the reading of the dilution y X of the glucose of the sample that did not react, the value obtained is subtracted with the initial amount of glucose. It is evident that the sample with 20 minutes of microwave radiation (it is the one that contains the lowest concentration of glucose), presents the highest yield of the reaction by guaranteeing that 42.8% have reacted.
To produce surfactants of the alkyl glucoside type, production times of about 120 min are required at temperatures between 90 °C and 120 °C in constant stirring, obtaining yields of less than 50% for a shorter alkyl glucoside from starch and sucrose [5]. Hence, we achieved a similar yield with lower residence times and with a lower temperature of the reaction, favoring energy efficiency.
Previous studies have reported yields ranging from 40% to 67% for the reaction between octanol and glucose, catalyzed by β-glucosidase at temperatures between 30 °C and 50 °C [27]. It is worth noting that the use of octanol as a feedstock requires additional steps in its production, which could reduce the overall yield of the process. Moreover, the previous study achieved a yield with a reaction time of three days, which was approximately 216 times less productive than the yield obtained in this work. This low productivity can make the scale-up process expensive and challenging.
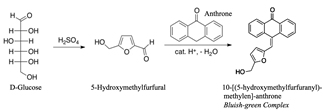
Source: The authors.
Figure 2 Schematic representation of the chemical reaction between furans and anthrone.
Table 2 Glucose conversion at different resident times.
Sample APG | Weight sample (g/mL) | Absorbance | Residual Glucose (%) | Reacted Glucose (%) |
APG-10 | 0.1272 | 1.809 | 100.0 | 0 |
APG-20 | 0.1138 | 0.958 | 57.2 | 42.8 |
APG-30 | 0.1220 | 1.240 | 69.1 | 30.9 |
APG-40 | 0.1169 | 1.155 | 67.1 | 32.9 |
Source: The authors.
The low miscibility of oil and glucose limits the yield of alkyl glucosides [27]. Hence, the production of alkyl glucosides with higher chains could potentially result in lower yields. For instance, previous studies have reported yields lower than 46% for the reaction between palm oil and glucose catalyzed by Starmerella riodocensis sp. nov [28]. This could explain why surfactants are mainly produced from coconut oil, which primarily contains C10 (7%), C12 (48%), and C14 (18%) vegetable oils [29]. In contrast, Sacha inchi is composed mainly of unsaturated fatty acid methyl esters (C18), particularly inoleic (50%) and linolenic (36%), which is expected to limit the yield of the reaction.
The use of a microwave-assisted process with low operating temperatures makes it favorable to incorporate enzymatic catalysts or other biocatalysts into the process, providing a greener alternative. Finally, the low yield of the alkyl glucoside production increasing reaction times may be related to the inhibitory effects of the product on the reaction following le Chatelier's principle. Hence, more research must be focused on recycling unreacted raw materials in the process (e.g., supernatant in Fig. 1), in cycles of repeated batches with product removal after reaction or considering in situ product separation.
3.2. FTIR
Fig. 4 shows the infrared spectrum obtained from the reagents used in Sacha inchi oil (1a) and glucose (1b). The result of the characterization of the oil (Fig. 1a) particularly shows the narrow band at 3017 cm-1 that corresponds to the stretching of the C=C-H bonds. The bands 2968 cm-1 and 2919 cm-1 which correspond to olefinic C-H bonds, the termination on one side with the methyl bond is shown in the band at 2856 cm-1 -CH3. At 1739 cm-1 the vibration of the C=O bond corresponding to a carboxylic acid can be seen. At 1648 cm-1 the vibration of the C=C-C=C bonds is observed. At 1369 cm-1 corresponds to C-H bonds [30], the presence of an -O-H group can be observed in the 1467 cm-1 band, which confirms the presence of alcohol; in bands 1160 cm-1 and 1096 cm-1 the -C-O bond is evident and finally, in 720 cm-1 the presence of C=C is confirmed [31]. In the glucose spectrum (Fig. 4b) the different characteristic bands of the hydroxyl groups are exhibited at 3422 cm-1 at 2940 cm-1 the C-OH bond, at 1341 cm-1 the C-O bond, and at 1104 cm-1 the C-O-C bond.
In the esterification tests, two phases were obtained in each vial and each phase was analyzed separately, calling them phases A and B. Phase B showed emulsification of the reaction products at 20, 30 and 40 min. While the 10 min reaction product did not show any apparent change, the initial phases remained intact. In the case of the emulsions, the separation was forced by subjecting to centrifugation.
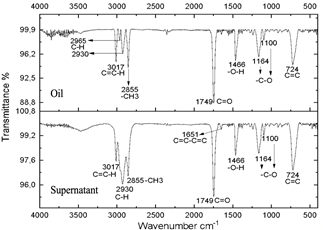
Source: The authors.
Figure 5 Spectra of oil and supernatant after centrifugation of the reaction subjected to 20 minutes of radiation.
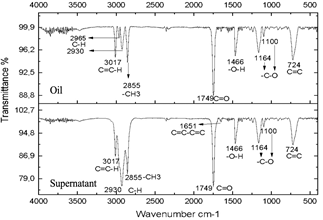
Source: The authors.
Figure 6 Spectra of oil and supernatant after centrifugation of the reaction subjected to 30 minutes of microwave radiation.
The resulting compounds were characterized. Phase A appears as the aqueous phase, which increases in volume by the condensation by-product (H2O). The compounds resulting from centrifugation were called supernatants and precipitates. The characterization of the supernatants can be observed in Figs. 5, 6, and 7, which showed that the oil did not present any significant change, this is evidenced in the bands marked.
The infrared spectra of Figs. 7 and 8 demonstrate characteristics of alkyl glucoside-type compounds, such as the bonds in the 3451 cm-1 (-OH) bands [32], which shows the presence of alcohol groups from glucose, confirming it in the band 2930 cm-1 (C-OH), at the height of 1749 cm-1 there is a narrow band which corresponds to the C=O bond and bonds of medium magnitude that are recognized between 1645 cm-1 and 1100 cm-1 the which correspond to C-O bonds [33], additionally in the 3017 cm-1 and 2855 cm-1 bands they correspond to aliphatic chain links confirming the characteristics of oils in the 724 cm-1 band.
These spectra were compared with a commercial glycoside which showed similarities in the bands which can be seen in Fig. 10, especially in the bands from 3451 cm-1 to 3423 cm-1 which are -OH type bonds, in addition to the bands located between 1749 cm-1 and 1639 cm-1 which represent C=O bonds and the other characteristic bands of aliphatic compounds in the bands between 2959 cm-1 and 2855 cm-1, the bands between 1164 cm-1 and 1083 cm-1 confirm C-O bonds [34]. In Fig. 11, shows no changes due to the carbonization of glucose due to the exposure time of the compound to radiation.
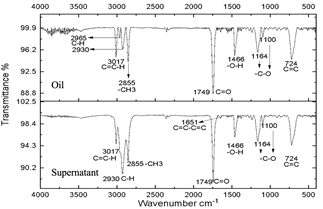
Source: The authors.
Figure 7 Spectra of oil and supernatant after centrifugation of the reaction subjected to 40 minutes of microwave radiation.
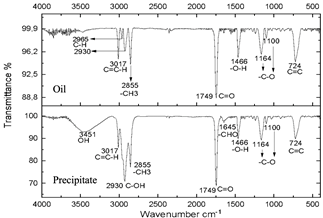
Source: The authors.
Figure 8 Spectra of oil and precipitate after centrifugation of the reaction subjected to 20 minutes of microwave radiation.
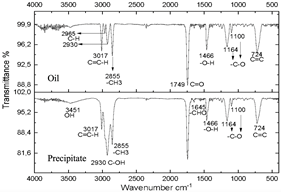
Source: The authors.
Figure 9 Spectra of oil and precipitate after centrifugation of the reaction subjected to 30 minutes of microwave radiation.
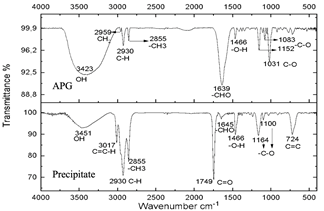
Source: The authors.
Figure 10 Spectra of commercial alkyl glucoside and precipitate from the reaction subjected to 20 minutes of microwave radiation.
4. Conclusions
It was possible to reduce the reaction time to obtain alkyl glucoside (APG) going from 120 min by conventional techniques to 20 min under microwave-assisted reaction with a power of 200 watts. The yield of the APG product under the microwave reaction was 42.8% w/w because it was carried out in a single step. The process does not require extensive purification procedures, which is favorable to possible scale-up production. The results achieved allow the planning of new experiments where the synergistic behavior with other unconventional heating modes is evaluated, eg. plasma, ultrasound, among others.